Active galaxy core

An active galaxies core also active galactic core or AGN (from English active galactic nucleus ), the central region is a galaxy which unusually large amounts of radiation not stellar origin emits.
From an astronomical point of view, the active core region is very small: it is roughly the size of the solar system and therefore appears point-like on images - similar to stars. From today's perspective, the accretion of matter through a supermassive black hole in the center of the galaxy in question is responsible for the release of energy.
The AGN standard model unifies a number of astronomical objects that have been discovered independently of one another and therefore have different names, in particular radio galaxies , Seyfert galaxies , quasars , BL-Lacertae objects , blazars and LINER .
Since active galaxy nuclei are among the most luminous objects in the universe, they are easy to see despite their great distances. Active galaxy nuclei therefore play an important role in astronomy as observation tools, for example to detect intergalactic hydrogen through absorption lines, as a distant light source for gravitational lenses or as virtually unchangeable reference points for astrometry or geodesy .
As the brightness of the core decreases, the light of the surrounding galaxy begins to dominate, so that at some point the core activity can no longer be detected. The transition to galaxies without an active core is fluid and depends on the technical observation possibilities. A good example is our home galaxy, the Milky Way . Generally not classified as an active galaxy core, occasional X-ray and infrared flares can be detected around the central black hole .
Discovery story

The first objects that today's astronomers count among the active galactic nuclei were discovered at the beginning of the 20th century. In 1909, Edward Fath (1880-1959) from the Lick Observatory published his observations of the spectral lines of distant spiral galaxies . Surprisingly, the spectrum of the spiral nebula NGC 1068 contained not only absorption lines , as would be expected if the radiation of the galaxy is largely due to the light from its stars, but also emission lines that are characteristic of gas ( planetary nebula ), which e.g. B. is ionized by high-energy radiation or shock waves.
The first systematic studies of galaxy nuclei, the spectrum of which shows emission lines, go back to Carl Seyfert , who proved in the 1940s that these lines differ systematically from the emission lines, for example from HII regions . Systematic spectroscopic investigations showed that there are two classes of such galaxies: specimens called Seyfert 1 today with narrow and broadened emission lines and Seyfert 2 galaxies in which only the narrow emission lines are visible.
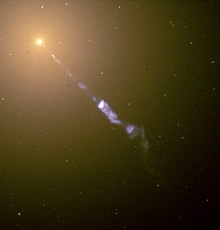
Independent of the emission line observations, the beginning of radio astronomy after the end of the Second World War led to further discoveries from the 1950s. After optical counterparts to newly discovered strong radio sources such as Cygnus A and Virgo A had been identified, it became clear that these were also extragalactic objects, namely those with enormous radiation outputs in the order of magnitude of 10 35 to 10 38 watts .
Extreme interpretations of the observation results arose in the early 1960s for the quasars , beginning with the determination of the redshift of 3C 273 by Maarten Schmidt and colleagues. The optical counterparts of the quasars appeared star-like (that is, they could not be distinguished from a point source at the given resolution). Their large redshift, however, suggested that they were extragalactic sources. With the beginning of X-ray astronomy in the early 1960s, it became apparent that some of the most prominent active galaxy nuclei also appeared as bright X-ray sources.
The enormous energy output of the active objects - first the radio galaxies, then especially the quasars - derived from brightness and distance estimates led to the assumption in the mid-1960s ( Fred Hoyle and William Alfred Fowler , Jakow Borissowitsch Seldowitsch , Edwin Salpeter ) that there was one the most effective forms of energy conversion for the course is: the release of gravitational energy during the accretion of matter onto compact objects. Black holes emerged as the most likely candidates for the required compact central objects (Seldowitsch, Salpeter, Donald Lynden-Bell ).
At the same time, references to connections between the various classes of active objects were gathered. From the early 1980s, it was possible to detect traces of the galaxies surrounding them around quasars. In the mid-1980s, Antonucci and Miller found that the Seyfert 2 galaxy NGC 1068 had similar broadened emission lines as a Seyfert 1 galaxy when observing the polarized components of the light. This indicates that the rapidly moving gas components are also present in the case of Seyfert-2 galaxies, but are hidden behind absorbing clouds; with direct observations the dimmed light of the broad lines is by far outshone; in the restriction to polarized light, the contributions of the light reflected by electrons (and polarized in this way) of the broad lines can be detected.
From these and other observations, a unified model of the active galaxy nuclei developed in the late 1980s and early 1990s, which understands all of the objects mentioned - Seyfert galaxies, quasars, blazars, radio galaxies - as different manifestations of one and the same type of system: one Supermassive black hole, surrounded by an accretion disk and other material, which partly has a shielding effect, partly stimulates its own glow.
In addition to observations of individual objects, systematic surveys played an increasingly important role in researching the active galaxy nuclei , which allowed statistical investigations on larger samples. These included the Hamburg-ESO Quasar Survey, more recently the 2df QSO Redshift Survey and the Sloan Digital Sky Survey .
Standard model
Today's standard model of active galaxy nuclei postulates a uniform structure for these objects: Inside there is a supermassive black hole with around 100 million solar masses , the Schwarzschild radius of which is around 2 astronomical units (around 16 light minutes). The source of the energy that AGNs emit is the released binding energy that arises when matter hits the accretion disk. At a distance of between 10 and 100 light days from the disk there is matter that circles the black hole quickly and is stimulated to glow by the intense thermal radiation of the accretion disk resulting from the released binding energy. The radiation of the strongly broadened emission lines comes from this so-called broad line region .
The accretion disk is also responsible for the creation of a tightly bundled jet of fast particles, which extends perpendicular to the disk plane in two opposite directions into space and can reach lengths in the range of thousands or even millions of light years. The jet starts in a comparatively tiny central region (radio core) around the accretion disk , only about a light day in size .
At a distance of a few to a few dozen light years from the center, the inner part of the system is surrounded by a frayed, thick ring of dust: the dust torus . This is aligned in the same way as the accretion disc itself. The high-energy UV light from the accretion disc is shielded by this dust torus. Above and below the torus opening, on the other hand, there are regions up to a distance of a few hundred light years in which the gas present there is ionized and thus excited to glow. This narrow line region is the source of the narrow emission lines of active galaxy nuclei.
The entire system is embedded in the central region, the so-called bulge of a galaxy, a spherical star distribution with a typical radius of around 15,000 light years.
The different appearances of active galaxies
Different appearances result from the structure of the standard model. On the one hand, active galaxy nuclei can differ in their activity level, largely determined by the accretion rate. The activity of quasars is very high, so that the active core outshines the rest of the galaxy by far. In Seyfert galaxies , the core is much less luminous, so that it appears as a bright region of the otherwise easily visible surrounding galaxy. According to the standard model, every quasar should have a surrounding galaxy (host galaxy) ; The prediction confirms that more and more of these galaxies can be detected with better and better observation methods.
The dust torus shields the ionizing UV radiation from the accretion disk. The broad line region (BLR) is therefore only visible from above or below . In this case the observer discovers strongly broadened lines in the spectrum, whereby the Seyfert 1 galaxies and the quasars of type 1 come about in the standard model.
