High voltage direct current transmission
The high-voltage direct current ( HVDC is) a process of electric power transmission with a high DC voltage .
Technical background
In power plants, electrical energy is almost always generated by synchronous generators as three-phase alternating current with a frequency of 50 Hz or 60 Hz. The transmission of high power (from around 1 GW) over longer distances (over 100 km) using economical and technically manageable cable diameters forces high electrical voltages of over 400 kV. For this purpose, the high voltage is generated with a very high degree of efficiency by power transformers and transformed down to lower voltages (e.g. 110 kV to 20 kV) at the end of the overhead lines in substations .
One of the basic requirements for this transmission with alternating currents, however, is that the capacitance both between the lines and with respect to earth potential remains sufficiently small to keep the reactive power low. In the case of overhead lines , this is achieved by appropriate spacing, but in the case of underground or submarine cables , their capacitive coating does not allow economical operation with alternating voltage at lengths of more than a few tens of kilometers. In this case, transmission with direct current has advantages because the line loss is limited to the ohmic resistance of the active current .
The problems with transmission with direct current are the generation of the high direct voltage and the conversion between alternating and direct current. The conversion of direct current into alternating current and vice versa can be done electromechanically with special electrical machines ( converters ) or electronically with power converters . These converter stations are the cause of the high production costs of HVDC compared to AC voltage transmission, which only pay for themselves with longer system lengths. Even today there are still enormous costs for the insulating materials and semiconductors used or the special converter transformers .
history
The first attempt at long-distance transmission with direct current took place in 1882 from Miesbach to Munich . Smaller and more medium-voltage systems emerged from the 1890s, particularly in Italy and Switzerland , for example St-Maurice - Lausanne (22 kV, 3.7 MW, 60 km; 1897). The first HVDC system was the Lyon – Moûtiers system with a 180 km long overhead line at 100 kV bipolar voltage and 14.7 MW transmission capacity in the final stage. The plant was in operation from 1906 to 1936 and worked without converters. The electrical energy was generated by direct current generators connected in series in a hydroelectric power station in Pomblière near Moûtiers and converted by direct current machines in Lyon to operate the electric tram. All of these systems were based on electro-mechanical converters . It was not until the 30s of the twentieth century that systems with power converters were developed.
The first German HVDC system was the bipolar cable transmission of the so-called Elbe project between the lignite power station Vockerode (near Dessau) and Berlin (symmetrical voltage of 200 kV to earth, maximum transmission capacity 60 MW) , which began in 1941 but never went into operation . This system was dismantled by the Soviet occupying forces and used in 1950 to build a 100-kilometer-long, monopolar high-voltage direct current line with a transmission capacity of 30 MW and an operating voltage of 200 kV between Moscow and Kaschira . This line has now been shut down. A system based on arc converters was planned as a competing project between the substations Umspannwerk Lehrte-Ahlten and Hallendorf (Salzgitter) . With a voltage of 300 kV, this should transmit a power of 150 MW on a reallocated 220 kV overhead line. In contrast, only the HVDC test section Lehrte-Misburg was actually in operation , which achieved an output of up to 12 MW at 80 kV with a transmission length of 5 km using arc converters.
In 1954, an HVDC system was put into operation between the Swedish island of Gotland and the Swedish mainland. The oldest HVDC system still in existence is the Kontiskan 1 between Denmark and Sweden. Essential work to improve HVDC technology was carried out in the 1960s by the Swedish electrical engineer Uno Lamm . The Uno Lamm Award , which has been awarded annually since 1981 by the IEEE Power Engineering Society for essential work in the field of HVDC technology, is named after him .
In 1972 the first HVDC system with thyristors was put into operation in the Canadian Eel River and in 1975 in England the HVDC Kingsnorth between the Kingsnorth power station and downtown London with mercury vapor rectifiers . On March 15, 1979, an HVDC transmission line went into operation between Cahora Bassa in Mozambique and the Johannesburg metropolitan area in South Africa (1420 km) with ± 533 kV and 1920 MW. This line was built by a consortium of AEG, BBC and Siemens. The Fenno-Skan between Sweden and Finland started operating in 1989.
