Phloem
The phloem [ flo 'e: m ] (from ancient Greek φλοῦς <φλό-ος phlóos , German , Bast, bark' ) or the screen member is that portion of a vascular bundle in vascular plants , of the screening elements, i.e. the assimilate conductive cells , and the accompanying parenchymal and consolidation cells . In trees , the active phloem is often referred to as bast .
The most important substances transported are sugar (mainly sucrose ) and amino acids . They are transported from the places of their production ( source , mainly the leaves , but also storage organs in the mobilization of nutrients) to the places of consumption ( sinks , storage organs, growing organs). The vascular bundles and thus also the phloem run through all organs of the plants. The transport takes place in special cells within the phloem: the sieve tube members in bedecktsamern , or the sieve cells in the other vascular plants. The sieve tube members form a functional unit with the escort cells, whereby the transport takes place in the sieve tubes and the escort cells are responsible for the metabolism .
According to its main function, the phloem is divided into three sections: in the collective phloem, mostly in the small vascular bundles of the photosynthetically active leaves, the phloem is loaded. In the transport phloem, long-distance transport takes place; in the release phloem, the transported substances are released to the surrounding cells.
Occurrence
A phloem in the true sense only occurs in vascular plants. It is part of the vascular bundle that contains the xylem in addition to the phloem . In the case of mosses , the haploid moss plants of many deciduous mosses have elongated cells that serve to conduct the assimilation and are referred to as leptoids .
Cell types
In vascular plants, a number of cell types occur in the phloem , which differ in shape and function.
Sieve elements
The sieve elements are the actual guide elements in which the transport takes place. They are specialized cells . In the case of the ferns and the naked samers these are sieve cells, in the case of the bedecktsamern sieve tubes composed of several members.
Sieve tubes

si = transverse walls of the young sieve tubes
pp = phloem parenchyma
cc = cambium
The sieve tubes of the bedecktsamer consist of individual cells, the sieve tube members. Together with the escort cells, they form the functional unit of the sieve tubes / escort cell complex. In contrast to the water-conducting vessels in the xylem, functional sieve tube members are living cells, but they differ significantly from an average plant cell: They are characterized by the lack of a cell nucleus . A vacuole is also missing, and ribosomes , cytoskeleton and Golgi apparatus are also absent in mature, functional sieve tube members. Plastids are present, mitochondria are few and probably not functional. The sieve tube members are dependent on the support of the escort cells due to their incomplete equipment.
In the course of cell maturation, the originally present cell nucleus dies and dissolves. In some species the chromatin is retained as an amorphous mass. The tonoplast that surrounds the vacuole also dissolves. However, the cell membrane remains intact. Van Bel described this process as “programmed cell half-death” . The endoplasmic reticulum (ER) and the proteins remain in the wall and are not captured by the transport stream. Seven nanometer long anchor molecules have been discovered that anchor ER, mitochondria, plastids to each other and to the cell membrane. Compared to the other organelles, the ER is well developed and is in the form of a network or stack and is called sieve element ER (SER). The ER may also serve to transport proteins from the escort cells into the sieve tubes. The central area of the cells where transport takes place is called the lumen. Due to the high sucrose concentration , the sieve tubes are subject to a high turgor that can reach up to 1.5 MPa (15 bar).
Sieve tubes are often short-lived, but can remain functional in palm trees for 30 years. The length of sieve tube members in secondary phloem in dicotyledons is between 100 and 500 micrometers with a diameter of 10 to 70 micrometers. In monocots, the sieve tube members in yams ( Dioscorea ) are 100 micrometers long and 5 to 10 micrometers wide, and in some palms 5000 micrometers (5 mm) long and 400 micrometers wide. The transverse walls of the sieve tube members are inclined to different degrees depending on the type and perforated like a sieve. Sieve pores with a much larger diameter (one to 14 micrometers) develop from the plasmodesmata of the young cells. In young sieve tubes, the pores are often surrounded by callose , which later largely dissolves. The sieve pores are in sieve fields or, if several sieve fields are on a transverse wall, in sieve plates. The plasmalemma of the cells passes through the pores, but not the endoplasmic reticulum.