If, on the other hand, the radiation from the BLR is shielded by the dust torus, only the narrow emission lines can be seen. This leads to Seyfert-2 galaxies or quasars of type 2. In this situation, only the part of the BLR radiation can be seen that escapes upwards or downwards from the torus and is then scattered in the direction of the observer. Taken by itself, this radiation is too weak to be detectable in comparison with the radiation from the narrow lines. However, due to the scattering, this BLR component is strongly polarized. Therefore, if one restricts oneself to measurements on polarized light, the BLR component is easily detectable.
Another, rare orientation effect occurs when one of the jets is aimed directly or almost directly at the observer. This is how the standard model explains blazars or, with low luminosity, BL-Lacertae objects .
The model also explains the roles of the different wavelength ranges of light: The X-ray radiation from the accretion disk is hardly attenuated by the dust torus and is therefore visible in all active galaxy nuclei. UV and visible light from the accretion disk and the associated emission lines of the BLR are only visible if the torus is properly oriented. In the infrared, on the other hand, we can also detect the radiation from the dust torus itself.
Depending on the inflow rate of the incident matter, the accretion disk heats up more or less. Cooler accretion disks give off less thermal radiation and offer favorable conditions for magnetic field lines that are carried along with the plasma and represent one of the possible mechanisms for the formation of a jet and the associated synchrotron radiation , especially in the radio range. Hotter panes radiate significantly more heat and offer less favorable conditions for jet formation. The standard model can explain the existence of radio loud (jet or synchrotron radiation dominate) and radio-iron AGN.
Central black hole
In the center of the active galaxy core is a supermassive black hole , which typically has around 100 million solar masses , in extreme cases 10 to 20 billion solar masses. The need for such a particularly compact central object to explain the luminosity of the AGNs was inferred from considerations on the extent of the energy release in AGNs. Accretion, i.e. the incidence of matter under the influence of gravity , can have the highest efficiency of all types of energy release (ratio of released energy to the mass of the incident matter).
Theoretical description
A naturally formed black hole will generally have a non-zero angular momentum, i.e. it will rotate. Rotating black holes are described by the so-called Kerr solution . It has a so-called ergosphere around the horizon , in which all matter located there is forced to rotate around the black hole. This fact plays an important role in some models for the formation of jets .
The innermost stable orbit of a particle, if gravitational wave effects are disregarded, is in a Kerr hole, especially for particle orbits with the same orbital sense as the black hole itself, much further inside than for non-rotating ( Schwarzschild ) holes. The proportion of binding energy that can be released before matter disappears in the black hole is correspondingly greater, which means that the efficiency that can be achieved during accretion increases accordingly. With a Kerr hole rotating at maximum speed, the efficiency would be 42% compared to just under 6% with a Schwarzschild hole.
Size and observation options
The radius from the center to the event horizon (Schwarzschild radius) of a black hole depends on its mass. For a supermassive black hole with 100 million solar masses it is 2 astronomical units . At 10 billion solar masses you get 200 AU and for the supermassive black hole in the center of our home galaxy (Milky Way) , with around 4 million solar masses, it is just under 0.1 AU. In the future it will be technically possible to observe such small structures in the center of our home galaxy. Outside of our galaxy, such observations will be technically impossible in the foreseeable future.
Mass determination
The mass of the central black hole can be determined in several ways. In our home galaxy, this goes through Kepler's third law , since the orbits of individual stars can be observed directly.
Another possibility of determination arises when spectra can be determined for different sections of the core area of a galaxy and it can thus be estimated within which limits the speed of the stars in the regions concerned lies. From the width of this distribution ( velocity dispersion ) one can deduce the gravitational potential via the virial theorem . The distance of the observed areas from the center follows from these observations and the distance to the galaxy, so that conclusions can be drawn about the enclosed mass from the potential value .
A third method is reverberation mapping , in German roughly "reverberation recording". This is used for type 1 galaxies, in which the radius of the broad line region cannot be observed spatially resolved. However, the radius is needed to determine the gravitational potential in order to infer the mass with the help of the virial theorem and the potential. In reverberation mapping , echo effects are analyzed in order to infer the radius. This takes advantage of the fact that the window radiation fluctuates on time scales down to less than an hour. This information reaches us directly through the AGN spectrum. The same fluctuations occur with a time delay in the broad spectral lines because the radiation first has to be reflected through the broad line region to the observer. Since the radiation propagates at the speed of light , the time delay is a measure of the distance from the accretion disk to the broad line region. A systematic evaluation of the time delays enables the radius to be determined.
Accretion disk
Material such as gas or dust that falls on a mass can only reach the central object directly for very special directions of movement. In general, the angular momentum of matter relative to the central object will cause the material to fall slightly past the object. If it collides with matter arriving from other directions, an accretion disk can arise. An accretion disk is a rotating gas and dust disk in which the angular momentum is transported to the outside through internal friction, i.e. due to the viscosity of the material, so that matter can fall onto the central object from the inner edge of the disk. According to current knowledge, the source of viscosity is turbulence due to magnetohydrodynamic instabilities.
The disk heats up considerably as a result of the collisions of the new material falling on the accretion disk with the existing material. Which temperatures are reached depends on the incidence rate (accretion rate). At lower rates the temperatures are around ten thousand and at higher rates up to a few hundred thousand Kelvin .
The power of the thermal radiation of an accretion disk results from the temperature profile . Following the Stefan-Boltzmann law , higher temperatures also generate larger amounts of radiation. The spectrum of the pane is not that of a Planck radiator with a single characteristic effective temperature , but results from the superposition of the Planck spectra of the pane regions with different temperatures from the outside to the inside.
The upper limit of the radiation power is given by the Eddington limit , above which the radiation pressure prevents further penetration of matter.
Hot and cooler slices
In connection with assumptions about the pane material and with the Stefan-Boltzmann law , the Eddington limit also gives an upper limit for the temperature of the pane. This upper limit is lower, the greater the mass of the central black hole. The following applies to a disk whose expansion is constant in relation to the Schwarzschild radius . More precise calculations with concrete disk models lead to the same connection. The maximum temperatures of the AGN disks are therefore well below the temperatures of the disks of stellar black holes. In particular, AGN panes do not produce any significant amounts of X-ray radiation as thermal radiation (for the origin of this radiation component, see section Corona and X-ray radiation ).
Hotter panes with effective temperatures of around one hundred thousand Kelvin produce considerable amounts of UV radiation. In the AGN spectrum, this leads to the (big) blue bump (analogously: to the “(large) blue hill”), a maximum in the extreme UV range , which ranges from higher energies to around 0.6 keV , to lower energies down to Wavelength range drops by 1 μm. The UV radiation causes the ionization of the matter in the broad line region and is thus indirectly responsible for the broad emission lines in type 1 AGNs. Based on the broad lines, conclusions can be drawn about the total radiation output of the pane.
On the other hand, cooler panes produce significantly less UV radiation. Therefore, the lines from the broad line region are significantly weaker, if they can still be detected at all. Discs with a cooler temperature favor the generation of jets and the associated synchrotron radiation (see section Jet ).
Hotter panes, where the thermal radiation and cooler panes, where the synchrotron radiation dominates, are the explanation for radio loud and radio quiet AGNs in the standard model.
Corona and x-rays
In contrast to the situation with stellar black holes, AGN accretion disks are not hot enough to directly produce large amounts of X-rays as thermal radiation. The fact that AGN still radiate intensely in this area is attributed to a corona: a region of extremely hot plasma directly above and below the disk. X-ray radiation occurs when UV radiation from the pane receives additional energy from the plasma electrons ( inverse Compton scattering ), is reflected back to the pane in this form and from there is emitted into space as softer X-ray radiation. In the spectrum of the AGN, this fact results in characteristic broad iron lines due to fluorescence as well as a characteristic maximum around 30 keV , as one would expect for the reflection of the radiation on cold matter (backscattering on the pane).