In Germany, the first HVDC system in the form of the HVDC close coupling was built in Etzenricht between 1991 and 1993 . In 1994 the Baltic Cable, a 262 kilometer long direct current line between Lübeck- Herrrenwyk and Kruseberg in Sweden, went into operation, followed in 1995 by the 170 kilometer long fully cabled Kontek between Bentwisch near Rostock and Bjæverskov Sogn in Denmark .
At 580 km, the NorNed connection , which was put into operation at the end of September 2008, between Feda near Kvinesdal in Norway and Eemshaven in the Netherlands is currently (2019) the longest submarine connection. The operators are the Norwegian Statnett and the Dutch Tennet TSO .
In January 2014, commercial operation of the southern HVDC Hami-Zhengzhou with a transmission voltage of ± 800 kV and a transmission capacity of 8000 MW was started in the People's Republic of China between the autonomous region of Xinjiang and the city of Zhengzhou over a distance of 2200 km .
The first 1100 kV HVDC link went into operation at the beginning of 2019. The line between Changji and Guquan has a length of 3284 km and a transmission capacity of 12 GW and as of the beginning of 2019 it is the most powerful HVDC line.
Asea Brown Boveri (ABB), GE Grid Solutions ( joint venture between General Electric and Alstom ) and Siemens are among the largest manufacturers of HVDC systems .
Applications

The high-voltage direct current transmission is used for the transmission of electrical energy in various application areas described below. In the list of HVDC systems there is a tabular listing of various implemented and planned systems.
DC short couplings
If the transmission length of the direct current is only a few meters and both converters are housed in the same building or in immediately adjacent buildings, this is known as an HVDC short coupling (DC short coupling , GKK, English back to back converter ). This form, technically an intermediate circuit, is used for the direct exchange of electrical energy between three-phase alternating current networks that are not operated with a synchronous network frequency and are assigned to different control ranges. Examples of this are the GKK Etzenricht, operated in Germany from 1993 to 1995, or the Châteauguay direct current back-to-back link from Hydro-Québec in Canada . Due to two different network frequencies in Japan, power can only be transmitted between the two frequency systems using HVDC close couplings. One example is the plant in Shizuoka . Since HVDC close couplings do not work without losses and often also determine the maximum possible transmission power, such systems are usually not used within synchronized networks. Existing systems are therefore usually shut down even if two networks that were not previously operated synchronously were synchronized with one another, which enables a direct energy exchange.
Energy transmission over long distances
The HVDC technology is used to transmit energy by direct current over long distances - these are distances of around 750 km upwards - because from certain distances, the HVDC has lower overall transmission losses than transmission with three-phase alternating current, despite the additional converter losses. Examples are the unfinished HVDC Ekibastus Center in Siberia, the 1700 km long HVDC Inga-Shaba in the Congo and the over 1000 km long HVDC Québec – New England between Canada and the USA. In Europe, due to the comparatively short distances between power stations and consumers, there are currently no HVDC systems in this length range.
The main line losses over long distances without converter losses are around 3.7% in implemented systems such as the NorNed (between Norway and the Netherlands) with a transmitted power of 600 MW (85% of the nominal power) and a line length of 580 km, which is about 6 , Corresponds to 4% relative losses over a cable length of 1000 km. For projects that have been considered and not yet implemented, such as Desertec or the European Supergrid , line losses of 14% are assumed for a 5000 km long HVDC line with 800 kV. This corresponds to around 2.8% relative line losses over 1000 km.
The use of HVDC technology is uneconomical for short distances. If the rectifier used and the inverter used together have the loss value v, then this is equivalent to adding an ohmic resistance with the value (3 * v * in the transmission path of a three-phase transmission system operated with voltage U, which transmits power P U * U) / P would insert. In concrete terms, this would be a value of 10.83 Ohm for P = 600 MW, U = 400 kV and v = 0.015. This corresponds to a line with quadruple bundles, in which each sub-conductor has an aluminum cross-section of 340 mm² and a steel cross-section of 30 mm², 509 km in length!