Up to now 150 to 200 soluble proteins have been detected in sieve tubes exudates . These include all the enzymes involved in glycolysis , proteins involved in protein breakdown ( ubiquitin ), oxygen radical scavengers ( glutaredoxin , glutathione reductase ), and various proteinase inhibitors (as a defense against herbivores ). Much of the protein seems to be linked to stress and defense reactions. The so-called P proteins (P for phloem) are characteristic of sieve tubes. In young sieve tubes, these are formed as protein bodies. In most species, these protein bodies dissolve at maturity, the proteins remain in the marginal plasma. The protein bodies are retained as such in around 10 percent of the species examined. In butterflies these are spindle-shaped, crystalline and dissolve in the event of injury or rapid turgor change in the sieve tube - generally signals to close the sieve plates to prevent the loss of phloem sap - whereby this dissolution is reversible. In other species, too, the P proteins detach from the wall when damaged and close the sieve pores. Other possibilities of closing the sieve pores are the “exploding” of the plastids and the formation of callose , whereby the formation of callose is viewed more as the permanent and slowly formed closure possibility .
Sieve tube plastids
The plastids of the sieve tubes are divided into two groups: P-type plastids contain protein bodies, S-type plastids lack these. In the Nacktsamern, the pine family (Pinaceae) are the only ones to have P-plastids, all other families have the S-type. Of 382 dicotyledonous families examined , 320 without exception have the S-type, only 48 have the P-type, and 14 families had species with both S and P-types. In the carnation-like (Caryophyllales) plastids of the P-type with filamentous protein inclusions predominate, in the monocot plants the P-type with crystalloid protein inclusions. The function of sieve tube plastids in general as well as the meaning of the individual types is unclear, a role in the wound reaction and as a storage organelle is discussed.
Sieve cells
Sieve cells occur in the nude Samers. At least in the pine-like species there is a core residue in the sieve cells. The sieve tube plastids belong to the P or S type, a vacuole is missing as well as ribosomes, a cytoskeleton or Golgi apparatus. The endoplasmic reticulum resembles that of the covered ones. In vascular spore plants normal plastids are present, the mitochondria are functional. The length of the sieve cells in pine trees is between 1400 and 4850 micrometers. The transverse walls between the sieve cells are inclined and, like the longitudinal walls, finely perforated in so-called sieve fields. In recent as well as fossil naked samers, the sieve cells are quite uniform.
Sieve elements of vascular pore plants
In the vascular pore plants ( bear moss plants and ferns ) the sieve elements are similar to elongated parenchymal cells. The diameter is around 10 micrometers, rarely more than 40 micrometers. The length remains below 600 micrometers. The cell wall between the sieve elements is vertical or slightly inclined, the sieve surfaces are small and variable, the sieve pores have a diameter of around one micrometer. The structure of the sieve elements is quite uniform for all representatives, both recent and fossil.
Escort cells
The escort cells are small parenchymatous cells and occur only in the coversamers. They contain a cell nucleus and numerous mitochondria . They are connected to the sieve tubes by numerous plasmodesmata. These plasmodesmata are special compounds and are called PPUs ( pore- plasmodems unit): They are branched and have up to 100 branches on the escort cell side, which unite in the middle to form a central cavity Form a slightly wider channel on the sieve tube side. The endoplasmic reticulum extends through the PPUs. Many, if not all, of the sieve tube proteins are synthesized in the escort cells and transported into the sieve tubes via the PPUs. Proteins up to 100 kDa can pass, probably due to special proteins that allow large molecules to pass through.
There are different forms of escort cells that are related to the type of phloem load. According to Yuri Gamalei, three types are distinguished according to the number of plasmodesmata between the surrounding parenchyma and the escort cells, who divided around 1000 species in this way. These are mostly family-specific :
- Type 1 has many plasmodesmata (10 to 60 per µm 2 cell surface). This type occurs mainly in the basal representatives of the Bedecktsamer and is therefore regarded as original. It occurs mainly in wood plants.
- Type 1–2a has a relatively high number of plasmodesmata (10 to 0.1 per µm 2 ). This type is found widely in the pedigree of the bedspreads.
- Type 2 has few plasmodesmata (less than 0.1 per µm 2 ). Type 2a, the common escort cells, are widespread, especially in monocots (not in palms , which have type 1–2a) and many useful plants. Type 2b are the transfer cells described in the next paragraph.
The following types are distinguished according to the morphology of the escort cells:
- Transitional cells ( intermediary cells ) have small vacuoles and plastids rudimentary without thylakoids or strength . They often correspond to type 1. The numerous plasmodesmata are formed in leaves through existing cell walls, shortly before the leaf becomes a carbohydrate exporter. All representatives with transition cells form carbohydrates of the raffinose family as transport substances. These do not occur in the surrounding mesophyll, so they do not diffuse through the plasmodesma and thus enable a higher carbohydrate concentration in the sieve tube-escort cell complex than in the surrounding area. Representatives are about the cucurbits , olive trees ( Olea ) and mullein ( Verbascum ), spindle bushes ( Euonymus ), and hydrangeas ( Hydrangea ).