Characteristic for the X-ray radiation from AGN are intensity fluctuations on astronomically very short time scales of hours or less. It follows that the regions in which the X-ray radiation is produced must be very small. Correspondingly, today's models are based on lump structures in the corona, which arise and fade on the corresponding time scales and, during the period of their existence, stimulate localized areas of the disk below to glow.
Disc spectrum and relativistic effects
The spectrum of a rapidly rotating accretion disk around a central black hole, which the observer does not view from above but rather at an angle, is largely determined by relativistic effects . In the classical case, the spectrum of a disk at rest would be symmetrical around the center of the spectrum with two lateral maxima corresponding to the Doppler red and blue shifted areas of the disk which, from the observer's point of view, have the greatest radial velocity . In the relativistic case, the effects of time dilation and gravitational redshift are added, which shift the spectrum as a whole towards the red, as well as relativistic beaming , which breaks the symmetry and makes the radiation of the disk sections that are maximally moved towards the observer appear significantly brighter than that of the observer moved away. A corresponding shape of the spectral lines was first detected in 1995 in the active galaxy MCG-6-30-15.
For a bright partial source that runs around the black hole, the same relativistic effects lead to a characteristic change in the spectrum over time. By measuring the spectrum, conclusions can be drawn about the mass and angular momentum of the black hole. In 2004, such effects could be detected for the first time in the X-ray spectra of the Seyfert galaxy NGC 3516 , which were recorded with the XMM-Newton space telescope . From this, the mass of the galaxy's central black hole could be estimated between 10 and 50 million solar masses.
Broad line region (BLR)
At a distance of a few thousand to a few tens of thousands AU (between 10 and 100 light days ) around the accretion disk is the Broad Line Region (BLR), in which the strongly broadened spectral lines of the AGN arise. The line width indicates high speeds of approx. 3000 km / s, while changes in the line width over time on time scales of hours or days demonstrate the small extent of the region. The fact that there are no forbidden lines under the wide lines indicates that the material is sufficiently dense.
jet
Jets are strongly bundled outflows of high speed, which are mostly bipolar , i.e. in two opposite directions from the central object. Jet sources always have accretion disks and strong magnetic fields .
It is now assumed that jets are magneto-hydrodynamically accelerated and collimated and that the outflowing matter originates from the accretion disk (model by Blandford & Payne, 1982). But purely electrodynamic processes can also play an important role. B. use the rotational energy of the black hole in the center of the AGN. The details and weighting of the different processes have not yet been finally clarified and are the subject of current research.
The direct contribution of the jets to the radiation of the AGN is the synchrotron radiation generated by the electrons in the jet , reinforced by effects such as the inverse Compton scattering of the radiation on the electrons of the jet or external matter. Shock fronts can develop in the jet itself , at which some electrons are accelerated to significantly higher energies, with Lorentz factors between 100 and 1000 ("shock nodes" in the jet).
Jets are physically significant for the loss of mass and angular momentum of the central source and the energy input into the surrounding medium (cf. the section on interaction with the surrounding galaxies ). Where the jet particles hit the surrounding intergalactic medium, extensive, radio-bright excitation areas arise (cf. the section Emission Areas (Praise) ).
Relativistic beaming
Many AGN jets move at a relativistic speed, with Lorentz factors up to about 10-15, corresponding to 99.5 to 99.8 percent of the speed of light .
The light emitted by the particles flowing in the jet (especially in the form of synchrotron radiation) is amplified many times over at such speeds due to the relativistic beaming effect for observers in or near the direction of movement: a combination of aberration and (relativistic) Doppler Blue shift can increase the radiation flux measured by such an observer by a factor of 100 or more. This is an important factor in explaining the great brightness of blazars, for example, where, according to the AGN standard model, we look directly into one of the jets. The same relativistic effects lead to a weakening of the radiation for a jet moving away from the observer. This is an important reason why the two jets of a radio galaxy usually appear differently bright on astronomical recordings ( Laing-Garrington effect ).
Apparent faster than light speeds
For objects that move relativistically towards the observer, light transit times can lead to a distant observer mistakenly concluding that the objects are moving faster than light. In this way, direct calculations of the type “change in angular distance (in radians ) × distance = tangential velocity ” for nodes in a jet that move away from the central source lead to velocities that are many times the speed of light.
From the correct calculation, which takes the light transit times into account, it follows that these apparent superluminal speeds are a clear indication that the jets in question are moving relativistically, i.e. with Lorentz factors significantly greater than 1. Such calculations are the first evidence of relativistic velocities in astrophysics.
Relativistic effects follow from these high speeds, in particular relativistic beaming , which explain the high luminosity of the jets with suitable orientations (jet moves towards the observer) and thus explain the properties of the blazars in the standard model.
Connection with cosmic rays
It has long been suspected that AGN's jets are the source of at least some of the high-energy cosmic rays that reach Earth from space. The measurements of gamma observatories such as the Pierre Auger Observatory , which have found correlations between the direction of incidence of cosmic rays and the positions of AGN in the sky, give evidence of a connection between cosmic rays and AGN .
Dust torus
The dust torus surrounds the inner area at a distance of a few hundredths to a few dozen light years (0.01 to 10 parsecs ). Its most important role in the standard model results from its shielding properties, which are particularly decisive for the differentiation between AGN of type 1 (light from the broad line region visible) and type 2 (light from the broad line region not visible).
More recent detailed investigations, which have made use of interferometric methods in the near and mid-infrared range in particular, question the image of a coherent dust torus. According to these observations, it is instead a more or less individual, irregular arrangement of dust clouds in the corresponding region.
Supply of matter

An AGN will only remain active as long as a sufficiently strong flow of matter onto the accretion disk is ensured. The main obstacle is the angular momentum of the matter falling towards the disk. If the angular momentum is too great, matter will never be able to reach the disk. In today's models, the last parsec (approx. 3 light years ) around the disk is particularly problematic in this regard .
According to some models, bar structures in the galaxy surrounding the AGN play an important role in transporting sufficient matter to the central regions. Other models see galaxy collisions and the resulting instabilities that lead to deeper potentials as the decisive mechanism to secure the supply of matter.
Emission Areas (Praise)

The emission areas, also called praise ( lobes or radio lobes ) based on the English , are the largest structures associated with the AGN , with dimensions between ten thousand and millions of light years (3 to 1000 kpc ). They arise when the fast particles - especially the electrons - of the jet hit the surrounding intergalactic medium and carry it with them in whole or in part. The entrained gas in the surrounding medium reaches supersonic speeds , so that a shock front is formed at the front end : a particularly strong, radiant “hot spot” .
The radio emission of the praises, which is synchrotron radiation due to the movement of charged particles in magnetic fields, exceeds the luminosity in the visible range by factors of 100 million to 10 billion.
classification
The conventional division of the active galaxy nuclei into different classes is based on observation features, in particular the properties of the spectrum, the luminosity of the AGN itself, the luminosity of the galaxy surrounding it and the presence of strong radio emissions ("radio loud", "radioleise").