Energy transmission using HVDC submarine cables

The high-voltage direct current transmission is also used to transmit energy over comparatively short distances of a few 10 km to a few 100 km if the transmission cable has a very high capacitive coating due to its design. Operation with three-phase current is then not economical, since a high reactive power would have to be used for constant reloading of the cable capacitance. When connecting power grids and connecting wind farms over the sea, a submarine cable is almost always the only option , which is why HVDC cable systems are almost without exception in this area of application. European examples are the submarine cable NorNed between Norway and the Netherlands, the submarine cable Baltic Cable between Sweden and Germany or BritNed between Great Britain and the Netherlands.
In addition, connections from offshore wind farms that are located a great distance from the coast are mostly connected to the grid via HVDC. With these systems, it is assumed that HVDC systems with a cable length of approx. 55 to 70 km are more economical than a conventional connection using high-voltage three-phase current technology.
There is a special feature of monopolar single-core HVDC submarine cables: the polarity switch occurs when the direction of the power flow changes, where the design of the earthing systems is designed for a fixed current direction. During operation with high DC voltage, space charges accumulate in the dielectric between the inner and outer conductors after a while . This is the result of different levels of electrical conductivity, which in turn is due to the radial temperature gradient from the inner conductor to the cooler outer area. In the event of a sudden change in polarity to reverse the direction of the power flow, the space charges in the dielectric, which are only slowly degrading, would lead to strong field increases that would trigger material- destroying partial discharges in the insulating material. For this reason, with monopolar HVDC submarine cable systems (e.g. HVDC Italy-Greece ), if the direction of the power flow is reversed, a certain period of time must be waited before the line is used again.
Energy transmission via HVDC cables over land
Cables arriving from sea to land are extended on land as underground cables . With some offshore HVDC connections, the land cable route is also longer than the associated submarine cable route.
A first European example of a pure HVDC underground cable connection is the first part of the HVDC connection SüdVästlänken between Norway and southern Sweden. Of this, the Barkeryd – Hurva section is mainly laid along the E4 motorway as XLPE plastic cable, with a nominal voltage of ± 300 kV. The system consists of two parallel HVDC underground cable systems, which together have a transmission capacity of around 600 MW.
Special applications
In addition, the HVDC technology is also used to a lesser extent for special solutions, such as the Flexible AC Transmission System (FACTS) to use the Unified Power Flow Controller (UPFC) technology to target individual lines in three-phase AC networks Carry out load flow controls by means of lateral and longitudinal regulation.
Geological measurements
Existing HVDC systems with a grounded return conductor or grounded center point were also used for geophysical measurements by measuring the return current through the earth at different locations.
execution
Converter systems
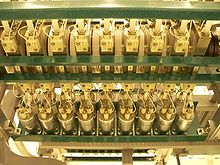
A converter station, also called a converter station, is located at both ends of a high-voltage direct current transmission system. In addition to the control systems, it essentially contains the power converters and, mostly in the outdoor area next to the hall, the power converter transformers , smoothing reactors and harmonic filters . The converters used can usually work in both directions, both as rectifiers and as inverters , and thus transmit energy in both directions. There are also special HVDCs such as the Pacific DC Intertie on the west coast of the USA or the HVDC Inter-Island Link in New Zealand, which only transmit electrical power in one direction during normal operation.
The interior of an HVDC converter hall with the inverter is usually completely metallic shielded from the outside area due to the electromagnetic compatibility and cannot be entered during operation due to the high field strengths and the risk of electric shocks. Thyristors or IGBTs connected in a twelve-pulse circuit are used as power converters in modern systems . Very large mercury vapor rectifiers were used in old systems . In the early days of HVDC technology, experiments were also carried out with electric arc converters , as was the case with the Lehrte-Misburg HVDC test facility . In order to achieve the required blocking voltages of over 500 kV, several dozen thyristors / IGBT are connected in series, as the blocking voltage per thyristor / IGBT is only a few kV for technical reasons. All thyristors connected in series must switch through almost simultaneously within a microsecond in order to avoid damage due to uneven voltage distribution on the inverter.