- Smooth cells do not form any vacuoles or cell wall indentations. They mostly correspond to type 1–2a and contain sucrose and, in some cases, sugar alcohols as transport carbohydrates.
- Transfer cells have indentations on the inside of the cell wall, which lead to an increase in the cell surface. They correspond to type 2b. Transfer cells are characterized by a significantly higher sucrose concentration than the neighboring parenchymal cells. Escort cells of this type are referred to as transfer cells of type A, while type B are parenchymal cells of the same structure, whose function is possibly in the release of sucrose in the apoplasts.
Ontogenetically , sieve tubes and escort cells arise as a common complex of common parent cells, which each divide into the larger sieve tube member and the smaller escort cell by unequal division.
In ferns and naked samers, there are also parenchymal cells that are closely connected to the sieve cells, but do not arise from the same mother cell. These cells are known as protein or Strasburger cells . The Strasburger cells are connected to the other parenchymal cells by many plasmodesmata.
Sclerenchymal cells

v = sieve tube, v * = sieve plate in a tube
c = escort cell, p = bast parenchyma, k = crystal-bearing cell
l = bast fibers, r = medullary ray
Sclerenchymal fibers are common in primary and secondary phloem. In the primary phloem (before the secondary growth in thickness ) they appear mostly on the outside, in the secondary phloem (after the secondary growth in thickness) they are distributed differently. The fibers may or may not be septate into multiple cells lengthways, and they may be alive or dead when fully differentiated.
Often you can also find sclerids . They occur alone or together with the fibers. They can be oriented axially or radially. They arise in older parts of the phloem through sclerification of parenchymal cells. The sclereids can lengthen and branch.
Parenchyma
The parenchymal cells store various substances, including starch , tannins and crystals. In the secondary phloem, a distinction is made between axial parenchyma and radiation parenchyma. Crystal cells have thickened cell walls.
Ontogeny
In the course of ontogeny , the different tissues in the embryo are differentiated approximately simultaneously with the formation of the cotyledons , whereby the vascular bundles from the procambium ( plasma-rich cells with small vacuoles) arise in the cotyledons and the hypocotyl- root axis. The vascular system of the seedling arises from this through enlargement and differentiation.
Primary phloem
The primary phloem is the phloem before the secondary growth in thickness. It consists of a protophloem and a metaphloem. The protophloem is formed in the youngest parts of the plant that are still elongated: in the elongation zone of the stem axis and the root. The protophloem is usually formed earlier than the protoxylem. The sieve elements of the protophloem already consist of seedless cells, which, however, are narrow and inconspicuous. Escort cells may be missing. With further growth, the cells of the protophloem are stretched and lose their functionality. Often there are elongated parenchymal cells in the protophloem, which stretch along with the growth and after the loss of the sieve elements in the protophloem differentiate into fiber cells. These fibers at the edge of the phloem are called pericyclic fibers.
The metaphloem differentiates after the end of elongation growth and forms the only existing phloem in plants without secondary growth in thickness, such as herbaceous plants .
In vascular spore plants, the phloem has a much simpler structure and consists only of sieve elements and parenchymal cells.
Secondary phloem
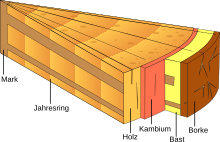
The secondary phloem is formed as part of the secondary growth in thickness of shoot axes and roots. It encompasses all of the tissue released from the cambium and is called bast . In general, it is much less pronounced compared to the wood = xylem part. The old phloem, which is on the outside, is compressed more and more as the thickness grows. The phloem, which is no longer functional, is often separated from the axis by a periderm .
Only the innermost part of the secondary phloem, located near the cambium, is functional.
Transported substances

The content of the sieve tubes is a 0.5 to 1 molar aqueous solution in which the carbohydrates predominate. The main carbohydrate is sucrose (100 to 300 g L −1 ). There is a lack of reducing carbohydrates.
Plant families that transport carbohydrates exclusively or almost exclusively in the form of sucrose are the ferns, the bare pods, the monocots and the butterflies.