This classification is opposed to the classification according to physical properties, as they result from the AGN standard model. The most important parameters are the mass of the central black hole and the accretion rate - these and the other physical parameters cannot be observed directly, but must be deduced from the observations.
The connection between the physical parameters and the radio volume of an AGN is less clear. The activity appears to increase as the mass of the black hole increases; however, there is greater variation here .
Seyfert galaxies
Historically, Seyfert galaxies are objects that clearly have the structure of galaxies and also have a very bright core. In the standard model, the brightness of the core corresponds to a high accretion rate. The fact that the surrounding galaxy is still clearly visible is what distinguishes Seyfert galaxies from the much brighter quasars. As the observation technique advances, more and more surrounding galaxies can be imaged with quasars, which weakens the traditional distinction.
Based on the Seyfert galaxies, the subdivision into type 1 (broad emission lines visible) and type 2 (only narrow emission lines) was made for the first time, which in the standard model is based on whether the inner regions near the accretion disk are visible (type 1) or through the Dust torus are covered (type 2). This classification was later extended to all AGNs (see section Appearance, Classification in Type 1 and Type 2 ). Based on the intensity relationships of the Hα and Hβ lines, Donald Osterbrock introduced an even finer subdivision with Seyfert galaxies of the types 1.5, 1.8 and 1.9 in 1977 and 1981, which, however, did not prevail among all astronomers in the field.
Quasars
Were historically quasars "quasi-stellar radio sources" (English. Quasi-stellar radio source ). The first examples ( 3C 48 and 3C 273 ) were discovered by Allan Sandage and co-workers in the early 1960s . Only with the determination of the redshift by Maarten Schmidt for 3C 273 it became clear that the objects are extragalactic and therefore very small and extremely bright from an astronomical point of view.
Phenomenologically, quasars were those very bright, active galaxy nuclei, in which no surrounding galaxy could be detected, only a bright nucleus. Due to the small size of the core region, only a star-like object, which cannot be distinguished from a point source with the telescope in question, was recorded.
In a modern classification, quasars differ from Seyfert galaxies by the particularly high luminosity of the accretion disk and the surrounding regions.
The AGN standard model does not recognize “naked quasars” without a surrounding galaxy. Observations indicate that for quasars with no detectable surrounding galaxies, these galaxies are too faint, so that it is a sample distortion due to the limitations of the observation instruments.
Quasars are divided into radio-loud or radio-quiet according to their luminosity. Some authors differentiate these two cases in the naming and only name the radio-loud objects quasars, while the radio-iron objects QSOs (for quasi-stellar object ).
Radio galaxies
Radio galaxies are characterized by their high radio luminosity, which can be up to 10 39 or 10 40 watts . That is more than a billion times the power that normal galaxies radiate in the radio range.
As a typical structure, many radio galaxies have two opposing excitation or emission regions, so-called lobes. These are vast areas of very hot gas that are created when the two jets emitted by the galaxy's core in opposite directions hit the intergalactic medium surrounding the galaxy .
From a modern point of view, radio galaxies are radio-loud quasars of type 2. They are each very massive elliptical galaxies, mostly the central galaxies of extensive galaxy clusters. Compared to Seyfert galaxies, radio galaxies have a much lower accretion rate - their emissions are accordingly dominated by the luminous phenomena associated with the jet.
The Fanaroff-Riley classification differentiates between two types of radio galaxies: Type I (FR-I), in which the brightness decreases from the center outwards, and the generally much more luminous type II (FR-II) radio galaxies, which are the sources are further out with the highest surface brightness.
Blazars: BL-Lac objects and optically highly variable quasars (OVV)

BL-Lac-Objects or BL-Lacertae-Objects are named after the constellation Lacerta (lizard), in which the first discovery by Cuno Hoffmeister in 1929 is. The BL is the name for the classification of variable stars and comes from the fact that Hoffmeister considered the object to be a variable star with irregular variations in brightness. With the advent of radio astronomy it became clear that these are radio-loud, very distant objects. The first distance determinations were not made until the 1970s.
From the point of view of the standard model, BL-Lac objects correspond to an orientation of the AGN in which the observer looks directly or almost directly into the jet. The same explanation is given by the standard model for the Flat Spectrum Radio Quasars (FSRQ), synonymous with optically violent variables (optically highly variable quasars, abbreviated OVV). Both object classes are therefore combined and referred to as blazars (from the English to blaze, to shine very brightly).
BL Lac and OVV differ in the properties of their lines, which in BL Lac are weaker and accompanied by absorption lines, the lines being strongly polarized, while OVV show strong and very broad emission lines. They have in common strong variations in brightness on short time scales (hours to days), which can be explained by the fact that the jet emerges from a compact region above the accretion disk, which due to its small size can change its properties on short time scales.
LINERs
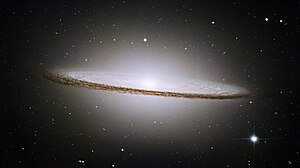
At the other end of the performance spectrum, near the borderline to inactive galaxies, are LINERs. The name comes from the English low-ionization nuclear emission-line region, in German about galaxy core regions with emission lines with a low degree of ionization. As the name implies, these objects have emission lines from weakly ionized ions or neutral atoms, such as O , O + , N + or S + , while emission lines from more strongly ionized atoms are comparatively weak.
LINER occur in around a third of the galaxies in our cosmic neighborhood. As a link between active and inactive galaxies, they suggested for the first time that non-active galaxies could also have supermassive holes in the center. This was only later confirmed by the discovery of the black hole in the center of the Milky Way .
Whether LINERs are actually AGNs that are driven by a supermassive central black hole according to the standard model is the subject of discussions. Instead, some astronomers suspect particularly powerful star-forming regions behind the activity .
Interaction with surrounding galaxies
The physical processes in an active galaxy core and the surrounding galaxy play out in size scales from the extent of the central region with a few light days to the thousands of light years of the bulge . Statistical relationships between the physical properties of the active nuclei and their galaxies, which indicate mutual influencing or coupled evolution, have been found since the 1990s.
Correlations of masses, brightnesses and velocity dispersion
In 1995 John Kormendy and Douglas Richstone noticed a correlation between the mass of the central black hole of AGN and the (blue) total brightness of the surrounding bulge (for elliptical galaxies : the entire galaxy). A further study by John Magorrian and colleagues in 1998 showed a correlation between the masses of the objects located in the bulge and the central black hole.
Later studies found correlations between the velocity dispersion of the stars in the bulge and the mass of the black hole. This relation has the smallest spread of those mentioned so far ; however, depending on the study, there were different parameters for the relationship (slope of the best-fit straight line ).
Active galaxy nuclei as observation tools
Quasars, radio galaxies and other AGN are not only of interest as objects of interest. They can also serve as observation tools that can be used to study other astronomical objects, such as the intergalactic medium or the galaxy surrounding the active galaxy core. The quasars in particular are used on earth as immobile, unchangeable reference points for geodetic measurements.
Absorption by matter between the quasar and the observer
Absorption of light from a quasar at characteristic frequencies provides information about the matter that is between the quasar and an earthly observer. The cosmological redshift plays an important role in the relevant distances . Depending on the distance of the absorbing material, the absorption lines appear in the quasar light at different wavelengths. The quasar spectrum thus contains information about the spatial distribution of matter along the line of sight.