The thyristors or IGBTs can not be controlled electrically directly because of the potential differences and the high rate of change in voltage, but the signals are transmitted with fiber optic cables. In the systems with mercury vapor rectifiers that are no longer in regular operation today, the ignition pulses were transmitted by means of high frequency.
To dissipate the power loss from the thyristors, liquid coolants such as pure water are used, which are pumped in electrically insulated pipe systems through the converter hall to the individual thyristors. The heat loss is given off to the ambient air in the outside area of the hall in the form of heat exchangers .
The smoothing coil at the direct current output is used to reduce the residual ripple of the direct current. It can be designed as an air or iron throttle . Its inductance is about 0.1 to 1 H .
The transformers on the AC voltage side not only generate the high voltage, they also suppress numerous harmonics with their inductance and switching method (series connection of delta and star connection , see twelve-pulse rectifier ) . Harmonic filters suppress further unwanted harmonics. With systems in twelve-pulse circuit, you only need to suppress the 11th, 13th, 23rd and 25th harmonics. Suction circuits matched to the 12th and 24th harmonics are sufficient for this.
They are also used to generate the reactive power required for commutation . In principle, an HVDC transmission can also be implemented without a harmonic filter, such as B. in the Volgograd station of the HVDC Volgograd-Donbass .
Piping systems and earth electrodes
The transmission can be monopolar as well as bipolar.
- Monopolar in this context means that a direct voltage with a certain nominal value such as B. +450 kV is present, with one pole at each of the two line ends being earthed and therefore one conductor is sufficient (earth as "return conductor").
- Bipolar means that, in contrast to monopolar HVDC, two metallic conductors are used, with grounding at medium potential: one conductor that has a positive voltage in relation to the earth potential and a conductor that has a negative voltage in relation to the earth potential, for example ± 450 kV. In this case, the DC voltage between the two conductors is twice the voltage as between a conductor and earth, i.e. 900 kV in this example. Depending on the design, a metallic return conductor can also be carried along, which is insulated from the earth. Then, in the event of a line failure, continued operation with half the voltage is possible. The bipolar variant has the advantage that the magnetic field of the current in the return conductor compensates that of the outgoing conductor fairly well, as they are oriented in opposite directions. After several meters the field strength is below that of the weak earth's magnetic field, the more the closer the outgoing and return lines are.
In a bipolar system, the grounding of the center potential is used to avoid damage to the insulation due to an uneven voltage distribution between the conductors, since the two conductors are isolated from earth potential. In bipolar systems, the earth electrode carries no operating current, but only a small equalizing current. In the case of a monopolar HVDC, the system's operating current of a few kiloamps is carried through the earth electrode. The earthing system must be correspondingly spacious, with an extension of a few kilometers, and be anchored in the ground with good conductivity, for example near the coast in the sea or near rivers. As with any earth electrode, the area and shape of the earth electrode and the electrical conductivity in the immediate vicinity of the earth electrode are primarily decisive for a low earthing resistance . Due to the large cross-sectional area, the electrical conductivity of the remaining earth material between the two earth electrodes of the HVDC converter systems, which are far from each other, is practically irrelevant.
Bipolar systems can also be designed in such a way that, if required, they can also be operated as two monopoles connected in parallel or as a single monopole. This was realized at the HVDC Inga-Shaba . Without a metallic return conductor, the direct current then leads to electrolytic decomposition on the earth electrode, depending on the direction of the current and the material used . The anode in particular is subject to a decomposition process, similar to a sacrificial anode , which is why it is made, for example, of petroleum coke or in the form of titanium meshes. Cathodes can be designed as large bare copper rings. Numerous bipolar systems are designed so that monopolar operation is also possible. If, as in these cases, electrodes are to serve as both a cathode and an anode, they must all be designed to be corrosion-resistant or, alternatively, a metallic return conductor must be available.