In addition to sucrose, a number of clans transport significant amounts of members of the raffinose family (raffinose, stachyose and verbascose , rarely also ajugose ), for example in cucurbits (Cucurbitaceae), linden ( Tilia ), hazel ( Corylus ), elm ( ulmus ) and olive trees ( Olea ). Furthermore, in the family Bignoniaceae (Bignoniaceae), celastraceae (Celastraceae) clethraceae (Clethraceae) combretaceae (Combretaceae), Labiatae (Lamiaceae), Myrtle (Myrtaceae), Onagraceae (Onagraceae), Rutaceae (Rutaceae) and verbena plants (Verbenaceae).
Other clans transport sugar alcohols . Ash ( Fraxinus ) and lilac ( Syringa ), for example, contain D- mannitol . The subfamilies Spiroideae , Maloideae and Prunoideae within the rose family contain sorbitol . The third sugar alcohol is dulcitol , which z. B. occurs in Celastraceae. Little is known about the physiology of the sugar alcohols in the phloem.
Reduced glutathione serves as the transport molecule for sulfur . The pH value is quite high at 7.4 to 8.7. Nitrogen-containing organic compounds are amino acids and amides, especially glutamate / glutamine and aspartate / asparagine . Nitrate and ammonium are absent in the phloem. Proteins and hormones are also transported via the phloem.
A number of mineral nutrients are also transported in the phloem, but these are of different phloem mobility. In addition to the sulfur and nitrogen already mentioned, potassium and magnesium are present to a large extent in the phloem, and phosphorus and chlorine are also good phloem -mobile. Iron , zinc , copper , boron and molybdenum are phloem-mobile to a limited extent . Calcium and manganese are hardly phloem-mobile . All elements except these two are mobilized before the autumn leaves fall and transported out of the leaf via the phloem. The phloem transport of inorganic ions is important for the supply of hardly perspiring, growing organs such as fruits and root tips. For calcium, the white lupine is an exception, which due to the high concentration of organic acids (especially succinate ) also has high calcium concentrations in the phloem.
The sieve tubes have a remarkably high concentration of ATP . They transport vitamins that can only be produced by photosynthetically active cells in plants and have to be transported to the heterotrophic organs, such as thiamine , niacin and pantothenic acid . Plant hormones such as indole acetic acid , gibberellins and cytokinins are also transported via the phloem and act as remote signals. Some proteins and also transported mRNA are regarded as remote signals .
In the phloem, insecticides and herbicides are transported on foreign substances , as are parasites and some viruses .
Phloem load
In order to be able to be transported to the places of consumption (sinks) , the carbohydrates and amino acids must get from the organs of their formation (sources) into the sieve tubes. The uptake takes place mainly in the finest vascular bundles of the photosynthetically active leaves, these areas are called collective phloem. The phloem load of sucrose has been studied best. In the area of the collecting phloem, the cross-section of the escort cells is much larger than that of the sieve tubes. There are basically two ways in which the transport substances can get from the mesophyll cells , where they are produced, into the sieve tubes: via the symplastic and the apoplastic route:
Apoplastic phloem load
In species with apoplastic phloem load there is usually no or hardly any plasmodesma between the parenchyma and the escort cells (type 2). The escort cells are usually designed as transfer cells. The sucrose is released by the parenchymal cells into the intercellular area ( apoplasts ) and actively taken up by the escort cells. To increase the surface area, the cell wall and thus also the plasmalemma of the escort cells are strongly arched. The inclusion of sucrose is performed by sucrose-H + - symporter against the concentration gradient. The energy required for this is provided by outwardly directed H + - ATPases , which build up a proton gradient with the use of energy . The protons flow in the direction of the proton gradient together with the sucrose (this against its gradient) through the sucrose-H + symporter into the transfer cell. The ATPases are mainly localized in the escort cells. The sucrose gradient achieved in this way is much stronger than the sugar gradient in the case of symplastic loading. The inflow of water into the sieve tubes / escort cell complex, which is necessary for mass transport, is facilitated by aquaporins .
Symplastic phloem load
Here the sucrose is transported between the cells via the connecting channels , the plasmodesmata . So the sucrose never leaves the symplast . It reaches the sieve tubes from the mesophyll cells via the escort cells. There are many plasmodesmata between the escort cells and the surrounding parenchymal cells. The assumption that all species with many plasmodesmata, i.e. with type 1, are species with a symplastic phloemic load, has turned out to be incorrect.
Clearly symplastic phloem loaders are species that transport carbohydrates of the raffinose family. With them sucrose gets through the plasmodesmata into the escort cells, which are transitional cells here. In the escort cells, the sucrose is converted into raffinose and the other members of the family. These higher molecular sugars can no longer get back through the plasmodesmata into the parenchyma (so-called polymer trap ). They enter the sieve tubes through the larger plasmodesmata. This enables the build-up of a higher carbohydrate concentration in the sieve tube / escort cell complex than in the surrounding parenchyma.