Lyman Alpha Forest
The most important expression of this phenomenon is the Lyman-alpha forest (Engl. Lyman alpha forest ). The dense series of absorption lines of the Lyman-Alpha transition of neutral hydrogen atoms, which are caused by hydrogen gas clouds at different distances (and thus different redshifts). A Lyman-Alpha forest was first detected in 1971 for the Quasar 4C 05.34.
Measurement of the "cosmic web"
Quasar absorption lines not only provide general information about the presence of intergalactic hydrogen gas, but can also be used to study how galaxies interact with the gas surrounding them. In conventional models, gas flowing from the “ cosmic web ” of intergalactic gas into a galaxy provides important supplies of raw material, which allows the galaxy to form new stars in the long term. Cold hydrogen gas detected by quasar absorption lines in the immediate vicinity of star-forming galaxies supports this picture.
Where only punctual statements about the cosmic network are possible based on direct absorption lines, fluorescence phenomena, in which a distant quasar illuminates a section of the network like a flashlight and thus stimulates its own glow, can make flat sections of the network visible. Corresponding recordings were first made in 2014 with one of the Keck telescopes .
Gunn Peterson Trough
The (little) hydrogen gas that is found everywhere in intergalactic space should not generate a forest of lines in view of its continuous distribution, but rather quasar light at all wavelengths between the unshifted Lyman-alpha wavelength and the Lyman-alpha, which is shifted with the redshift of the quasar - Weaken line. This so-called Gunn-Peterson trough was postulated in 1965 by Gunn and Bruce A. Peterson and used to estimate an upper limit for the cosmic density of neutral hydrogen.
The Gunn-Peterson trough becomes interesting with regard to the ionization history of the early universe. In the development models accepted today, the first hydrogen atoms are formed around 380,000 years of cosmic time (at the same time the cosmic background radiation is released). During the reionization epoch between 150 million and 1 billion years later, this atomic gas is re-ionized by the high-energy radiation from the first stars. A Gunn-Peterson trough should therefore be detectable for those areas along the line of sight to a distant quasar that the light passed through in the time before reionization. Its location in turn allows conclusions to be drawn about the timing of the reionization phase, and thus about the age of the oldest stars. Such a trough was observed directly for the first time in 2001 in the spectrum of a quasar with redshift z = 6.28.
Chemical evolution: deuterium
Absorption lines, which can be assigned their redshifts according to the distant cosmic past, are also important for the reconstruction of chemical evolution, i.e. the temporal development of the element and isotope abundances. Quasar absorption lines play a role in particular for the reconstruction of the deuterium abundance in the early universe and make it possible to test the predictions of the Big Bang models for the formation of this light element ( primordial nucleosynthesis ).
The primordial deuterium abundance is the most sensitive indicator of the number of baryons in the early universe, defined as the ratio of the numbers of baryons .
Variability of natural constants
Quasars allow conclusions to be drawn as to whether and how important fundamental natural constants may have changed in the course of cosmic evolution. The patterns of the spectral lines have a characteristic structure ( multiplets ), which results from the quantum mechanical properties of the generating atoms and molecules . The wavelength differences of the lines of one and the same group depend in a precisely describable way on the fine structure constant , which indicates the strength of the electromagnetic interaction . Quasar observations of absorption lines make it possible to determine the fine structure constant from such wavelength differences at different distances and thus at different times in cosmic history. For example, the light from spectral lines with a redshift of z = 0.5 was generated 5 billion years ago and thus carries information about the strength of the electromagnetic interaction precisely that time. Measurements on absorption lines of distant quasars have given indications of slight variations in the fine structure constant, which, however, are currently still being discussed controversially.
Astrometry and Geodesy
Due to their high luminosity, quasars are among the objects that are still visible at the greatest distances. The high distances mean that the apparent position of a quasar in the night sky does not change for an observer on earth, even if the quasar moves at high speed relative to its cosmic environment. Quasars are therefore suitable for determining the International Celestial Reference System (ICRS) with great accuracy.
The astrometry satellite Gaia , which measures the distance of around one billion stars using the parallax method with unprecedented accuracy, will carry out a survey of around 500,000 quasars as part of its mission and use some of them as a tool to calibrate its parallax measurements.
Relative to the frame of reference given by the quasars, very long baseline interferometry (VLBI) can also be used to determine terrestrial movements and locations with great accuracy. In this way, precise measurements of the parameters of the earth's rotation as well as the continental drift are possible .
AGN as standard candles
Cosmologists working with Darach Watson from the University of Copenhagen have developed a method for determining cosmic distances with the help of AGN . The method is based on the relationship between the absolute brightness of galaxy nuclei and their brightness as it appears from Earth.
If matter falls into the central black hole of the galaxy, it heats up considerably and emits high-energy radiation as a result. This ionizes neighboring gas clouds and thereby stimulates them to glow. A lighter central region around the black hole leads to deeper ionization of the surrounding gas clouds and thus also to a more extensive area in which gas clouds glow. Changes in the brightness of the central region are also expressed with a delay in the brightness of neighboring gas clouds. Using this, the size of the glowing gas clouds for 38 AGN and then the absolute brightness of the AGN itself were determined. In this way, distances up to a redshift of 4 can be determined, corresponding to approx. 55% of the radius of the visible universe.
Role as a marker
According to the standard model, quasars are embedded as active galaxy nuclei in surrounding galaxies. Quasars can thus be used as markers, because where a distant quasar is detected, there should also be a whole galaxy, which can now be searched for in a targeted manner.
Gravitational Lenses and Relativistic Optics

Because of their great distance and great brightness, quasars are suitable as light sources for gravitational lenses , i.e. for situations in which the light of a distant object is deflected by the mass of an object closer to the observer. This deflection typically leads to amplification effects, but with a suitable arrangement also to multiple images, which are often distorted in an arc-like manner. In fact, in 1979 the “twin quasar” Q0957 + 561 was the first ever known example of a gravitational lens.
In addition to large-scale gravitational lensing effects in which separate images are visible, quasars also have micro-gravitational lensing effects in which the individual images cannot be distinguished from one another, but the lens effect leads to a significant light intensification. Conclusions can be drawn about the light-emitting regions of the quasar ( continuum source and broad line region) as well as about the properties of the lens objects from the brightness fluctuations that result from such microlens effects.
The statistics of quasar lenses have interesting cosmological consequences: From the proportion of quasars that are part of a gravitational lens system, it is possible to estimate the total amount of matter in the universe that is present in the form of compact objects (i.e. potential gravitational lens masses).
Quasars are also suitable for checking the influence of the sun's mass on the light of distant objects with the help of Very Long Baseline Interferometry (VLBI) as another example of relativistic light deflection . The cumulative evaluation of the corresponding data from over 2 million VLBI observations on 541 quasars and radio galaxies is one of the most precise measurements of light deflection by the sun and thus one of the strictest tests of the predictions of the general theory of relativity about this light deflection.
literature
- Volker Beckmann and Chris R. Shrader: Active Galactic Nuclei . Wiley-VCh, 2012, ISBN 978-3-527-41078-1 .
- Bradley W. Carroll and Dale A. Ostlie: An Introduction to Modern Galactic Astrophysics and Cosmology . Addison-Wesley, 2007, ISBN 0-8053-0347-2 .
- Bradley M. Peterson: An Introduction to Active Galactic Nuclei . Cambridge University Press, 1997, ISBN 0-521-47911-8 .