HG overhead lines usually have two conductors. For a later bipolar expansion, monopolar lines are often equipped with two conductor cables which, as long as the bipolar expansion has not been completed, are connected in parallel or one of which serves as a low-voltage conductor for the earth electrode. The one-level arrangement of the conductors is almost always used.

The conductor for the grounding electrode can also function as a grounding wire , as it is grounded with a very low resistance via the grounding electrode. However, in order to avoid electrochemical corrosion of the masts, it must be attached to the masts in an insulated manner. Spark gaps on the insulators are therefore required to divert lightning currents.
To avoid electrochemical corrosion, the grounding electrode must not lie directly next to the line route, so that a separate route is necessary for at least the last piece of the electrode line. As in the case of non-parallel laying of the electrode line to the high-voltage road, this can be designed either as an overhead line (similar to a medium-voltage line), as an underground cable or as a combination of overhead line and underground cable. The insulation of the electrode line is usually designed for an operating voltage of around 10–20 kV (medium voltage range).
advantages

In the widespread three-phase networks , connections with at least three conductor strands are always necessary. In contrast, direct current transmission manages with two, and when using earth as the second pole, even only one conductor. This saves high costs for both the line material and the overhead line system (masts and insulators, etc.). In addition, HVDC lines can transmit significantly more power than AC systems, so that HVDC lines can be built more than half narrower with the same transmission power.
The HVDC allows energy to be transmitted over long distances through submarine cables . Due to the principle-related structure of a cable with outer shielding and inner conductor, an undersea cable has a high capacitance per unit length compared to an overhead line . With alternating voltage , this generates reactive currents that put additional stress on the cable. In the case of three-phase lines, reactive power compensation of the line is necessary so that the cable is loaded with the natural power . Compensation coils must therefore be installed along the line at certain intervals . With submarine cables, this is only possible with great technical effort. This is why HVDC is used from a transmission length of around 70 km underwater. The differences are shown in the adjacent picture and are explained using the transmission of 1500 MVA over a distance of 500 km.
- With an overhead line, the total reactive power is 500 km · 3.8 Mvar / km = 1900 Mvar (inductive).
- In a cable system, the total reactive power is 500 km * 8 Mvar / km = 4000 Mvar (capacitive).
- With direct current there is no reactive power.
With direct current, the skin effect does not appear, which with alternating current leads to the current being displaced to the edges of the line cross-section. Therefore, large cable cross-sections can be used better than with a comparable alternating current transmission.
With DC voltage, there are no dielectric losses in the cable insulation . In overhead lines, the losses due to corona discharges are significantly lower with direct voltage than with an equally high alternating voltage; In the case of alternating voltages, they require bundle conductors even at lower voltages above about 100 kV in order to reduce the field strength on the conductor surface.
While synchronization is absolutely necessary within an alternating current network, this is not necessary with direct current transmission. HVDC is also sometimes used on interconnections in a large spatially extended synchronous AC network. An example of such a route is the HVDC Italy-Greece within the synchronous European network between the Italian town of Galatina and the approximately 300 km distant town of Arachthos in Greece - however, HVDC is necessary here because of the length of the submarine cable.
In addition, the insulation in the direct current network does not have to be designed for a peak value of , but only for .
disadvantage
The disadvantage is the higher technical effort with direct current for the power converter (current converter) compared to a transformer . The converter stations are compared to three-phase transformers also little overloaded. The converter transformers installed in the outside area of the converter station generate more noise than comparable three-phase transformers due to the harmonics .
In the case of short connections, the losses that occur in the converter are greater than the reduction in the losses in the line through the use of direct current, which is why HVDC usually does not make sense for short transmission routes. The exceptions are the HVDC close couplings, with which asynchronous three-phase networks can only be connected with direct current technology and with the high converter losses.