In some species, which were considered to be symplastic phloemic load due to their numerous type 1 plasmodesma, a predominantly apoplastic load could be detected. These types almost only transport sucrose. The few species examined show high plasmodesma densities in the entire leaf mesophyll, the plasmodesma of the escort cells probably have no connection with the phloem load. The backflow of sucrose from the escort cells into the parenchyma is possibly achieved by temporarily closing the plasmodesmata. Possibly all or many of the Type 1 species that do not carry raffinose are apoplastic loaders.
Otherwise, in species with a symplastic load, but which only transport sucrose, the problem is how the sucrose gets into the escort cells. Active transport processes for small molecules such as sucrose are not known for plasmodesma. One possibility for the functionality is a general sucrose gradient from the mesophyll to the sieve tubes.
The smooth-walled escort cells lack a concentration gradient between parenchyma and escort cells. This type of escort cell was only examined more closely in the weeping willow ( Salix babylonica ): Here there should be no active loading of the phloem. The only decisive factor for the transport seems to be the concentration gradient between the mesophyll and the transport phloem.
Phloem discharge
The phloem discharge takes place at the places of consumption. In this phloem area the escort cells are very small or completely absent. Due to the always high concentration gradient between sieve tube and parenchyma, the discharge is likely to be predominantly symplastic, but both transport routes were observed. In the case of potatoes , the stolons are unloaded apoplastically as they grow in length, and when the tuber begins to grow, there is a switch to symplastic unloading. In the root tips and in young leaves, the discharge should be purely symplastic.
Transport mechanisms
The transport phloem comprises most of the entire phloem. In the transport phloem, the cross-sectional area of the sieve tubes is significantly larger than that of the escort cells, corresponding to the predominant function of transport. In this area, H + -ATPases and sucrose transporters occur both in escort cells and in sieve tubes. The sieve tubes of the transport phloem are not absolutely tight tubes. They lose a not insignificant part of the sucrose transported (with Phaseolus vulgaris around six percent per centimeter of the stem axis), a large part of which is taken up again in the sieve tubes. The rest is used to supply the tissues in the trunk. In the transport phloem, the sieve tubes / escort cell complexes are likely to be largely symplastically isolated.
Of several transport mechanisms that have been discussed, two remain, but they are not mutually exclusive, but rather represent the extreme points of a transition series. However, the pressure flow theory is given greater importance.
The pressure flow theory, first formulated in detail by Ernst Münch in 1930, is largely accepted today. It states that the flow through the phloem is based on the difference in osmotic pressure between the ends of the phloem. The osmotic pressure causes water to flow into the collecting phloem and thus a hydrostatic pressure gradient. This drives a mass flow through the sieve tube according to the law of Hagen-Poiseuille . In the discharge phloem, together with the discharge of the sucrose, water flows out. This water is either used for growth (root tips, fruits) or transported back via the xylem. According to the pressure flow theory, sucrose is both a driving agent and a transported substance. The substances present in low concentrations are therefore passively transported in the mass flow. Mass flow was detected using thermoelectric measurements and a confocal laser scanning microscope . Münch's original theory has already been adapted several times, so the transport phloem is not impermeable, as Münch assumed.
The mass flow is the product of the volume flow (J v ), the cross-sectional area of the sieve tubes (A) and the concentration of the transported assimilates (C):
The volume flow is in turn the product of the hydraulic conductivity (L p ) of the phloem and the difference in hydrostatic pressure between the place of loading ( source ) and place of discharge ( sink ) (P source - P sink ):
The hydraulic conductance L p A is not considered to be transport-limiting, so the mass flow is determined by the hydrostatic pressure difference and the assimilate concentration. This means that phloem loading and unloading are crucial for transport.
The second theory is the volume flow theory, which was established by Walter Eschrich in 1972. It assumes that osmotic processes not only take place at the beginning and end of the phloem, but along the entire route. Sucrose can therefore be loaded and unloaded at any point in the phloem. This is supported by the presence of sucrose transporters in the sieve tubes of the transport phloem of at least some species. The subsequent subsequent flow of water leads to local volume increases in the phloem and thus to a movement of the sucrose molecules away from this point. However, the water molecules do not migrate in total, so there is no mass flow of water plus sucrose. The transport therefore takes place due to many small lateral osmotic gradients, not due to a large longitudinal pressure gradient as in the pressure flow theory.
evolution
The structures resembling the sieve elements in some macrophytic brown algae (such as Microcystis ) developed independently of the phloem of the land plants.