- Kenneth Lang : Essential Astrophysics . Springer, 2013, ISBN 978-3-642-35962-0 .
- Martin J. Rees : Black Hole Models for Active Galactic Nuclei . In: Annu. Rev. Astron. Astrophys. tape 22 , 1984, pp. 471–506 ( online version on NED ).
- Ian Robson: Active Galactic Nuclei . John Wiley & Sons, Chichester 1996, ISBN 978-0-471-96050-8 .
- Gregory A. Shields: A brief history of AGN . In: Publications of the Astronomical Society of the Pacific . tape 760 , no. 111 , p. 661–678 , arxiv : astro-ph / 9903401v1 .
- Donald E. Osterbrock : Astrophysics of Gaseous Nebulae and Active Galactic Nuclei . Palgrave Macmillan, 2005.
- Julian H. Krolik: Active Galactic Nuclei: From the Central Black Hole to the Galactic Environment . Princeton University Press, 1998.
- Daniel W. Weedman: Quasar Astronomy . Cambridge University Press, 1988, ISBN 0-521-35674-1 .
See also
- Compact Symmetric Object , possible forerunner of active galaxy nuclei
Web links
Individual evidence
- ↑ a b According to Section 2.1 in Peterson 1997.
- ↑ For the first time FK Baganoff u. a .: Rapid X-ray flaring from the direction of the supermassive black hole at the Galactic Center . In: Nature . tape 413 , 2001, p. 45–48 , bibcode : 2001Natur.413 ... 45B .
- ^ EA Fath: The spectra of some spiral nebulae and globular star clusters . In: Lick Obs. Bull. Band 5 , 1909, pp. 71 , bibcode : 1909LicOB ... 5 ... 71F .
- ↑ See Sections 11.1 and 13.2 in Lang 2013.
- ↑ C. Seyfert: Nuclear Emission in Spiral Nebulae . bibcode : 1943ApJ .... 97 ... 28S . See Section 2 in Shields 1999.
- ↑ a b E. Ya. Khachikyan, DW Weedman: A spectroscopic study of luminous galactic nuclei . In: Astrophysics . tape 7 , no. 3 , p. 231–240 , bibcode : 1971Ap ...... 7..231K .
- ↑ On radio galaxies, section 2, on quasars, section 3 in Shields 1999.
- ↑ Maarten Schmidt: 3C 273: A Star-Like Object with Large Red-Shift . In: Nature . tape 197 , 1963, pp. 1040 , bibcode : 1963Natur.197.1040S .
- ↑ See Section 4 in Shields 1999.
- ↑ General: Section 4.2. in Shields (1999) and Werner Israel (1987): Dark stars: the evolution of an idea. In: Stephen W. Hawking, Werner Israel: 300 Years of Gravitation. Cambridge University Press, pp. 199-276, ISBN 0-521-37976-8 .
- ^ S. Wyckoff, T. Gehren, PA Wehinger: Resolution of quasar images . In: Astrophysical Journal . tape 247 , 1981, pp. 750-761 , bibcode : 1981ApJ ... 247..750W .
- ↑ JB Hutchings, D. Crampton, B. Campbell: Optical imaging of 78 quasars and host galaxies . In: Astrophysical Journal . tape 280 , 1984, bibcode : 1984ApJ ... 280 ... 41H .
- ^ RRJ Antonucci. JS Miller: Spectropolarimetry and the nature of NGC 1068 . In: Astrophysical Journal . tape 297 , 1985, pp. 621-632 , bibcode : 1985ApJ ... 297..621A .
- ^ A b R. Antonucci: Unified Models for Active Galactic Nuclei and Quasars . In: Annual Reviews in Astronomy and Astrophysics . tape 31 , no. 1 , 1993, p. 473-521 , doi : 10.1146 / annurev.aa.31.090193.002353 , bibcode : 1993ARA & A..31..473A .
- ^ Meg Urry, Paolo Padovani: Unified schemes for radioloud AGN . In: Publications of the Astronomical Society of the Pacific . tape 107 , 1995, pp. 803-845 , doi : 10.1086 / 133630 , arxiv : astro-ph / 9506063 , bibcode : 1995PASP..107..803U .
- ^ The 2dF QSO Redshift Survey (2QZ).
- ^ R. Antonucci: Unified Models for Active Galactic Nuclei and Quasars . In: Annual Review of Astronomy and Astrophysics . tape 31 , 1993, pp. 473 .
- ^ Matt Visser: The Kerr spacetime - a brief introduction. (First publication: arxiv : 0706.0622 ), page 35 , Fig. 3.
- ↑ Section 13.14 in MP Hobson, GP Efstathiou and AN Lasenby: General Relativity: An Introduction for Physicists . Cambridge University Press, 2006, ISBN 978-0-521-82951-9 .
- ↑ Vincent L. Fish, Sheperd S. Doeleman: Observing a black hole event horizon: (sub) millimeter VLBI of Sgr A * . In: Relativity in Fundamental Astronomy: Dynamics, Reference Frames, and Data Analysis, Proceedings of the International Astronomical Union, IAU Symposium . tape 261 , p. 271-276 .
- ↑ Holland C. Ford, Richard J. Harms, Zlatan I. Tsvetanov, George F. Hartig, Linda L. Dressel, Gerard A. Kriss, Ralph C. Bohlin, Arthur F. Davidsen, Bruce Margon, Ajay K. Kochhar: Narrowband HST images of M87: Evidence for a disk of ionized gas around a massive black hole . In: Astrophysical Journal Letters ( ISSN 0004-637X ) . tape 435 , 1994, pp. L27-L30 , bibcode : 1994ApJ ... 435L..27F .
- ↑ RD Blandford, CF McKee: Reverberation mapping of the emission line regions of Seyfert galaxies and quasars . In: Astrophysical Journal . tape 255 , 1982, pp. 419-439 , bibcode : 1982ApJ ... 255..419B .
- ↑ Steven A. Balbus and John F. Hawley: A powerful local shear instability in weakly magnetized disks. I - linear analysis. II - nonlinear evolution . In: Astrophysical Journal . tape 376 , 1991, pp. 214-233 .
- ↑ EW Bonning u. a .: Accretion Disk Temperatures and Continuum Colors in QSOs . In: Astrophysical Journal . tape 659 , 2007, p. 211–217 , doi : 10.1086 / 510712 , arxiv : astro-ph / 0611263 .
- ↑ YY Zhou et al. a .: Statistical Properties of the Big Blue Bump in Active Galactic Nuclei . In: Astrophysical Journal . tape 475 , 1997, pp. L9 – L12 , bibcode : 1997ApJ ... 475L ... 9Z .
- ^ Francesco Haardt, Laura Maraschini: X-ray spectra from two-phase accretion disks . In: Astrophysical Journal . tape 413 , 1993, pp. 507-517 , bibcode : 1993ApJ ... 413..507H .
- ↑ Richard F. Mushotzky, Christine Done, Kenneth A. Pounds: X-ray spectra and time variability of active galactic nuclei . In: Annual review of astronomy and astrophysics . tape 31 , 1993, pp. 717-761 .
- ↑ Francesco Haardt, Laura Maraschini, Gabriele Ghisellini: A model for the X-ray and ultraviolet emission from Seyfert galaxies and galactic black holes . In: Astrophysical Journal . tape 432 , 1994, pp. L95 – L99 , bibcode : 1994ApJ ... 432L..95H .