In the case of high DC voltages, problems arise due to inhomogeneous insulating distances, e.g. B. also from pollution and wetting by rainwater (outdoor systems) on the insulator surfaces and conductor bushings. Inhomogeneities in insulating materials and on surfaces lead, unlike with alternating voltage, to a distortion of the electrical field. For this reason, significantly longer isolators are used with HVDC than with AC voltage. Insulating materials and insulator constructions must have special properties in order to maintain the homogeneity of the field. The cause of the field distortion are inhomogeneous specific volume and surface resistances, which in turn are strongly dependent on temperature and field strength.
outlook
Continental electricity grids based on HVDC technology are seen as an important component of renewable energy systems, as they are able to partially compensate for the regionally different feed-in of renewable energies and thus reduce the need for electricity storage. As an alternative to HVDC technology with line-commutated converters with an intermediate circuit , technologies with self-commutated converters with a voltage intermediate circuit are increasingly being used. IGBTs , for example, are used as switching elements . Such systems have so far only been used for smaller services.
In Germany, the network development plan contains several projects for the construction of HVDC lines. The following lines are planned and laid down in the Federal Requirements Plan Act, which should primarily be carried out as underground cables:
- Project 1: A-North from Emden Ost in Lower Saxony to Osterath in North Rhine-Westphalia ( Amprion project developer ), planned commissioning in 2025
- Project 2: Ultranet , from Osterath to Philippsburg in Baden-Württemberg (project sponsors Amprion and TransnetBW ), planned commissioning in 2024
- Project 3: South link from Brunsbüttel in Schleswig-Holstein to Großgartach in Baden-Württemberg (project developer Tennet and TransnetBW), planned commissioning in 2026
- Project 4: South link from Wilster in Schleswig-Holstein to Grafenrheinfeld in Bavaria (project developer Tennet and TransnetBW ), planned commissioning in 2026
- Project 5: South-east link from Wolmirstedt in Saxony-Anhalt to the Isar substation in Bavaria (project sponsor 50Hertz Transmission and Tennet), planned commissioning in 2025
In November 2012, ABB announced that it had developed a DC circuit breaker for high voltages and currents and that it wanted to use it in pilot projects. ABB claims to have equipped 70 HVDC systems and thus half of the HVDC systems installed worldwide. This would make the construction of a meshed HVDC network much easier. The circuit breaker consists of a combination of electronic and mechanical elements.
In order to simplify the necessary approval procedures for HVDC lines, consideration is also being given to replacing existing or planned three-phase lines with HVDC lines.
Superconducting cables, which can be operated either with three-phase or direct current, promise an even significantly lower space requirement than HVDC lines and thus greater public acceptance . The technology, which has even lower losses compared to HVDC, is still at the beginning of its commercialization; as of December 2015, there are only a few implemented applications in the short-haul range.
An approximately 1000 km cable is being considered to carry electricity from geothermal power plants in Iceland to the UK.
HVDC networks
Direct current networks must be controlled differently than three-phase high-voltage transmission systems . In meshed alternating current networks such as the Verbundnetz , the load flows in individual lines are controlled by targeted phase shifts. In the interplay with the reactive power present on the lines, this results in which power plant feeds which part of the total power into the network.
In contrast, direct current networks can only be controlled via the level of electrical voltage at certain nodes in the network. The current then follows the voltage according to the resistance of the lines between the individual stations: If a feeding station wants to feed in more current, it has to increase its voltage accordingly; if a drawing station wants to draw more current (and therefore more power) it has to lower their voltage accordingly. In principle, the HVDC converter stations are already able to adjust their voltage in small steps accordingly, but the necessary control software still has to be implemented.
Another problem is that in a meshed HVDC network there must be a possibility to disconnect a defective HVDC converter station or a defective HVDC cable from the network in the event of a short circuit, even under load, so that the other parts of the network can continue to work safely . However, mechanical AC circuit breakers are not suitable for direct current. The reason for this is the high voltages and currents that are more than sufficient to ignite an arc between the two poles of an opening switch . Due to the inductance of the line, the voltage at the end of the line even rises above the nominal voltage at the moment of disconnection. It is therefore even desirable that the arcing, which is unavoidable due to the inductive voltage rise, occurs in the (encapsulated) switch and not in other parts of the system where arcing can cause severe damage. At 50 Hz mains frequency, however, the voltage drops to zero one hundred times per second, and during these zero crossings the arc in the switch is extinguished by itself. Suitable media in the switch (usually transformer oil and / or the SF 6 gas ) then prevent the arc immediately re-ignites. This means that AC circuit breakers can reduce the current flow to below 1 A within 10 ms, even in the event of a short circuit. Mechanical isolators then completely interrupt the flow of current in a second step. With direct current, however, there are no zero crossings and the arc does not extinguish.