For terrestrial and vascular plants, however, due to the soft tissue, sufficient fossil finds are lacking in order to be able to understand the evolution of the sieve elements and the types of escort cells. There is very little information on phloem anatomy prior to the Carboniferous period . Thin-walled cells in Rhynia and Trimerophyton from the Devonian are at the location of the phloem and are therefore interpreted as such, and they also have slightly inclined end walls. In the carbon, the sieve elements of the vascular spore plants and the gymnosperms are already similar to today's forms, there are no known transitional forms between the two. Little is known about the phloem of fossil bedspreads.
A comparison between the individual groups of land plants reveals the following trends: The porosity of the transverse walls of the sieve elements is increased by enlarging the symplastic windows. The sieve elements get longer and develop thicker cell walls, possibly to withstand the higher turgor pressure . The organelles in the cytoplasm of the sieve elements are greatly reduced in order to reduce the resistance to the mass flow.
A comparison with the ontogeny of the phloem allows some conclusions to be drawn about the phylogeny: In the protophloem of the few species of flowering plants examined, the escort cells are missing, there are only sieve elements. This corresponds to the state of the ferns and mosses.
The evolution of the escort cell types in the Bedecktsamern is unclear. It is generally believed that apoplastic phloem load is an inferred trait. Symplastic loading occurs particularly in the basal groups of the bed covers, especially in tropical trees. Apoplastic loading occurs more frequently in herbaceous representatives. Apart from a general tendency towards a decrease in the number of plasmodesmata, no structure is discernible for the individual types; the individual types with few plasmodesmata are likely to have developed independently several times.
use
The fibers in the phloem of some species are used commercially for fiber production, the most important are flax ( Linum usitatissimum ) and hemp ( Cannabis sativa ).
The phloem sap of some plant species is used by humans. Some monocots produce large amounts of phloem sap after injury. This is used for the production of sugar and alcoholic beverages. These are mostly palm trees : in Asia the genera Arenga , Borassus , Caryota , Cocos , Corypha , Nypa and Phoenix , in Africa Elaeis and Raphia , in South America Copernicia , Jubaea and Mauritia . Today this sugar production is of little economic importance, but at the beginning of the 20th century, 500,000 tons of sugar were extracted from palm trees in India alone. The quantities obtained from a plant can be very large: Corypha elata delivers up to 45 liters per day or 2700 liters in just over four months. Phoenix delivers up to 19 liters per day.
In addition to palm trees, the agave genus is also used. Agaves have been tapped in Mexico since pre-Columbian times and the phloem sap, called aquamiel , fermented into pulque . Agave salmiana is mainly used for this. At the beginning of the Spanish colonization, pulque was distilled, today mezcal and tequila are obtained directly from the strains and not from tapped phloem juice. During a season that lasts three months per year, up to 200 liters of aquamiel can be obtained from one plant . The latex that is extracted from the rubber tree ( Hevea brasiliensis ) is also found in milk tubes in the tree's living phloem.
Research history
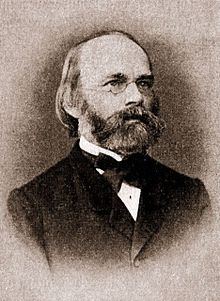
The term phloem in its current meaning goes back to Carl Wilhelm von Nägeli , who defined it together with its counterpart Xylem in 1858 in his work The growth of the trunk and the root in vascular plants and the arrangement of the vascular cords in the stem . Its name phloem is derived from the Greek phloios , which Theophrastus used in the sense of bark , bast , bark . The term sieve part was first used by Anton de Bary in 1877.
In the early 19th century, Heinrich Cotta , Augustin-Pyrame de Candolle, and Thomas Andrew Knight realized that carbohydrates were transported from the leaves into the stems and roots. Theodor Hartig discovered sieve tubes in 1837 . The division of transport into xylem and phloem and the substances transported in them were recognized in the mid-19th century. The question of the transport mechanism remained open for a long time and was hindered for a long time by Julius Sachs ' dogmatic view that transport was carried out exclusively through diffusion . At the beginning of the 20th century, Dixon and his students were able to clearly show that the transport rates are far beyond what is possible through diffusion. The decisive impetus for the elucidation of the transport mechanism were the work of Ernst Münch , who set up the pressure current theory ( Die Stoffbewegung in der Pflanzen , Gustav Fischer, Jena 1930).