- ↑ Y. Tanaka et al. a .: Gravitationally redshifted emission implying an accretion disk and massive black hole in the active galaxy MCG-6-30-15 . In: Nature . tape 375 , 1995, pp. 659-661 , bibcode : 1995Natur.375..659T .
- ↑ M. Dovčiak, V. Karas, T. Yaqoob: An Extended Scheme for Fitting X-Ray Data with Accretion Disk Spectra in the Strong Gravity Regime . In: Astrophysical Journal . tape 153 , 2004, p. 205-221 .
- ↑ K. Iwasawa, G. Miniutti, AC Fabian: Flux and energy modulation of redshifted iron emission in NGC 3516: implications for the black hole mass . In: MNRAS . tape 355 , 2004, pp. 1073-1079 , bibcode : 2004MNRAS.355.1073I .
- ^ RD Blandford, DG Payne: Hydromagnetic flows from accretion discs and the production of radio jets . In: MNRAS . tape 1999 , 1982, pp. 883-903 , doi : 10.1093 / mnras / 199.4.883 .
- ^ RD Blandford, RL Znajek: Electromagnetic extraction of energy from Kerr black holes . In: MNRAS . tape 179 , 1977, pp. 433-456 , bibcode : 1977MNRAS.179..433B .
- ↑ RA Laing: The sidedness of jets and depolarization in powerful extragalactic radio sources . In: Nature . 331, No. 6152, 1988, p. 149. bibcode : 1988Natur.331..149L . doi : 10.1038 / 331149a0 .
- ^ S. Garrington, JP Leahy, RG Conway, RA Laing: A systematic asymmetry in the polarization properties of double radio sources . In: Nature . 331, No. 6152, 1988, p. 147. bibcode : 1988Natur.331..147G . doi : 10.1038 / 331147a0 .
- ^ The Pierre Auger Collaboration: Correlation of the Highest-Energy Cosmic Rays with Nearby Extragalactic Objects . In: Science . tape 318 , 2007, p. 938-943 , doi : 10.1126 / science.1151124 .
- ↑ L. Burtscher et al. a .: A diversity of dusty AGN tori. Data release for the VLTI / MIDI AGN Large Program and first results for 23 galaxies. Astronomy & Astrophysics 558, A149 (2013). bibcode : 2013A & A ... 558A.149B
- ↑ The classification used here is that from Chapter 2 in Peterson 1997.
- ^ Donald Osterbrock: Emission-line spectra and the nature of active galactic nuclei . In: HR Miller, PJ Wiita (Eds.): Active Galactic Nuclei . Springer, 1988, ISBN 978-3-540-19492-7 , pp. 1–18 ( online version on NED ).
- ↑ Thomas A. Matthews, Allan R. Sandage: Optical Identification of 3c 48, 3c 196, and 3c 286 with Stellar Objects . In: Astrophysical Journal . 138, 1963, pp. 30-56. bibcode : 1963ApJ ... 138 ... 30M . doi : 10.1086 / 147615 .
- ↑ Physics: Imagination and Reality (accessed May 8, 2013).
- ↑ John N. Bahcall, Sofia Kirhakos, David H. Saxe, Donald P. Schneider: Hubble Space Telescope Images of a Sample of 20 Nearby Luminous Quasars . In: Astrophysical Journal . tape 479 , 1997, pp. 642–658 , arxiv : astro-ph / 9611163 , bibcode : 1997ApJ ... 479..642B .
- ↑ RD Blandford, MJ Rees: A 'twin-exhaust' model for double radio sources. Twin jet model. 1974. bibcode : 1974MNRAS.169..395B
- ↑ BL Fanaroff, JM Riley: The morphology of extragalactic radio sources of high and low luminosity. bibcode : 1974MNRAS.167P..31F
- ^ Cuno Hoffmeister: 354 new variables . In: Astronomical News . tape 236 , 1929, pp. 242 , bibcode : 1929AN .... 236..233H .
- ^ John L. Schmitt: BL Lac identified as a radio source . In: Nature . tape 218 , 1968, pp. 663 , bibcode : 1968Natur.218..663S .
- ↑ LC Ho, AV Filippenko, WLW Sargent: A Search for “Dwarf” Seyfert Nuclei. III. Spectroscopic Parameters and Properties of the Host Galaxies . In: Astrophysical Journal Supplement . 112, No. 2, 1997, pp. 315-390. arxiv : astro-ph / 9704107 . bibcode : 1997ApJS..112..315H . doi : 10.1086 / 313041 .
- ↑ a b T. M. Heckman: An optical and radio survey of the nuclei of bright galaxies - Activity in normal galactic nuclei . In: Astronomy and Astrophysics . 87, 1980, pp. 152-164. bibcode : 1980A & A .... 87..152H .
- ↑ G. Kauffmann: The discovery of LINERs and a premonition that black holes are found in every galaxy. Commentary on: Heckman ™, 1980, A&A, 87, 152 . In: Astronomy & Astrophysics . tape 500 , 2009, pp. 201–202 , bibcode : 2009A & A ... 500..201K .
- ↑ LC Ho, AV Filippenko, WLW Sargent: A Reevaluation of the Excitation Mechanism of LINERs . In: Astrophysical Journal . 417, 1993, pp. 63-81. bibcode : 1993ApJ ... 417 ... 63H . doi : 10.1086 / 173291 .
- ↑ R. Terlevich, J. Melnick: Warmers - The missing link between starburst and Seyfert galaxies . In: Monthly Notices of the Royal Astronomical Society . 213, 1985, pp. 841-856. bibcode : 1985MNRAS.213..841T . doi : 10.1093 / mnras / 213.4.841 .
- ↑ JC Shields: Normal O stars in dense media generate LINERs . In: Astrophysical Journal Letters . 399, 1992, pp. L27-L30. bibcode : 1992ApJ ... 399L..27S . doi : 10.1086 / 186598 .
- ↑ Section 6 in Kormendy, John; Richstone, Douglas: Inward Bound - The Search For Supermassive Black Holes In Galactic Nuclei . In: Annual Review of Astronomy and Astrophysics . tape 33 , 1995, pp. 581–624 , bibcode : 1995ARA & A..33..581K .
- ↑ John Magorrian et al. a .: The Demography of Massive Dark Objects in Galaxy Centers . In: Astronomical Journal . tape 115 , 1998, pp. 2285-2305 , bibcode : 1998AJ .... 115.2285M .
- ↑ Laura Ferrarese, David Merritt: A Fundamental Relation between Supermassive Black Holes and Their Host Galaxies . In: Astrophysical Journal . tape 539 , 2000, pp. L9 – L12 , bibcode : 2000ApJ ... 539L ... 9F . vs. Karl Gebhardt u. a .: A Relationship between Nuclear Black Hole Mass and Galaxy Velocity Dispersion . In: Astrophysical Journal . tape 539 , 2000, pp. L13 – L16 , bibcode : 2000ApJ ... 539L..13G .
- ↑ Khee-Gan Lee: The Shadow of the Cosmic Web . In: Stars and Space . October, 2014, p. 36 ( online version (fee required) ).
- ^ Roger Lynds: The Absorption-Line Spectrum of 4C 05.34 . In: Astrophysical Journal . tape 164 , 1971, p. L73 – L78 , bibcode : 1971ApJ ... 164L..73L .