Meshed HVDC grids for building intelligent power grids are the subject of research as of 2017 ( CIGRE WG B4.52 and others). In April 2013, CIGRE Working Group B4.52 presented a feasibility study for HVDC networks. In it, essential parameters of HVDC networks are examined. The study comes to the conclusion that HVDC grids are feasible and the HVDC converter stations will not be more expensive than those currently installed. In order to implement further technical aspects of HVDC networks, the CIGRE Working Grops B4.56 to B4.60 were founded as part of the study. They deal with grid codes , model development for converters, load flow and voltage regulation, network protection and reliability of HVDC Dealing with networks.
Hybrid circuit breakers are also available which, through the combination of mechanical switches and electronic IGBTs, can reliably switch off a direct current connection at 320 kV nominal voltage, 2 kA nominal current and 9 kA short-circuit current within 5 ms. Higher voltages can be achieved by connecting several switches in series, higher currents by connecting in parallel. In the closed case, the losses of this hybrid switch are around 0.01% of the power flowing through it.
As of 2016, there are individual HVDCs with simple branches such as SACOI (HVDC Italy-Corsica-Sardinia).
See also
literature
- Dietrich Oeding , Bernd R. Oswald : Electrical power plants and networks . Verlag Springer, Berlin 2004, ISBN 3-540-00863-2 , p. 838ff ( limited preview in the Google book search).
- H. Wayne Beaty , Donald G. Fink : Standard Handbook for Electrical Engineers . McGraw-Hill, ISBN 978-0-07-144146-9 , chapter 15
- Adolf J. Schwab : electrical energy systems. Generation, transport, transmission and distribution of electrical energy . 3. Edition. Springer, Berlin 2012, ISBN 978-3-642-21957-3
Web links
- High voltage direct current transmission. Proven Technology for Power Exchange (PDF file; 3.55 MB). Siemens, 2008 (technical application note; Eng.)
- OffshoreGrid: Offshore Electricity Infrastructure in Europe (PDF file; 7.37 MB) Study on the planned offshore grid in the North Sea at www.offshoregrid.eu. Accessed on October 7, 2011.
- dena grid study II (PDF file; 13.56 MB), German Energy Agency, 2010, p. 204ff
- Announcement of a recommendation by the Radiation Protection Commission (biological effects of emissions from high-voltage direct current transmission lines [HVDC] of September 12, 2013) of February 25, 2014, BAnz AT August 7 , 2014 B3
References and comments
- ↑ Electrosuisse: René Thury (PDF; 31 kB)
- ^ Karl Baudisch: Energy transmission with high direct current, high voltage . Springer-Verlag, Berlin Heidelberg 1950, p. 292 ff
- ↑ Dieter Kind: Erwin Marx and his contribution to the development of high-voltage direct current transmission from 1930 to 1945 , Braunschweig 2013, urn: nbn: de: gbv: 084-13041515485
- ↑ NorNed. At 580 kilometers, it is the world's longest underwater cable line. tennet.eu, accessed on May 4, 2019 .
- ↑ Southern Hami - Zhengzhou UHVDC project is put into commercial operational ( Memento of 20 April 2014 Internet Archive ). State Grid Corporation website , accessed May 8, 2014
- ↑ World's Biggest Ultra-High Voltage Line Powers Up Across China . In: Bloomberg News , January 2, 2019. Retrieved April 2, 2019.
- ↑ The world's first 1100 kV HVDC transformers. July 11, 2016, accessed April 2, 2019 .
- ↑ A new age in HVDC technology. Siemens, accessed April 2, 2019 .