With the advent of electron microscopy , the resistance to the pressure flow theory increased: the images showed that the screen pores were mostly covered by massive deposits of callose . The electro-osmotic theory was proposed as alternatives or the observed protein strands were viewed as the cause of the transport. The physiological findings, however, spoke more and more in favor of the pressure flow theory. The clogging of the sieve pores turned out to be an artifact in the mid-1970s. With careful preparation, the sieve plates were free. Visible evidence of the mass flow was also images of intact phloem in the 1990s using a confocal laser scanning microscope , which showed the transport of fluorescent dyes in the sieve tubes of Vicia faba . Another important method for phloem research is the extraction of the phloem sap with the help of aphid proboscis , whereby the proboscis is separated from the aphid after it has been pricked. Questions of phloem loading and unloading were essentially clarified with the help of the identification of the transport molecules involved and their cloning, as well as the manipulation of the normal loading process by incorporating foreign enzymes or by switching off individual enzymes.
supporting documents
Individual evidence
Most of the information in this article has been taken from the sources given under literature; the following sources are also cited:
- ↑ a b Gerhard Wagenitz : Dictionary of Botany. The terms in their historical context . 2nd expanded edition. Spectrum Academic Publishing House, Heidelberg / Berlin 2003, ISBN 3-8274-1398-2 , p. 241 f .
- ↑ a b c d e A.JE van Bel, M. Knoblauch: Sieve element and companion cell: the story of the comatose patient and the hyperactive nurse . Australian Journal of Plant Physiology, Volume 27, 2000, pp. 477-487. doi: 10.1071 / PP99172
- ↑ a b c d e f g h i j k l m van Bel: The phloem, a miracle of ingenuity , 2003.
- ↑ K. Ehlers, M. Knoblauch, AJE van Bel: Ultrastructural features of well-preserved and injured sieve elements: Minute clamps keep the phloem transport conduits free for mass flow . Protoplasma, Vol. 214, 2000, pp. 80-92. doi: 10.1007 / BF02524265
- ^ A b c Karl J. Oparka, Robert Turgeon: Sieve Element and Companion Cells - Traffic Control Centers of the Phloem . The Plant Cell, Vol. 11, April 1999, pp. 739-750. ISSN 1040-4651 (full text HTML)
- ↑ a b c Schopfer, Brennicke: Plant Physiology , p. 337f.
- ↑ Numerical values according to MV Rarthasarathy: Sieve-Element Structure . In: MH Zimmermann, JA Milburn (Ed.): Transport in Plants I. Phloem Transport . (= Encyclopedia of Plant Physiology New Series, Volume 1). Springer Berlin 1975, pp. 3-38. ISBN 3-540-07314-0
- ↑ Hiroaki Hayashi, Akari Fukuda, Nobuo Suzuki, Shu Fujimaki: Proteins in the sieve element-companion cell complexes: their detection, localization and possible functions . Australian Journal of Plant Physiology, Volume 27, 2000, pp. 489-496. doi: 10.1071 / PP99184
- ↑ Christina Walz, Patrick Giavalisco, Martina Schad, Melanie Juenger, Joachim Klose, Julia Kehr: Proteomics of curcurbit phloem exudate reveals a network of defense proteins . Phytochemistry, Vol. 65, 2004, pp. 1795-1804. doi: 10.1016 / j.phytochem.2004.04.006
- ↑ Dietrich Frohne, Uwe Jensen: Systematics of the plant kingdom with special consideration of chemical characteristics and plant drugs . 4th revised edition. Gustav Fischer, Stuttgart / Jena / New York 1992, ISBN 3-437-20486-6 , pp. 101 .
- ↑ a b c d Edith L. Taylor: Phloem Evolution: An Appraisal Based on the Fossil Record . In: H.-D. Behnke, RD Sjolund: Sieve Elements. Comparative Structure, Induction and Development . Springer, Berlin, Heidelberg 1990, pp. 285-298. ISBN 3-540-50783-3
- ↑ a b c d e Robert Turgeon, Richard Medville, Kevin C. Nixon: The Evolution of minor vein phloem and phloem loading . American Journal of Botany, Volume 88, 2001, pp. 1331-1339. ISSN 0002-9122 (abstract and full text)
- ^ A b c d Hubert Ziegler : Nature of Transported Substances . In: MH Zimmermann, JA Milburn (Ed.): Transport in Plants I. Phloem Transport . (= Encyclopedia of Plant Physiology New Series, Volume 1). Springer Berlin 1975, pp. 59-100. ISBN 3-540-07314-0
- ↑ Hans-Walter Heldt , Fiona Heldt : Plant biochemistry . Spektrum Akademischer Verlag, Heidelberg 1996, ISBN 3-8274-0103-8 , p. 263 .