- ^ Neil HM Crighton, Joseph F. Hennawi, J. Xavier Prochaska: Metal-poor, Cool Gas in the Circumgalactic Medium of az = 2.4 Star-forming Galaxy: Direct Evidence for Cold Accretion? In: Astrophysical Journal Letters . tape 776 , 2013, p. L18 , bibcode : 2013ApJ ... 776L..18C .
- ↑ Sebastiano Cantalupo, Fabrizio Arrigoni-Battaia, J. Xavier Prochaska, Joseph F. Hennawi, Piero Madau: A cosmic web filament revealed in Lyman-alpha emission around a luminous high-redshift quasar . In: Nature . tape 506 , 2014, p. 63–66 , bibcode : 2014Natur.506 ... 63C .
- ↑ James E. Gunn, Bruce A. Peterson: On the Density of Neutral Hydrogen in Intergalactic Space . In: Astrophysical Journal . tape 142 , 1965, bibcode : 1965ApJ ... 142.1633G .
- ↑ RH Becker u. a .: Evidence For Reionization at z ~ 6: Detection of a Gunn-Peterson Trough In A z = 6.28 Quasar . In: Astronomical Journal . tape 122 , no. 6 , 2001, p. 2850–2857 , doi : 10.1086 / 324231 , arxiv : astro-ph / 0108097 .
- ↑ D. Tytler, XM Fan, S. Burles: Cosmological baryon density derived from the deuterium abundance at redshift z = 3.57 . In: Nature . tape 381 , 1996, pp. 207 ( online version on NED ).
- ^ Robert V. Wagoner: Big-Bang Nucleosynthesis Revisited . In: Astrophysical Journal . tape 179 , 1973, pp. 343-360 , bibcode : 1973ApJ ... 179..343W .
- ↑ JK Webb u. a .: Search for Time Variation of the Fine Structure Constant . In: Physical Review Letters . 82, No. 5, 1999, pp. 884-887. arxiv : astro-ph / 9803165 . bibcode : 1999PhRvL..82..884W . doi : 10.1103 / PhysRevLett.82.884 . MT Murphy et al. a .: Possible evidence for a variable fine-structure constant from QSO absorption lines: motivations, analysis and results . In: Monthly Notices of the Royal Astronomical Society . 327, No. 4, 2001, p. 1208. arxiv : astro-ph / 0012419 . bibcode : 2001MNRAS.327.1208M . doi : 10.1046 / j.1365-8711.2001.04840.x . JK Webb et al. a .: Further Evidence for Cosmological Evolution of the Fine Structure Constant . In: Physical Review Letters . 87, No. 9, 2001, p. 091301. arxiv : astro-ph / 0012539 . bibcode : 2001PhRvL..87i1301W . doi : 10.1103 / PhysRevLett.87.091301 . PMID 11531558 . MT Murphy, JK Webb, VV Flambaum: Further Evidence for a Variable Fine-Structure Constant from Keck / HIRES QSO Absorption Spectra . In: Monthly Notices of the Royal Astronomical Society . 345, No. 2, 2003, p. 609. arxiv : astro-ph / 0306483 . bibcode : 2003MNRAS.345..609M . doi : 10.1046 / j.1365-8711.2003.06970.x . H. Chand et al. a .: Probing the Cosmological Variation of the Fine-Structure Constant: Results Based on VLT-UVES Sample . In: Astronomy & Astrophysics . 417, No. 3, 2004, p. 853. arxiv : astro-ph / 0401094 . bibcode : 2004A & A ... 417..853C . doi : 10.1051 / 0004-6361: 20035701 . R. Srianand et al. a .: Limits on the Time Variation of the Electromagnetic Fine-Structure Constant in the Low Energy Limit from Absorption Lines in the Spectra of Distant Quasars . In: Physical Review Letters . 92, No. 12, 2004, p. 121302. arxiv : astro-ph / 0402177 . bibcode : 2004PhRvL..92l1302S . doi : 10.1103 / PhysRevLett.92.121302 . PMID 15089663 . MT Murphy, JK Webb, VV Flambaum: Comment on “Limits on the Time Variation of the Electromagnetic Fine-Structure Constant in the Low Energy Limit from Absorption Lines in the Spectra of Distant Quasars” . In: Physical Review Letters . 99, No. 23, 2007, p. 239001. arxiv : 0708.3677 . bibcode : 2007PhRvL..99w9001M . doi : 10.1103 / PhysRevLett.99.239001 . MT Murphy, JK Webb, VV Flambaum: Revision of VLT / UVES Constraints on a Varying Fine-Structure Constant . In: Monthly Notices of the Royal Astronomical Society . 384, No. 3, 2008, p. 1053. arxiv : astro-ph / 0612407 . bibcode : 2008MNRAS.384.1053M . doi : 10.1111 / j.1365-2966.2007.12695.x .
- ↑ AH Andrei u. a .: The large quasar reference frame (LQRF): An optical representation of the ICRS . In: Astronomy and Astrophysics . tape 505 , 2009, p. 385–404 , doi : 10.1051 / 0004-6361 / 200912041 , bibcode : 2009A & A ... 505..385A .
- ↑ ESA's astrometric Gaia mission has been running since 2013, see Gaia factsheet at ESA
- ↑ AH Andrei u. a .: Gaia initial QSO catalog: the variability and compactness indexes . In: Memorie della Societa Astronomica Italiana . tape 83 , 2012, p. 930-933 , bibcode : 2012MmSAI..83..930A .
- ^ H. Schuh, D. Behrend: VLBI: A fascinating technique for geodesy and astrometry . In: Journal of Geodynamics . tape 61 , 2012, p. 68–80 , bibcode : 2012JGeo ... 61 ... 68S .
- ↑ Active galactic nuclei help determine distances. In: Spectrum of Science. November 2011. Spectrum of Science Verlagsgesellschaft mbH, Heidelberg. ISSN 0170-2971 .
- ^ D. Watson, KD Denney, M. Vestergaard, TM Davis: A new cosmological distance measure using AGN. September 21, 2011. arxiv : 1109.4632 .
- ^ P. Schneider, J. Ehlers, EE Falco: Gravitational lenses . Springer, 1992, ISBN 3-540-66506-4 .
- ^ D. Walsh, RF Carswell, RJ Weymann: 0957 + 561A, B: twin quasistellar objects or gravitational lens? (pdf) nature 279. pp. 381-384. May 31, 1979. Retrieved April 16, 2011.
- ↑ Section 4.2. in Joachim Wambsganss: Gravitational Lensing in Astronomy. Living Rev. Relativity 1, (1998), 12. ( Online version on LRR , last accessed September 17, 2014).
- ↑ EL Turner, JP Ostriker, JR Gott III: The statistics of gravitational lenses: the distributions of image angular separations and lens redshifts. Astrophys. J., 284, 1-22, (1984). bibcode : 1984ApJ ... 284 .... 1T .
- ↑ DE Lebach, BE Corey, II Shapiro, MI Ratner, JC Webber, AEE Rogers, JL Davis, TA Herring: Measurement of the Solar Gravitational Deflection of Radio Waves Using Very-Long-Baseline Interferometry. Phys. Rev. Lett., 75, 1439-1442, (1995).
- ↑ SS Shapiro, JL Davis, DE Lebach, JS Gregory: Measurement of the solar gravitational deflection of radio waves using geodetic very-long-baseline interferometry data, 1979-1999. Phys. Rev. Lett., 92, 121101, (2004).