- ↑ Outaouais DC close coupling ( memento of the original from November 20, 2008 in the Internet Archive ) Info: The archive link was automatically inserted and not yet checked. Please check the original and archive link according to the instructions and then remove this notice. , Hydro-Québec, technical description
- ^ Jan-Erik Skog, Kees Koreman, Bo Pääjärvi, Thomas Worzyk, Thomas Andersröd: The NorNed HVDC Cable Link. A Power Transmission Highway Between Norway And The Netherlands (PDF; 504 kB)
- ↑ Volker Quaschning , Regenerative Energy Systems. Technology - calculation - simulation. Munich 2011, p. 162
- ↑ Mikel De Prada Gil et al., Feasibility analysis of offshore wind power plants with DC collection grid . In: Renewable Energy 78, (2015), 467-477, p. 467, doi: 10.1016 / j.renene.2015.01.042 .
- ↑ Andreas Küchler: High voltage technology: Basics - Technology - Applications . 3. Edition. Springer, 2009, ISBN 978-3-540-78412-8 , pp. 99, 100 and 428 .
- ↑ Interaction of electromagnetic fields of ELF controlled sources with the ionosphere and Earth's crust. Proceedings of the All-Russian (with the International Participation) Research and Practice Workshop. Soot. Acad. Sci., Depart. of Earth Sci., Geological Institute Kola Science Center; Ed.-in-Chief Acad. RAS EP Velikhov, Deputy Ed.-in-Chief Dr Sci. Yu.L. Voytekhovsky. - Apatity, 2014, accessed January 11, 2018.
- ↑ HVDC Inter-Island , Grid New Zealand (Eng.)
- ↑ Clark W. Gellings, Let's Build a Global Power Grid . In: IEEE Spectrum , July 28, 2015. Retrieved July 29, 2015.
- ↑ Bernhard Lutz: Influential factors on the electrical field distribution in insulation systems with polymeric insulating materials with direct voltage load , dissertation at the Technical University of Munich 2011, pages 36ff
- ^ Kuhn et al .: Challenges and opportunities of power systems from smart homes to super-grids . In: Ambio . tape 45 , 2016, p. 50-62 , doi : 10.1007 / s13280-015-0733-x .
- ↑ Please switch . In: Technologie Review, Heise Verlag , January 14, 2013, accessed December 17, 2013
- ↑ Network expansion - BBPlG 1. Accessed on January 21, 2019 .
- ↑ Network expansion - BBPlG 2. Accessed on January 21, 2019 .
- ↑ Network expansion - BBPlG 3. Accessed on January 21, 2019 .
- ↑ Network expansion - BBPlG 4. Accessed on January 21, 2019 .
- ↑ Network expansion - BBPlG 5. Accessed on January 21, 2019 .
- ↑ ABB solves the 100-year-old central puzzle in electrical engineering . In: ABB Press Service , November 7, 2012, accessed on February 21, 2013
- ↑ HDVC Breaker Description
- ↑ see the amendment to the 26th BImSchV and the information on high-voltage direct current transmission (HVDC) from the Federal Office for Radiation Protection
- ↑ https://www.smarterworld.de/smart-energy/smart-grid/artikel/119019
- ↑ Heiko Thomas et al .: Superconducting transmission lines - Sustainable electric energy transfer with higher public acceptance? In: Renewable and Sustainable Energy Reviews . tape 55 , 2016, p. 59-72 , doi : 10.1016 / j.rser.2015.10.041 .
- ↑ Iceland wants to become a “green battery” for the British with submarine cables . ORF.at from January 10, 2013
- ↑ Functionality and areas of application of the hybrid DC circuit breaker , presentation by ABB, accessed on September 21, 2017
- ↑ CIGRE Working Group B4.52, Gunnar Asplund et al: Technical Brochure TB 533 HVDC Grid Feasibility Study pdf, 189 pages, 10.8 MB
- ↑ The Hybrid HVDC Breaker , Magnus Callavik, Anders Blomberg, Jürgen Häfner, Björn Jacobson, ABB Grid Systems, Technical Paper Nov'2012, accessed on September 21, 2017