- ^ Robert Turgeon: Plasmodesmata and solute exchange in the phloem . Australian Journal of Plant Physiology, Volume 27, 2000, pp. 521-529. doi: 10.1071 / PP99163
- ^ Horst Marschner : Mineral Nutrition of Higher Plants . Second edition, Academic Press, London 1995, pp. 92-115. ISBN 0-12-473543-6
- ↑ Van Bel: The phloem: a miracle of ingenuity , 2003; Frank Lichtner: Phloem mobility of crop protection products . Australian Journal of Plant Physiology, Vol. 27, 2000, pp. 609-617. doi: 10.1071 / PP99185
- ^ A b Robert Turgeon, Richard Medville: Phloem Loading. A Reevaluation of the Relationship between Plasmodesmatal Frequencies and Loading Strategies . Plant Physiology, Vol. 136, 2004, pp. 3795-3803. doi: 10.1104 / pp.104.042036
- ↑ Robert Turgeon, Richard Medville: The absence of phloem loading in willow leaves . Proceedings of the National Acadademy of Sciences USA, Volume 95, pp. 12055-12060, September 1998. ISSN 0027-8424 (abstract and full text)
- ↑ Schopfer, Brennicke: Plant Physiology , p. 342f.
- ↑ cf. P. Sitte, EW Weiler, JW Kadereit, A. Bresinsky, C. Körner: Strasburger - textbook of botany for universities . 35th edition, Spektrum Akademischer Verlag, Heidelberg 2002, pp. 312f. ISBN 3-8274-1010-X ; van Bel: The phloem, a miracle of ingenuity , 2003; Hans Werner Heldt: Plant Biochemistry . Spektrum Akademischer Verlag, Heidelberg 1996, p. 339. ISBN 3-8274-0103-8
- ↑ Schopfer, Brennicke: Plant Physiology , p. 343f.
- ↑ a b c S. Lalonde, M. Tegeder, M. Throne-Holst, WB Frommer, JW Patrick: Phloem loading and unloading of sugars and amino acids . Plant, Cell and Environment, Volume 26, 2003, pp. 37-56. doi: 10.1046 / j.1365-3040.2003.00847.x
- ↑ for a more detailed consideration cf. also: MT Tyree, J. Dainty: Theoretical Considerations . In: MH Zimmermann, JA Milburn (Ed.): Transport in Plants I. Phloem Transport . (Encyclopedia of Plant Physiology New Series, Volume 1) Springer Berlin 1975, pp. 367-392. ISBN 3-540-07314-0
- ↑ a b J. Van Die, PML Tammes: Phloem Exudation from Monocotyledonous Axes . In: MH Zimmermann, JA Milburn (Ed.): Transport in Plants I. Phloem Transport . (= Encyclopedia of Plant Physiology New Series, Volume 1). Springer Berlin 1975, pp. 196–222. ISBN 3-540-07314-0
- ^ MH Zimmermann, JA Milburn: Introduction . In: the same (ed.): Transport in Plants I. Phloem Transport . (= Encyclopedia of Plant Physiology New Series, Volume 1). Springer Berlin 1975, pp. VII-IX. ISBN 3-540-07314-0
- ↑ Overview at: AJ Peel: Investigations with Aphid Stylets into the Physiology of the Sieve Tube . In: MH Zimmermann, JA Milburn (Ed.): Transport in Plants I. Phloem Transport . (= Encyclopedia of Plant Physiology New Series, Volume 1). Springer Berlin 1975, pp. 171-195. ISBN 3-540-07314-0
literature
- Aart JE van Bel: The phloem, a miracle of ingenuity . In: Plant, Cell and Environment. Volume 26, 2003, pp. 125-149, doi: 10.1046 / j.1365-3040.2003.00963.x .
- Katherine Esau : Anatomy of Seed Plants . John Wiley, 1960, pp. 122-141. (Cell types)
- Peter Schopfer, Axel Brennicke: Plant Physiology . Founded by Hans Mohr . 6th edition. Elsevier, Spektrum, Munich / Heidelberg 2006, ISBN 3-8274-1561-6 , pp. 333–346 ( limited preview in Google Book search).