magnetism
The magnetism is a physical phenomenon that, among other things as a force effect between the magnet magnetic or magnetizable objects and the moving electric charges is expressed. It can be described by a field ( magnetic field ) that is generated by these objects on the one hand and acts on them on the other.
On the one hand, magnetic fields arise with every movement of electrical charges. This is the basis of electromagnets and, because of the law of induction, also of inductive electronic components . On the other hand, the magnetic moment of elementary particles exists as a result of their spin , which leads to permanent magnets and other magnetic properties of solids, but also liquids and gases.
Magnetism is a branch of electromagnetism . The underlying force is called electromagnetic interaction .
Magnetic fields and field lines
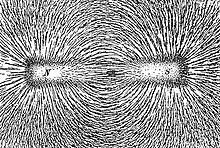
To describe the phenomena of magnetism, the concept of the magnetic field was introduced . Magnetic fields can be caused by
- magnetic materials, such as a permanent magnet ,
- electrical currents , e.g. B. a current-carrying coil or
- change in an electric field over time .
Magnetic field lines illustrate the direction and sense of direction of the magnetic field or the magnetic flux at every point of the field . This direction is determined by how the north pole of a test magnet would align. The strength of the magnetic field is proportional to the torque that this test magnet would experience if it were deflected from this direction by a certain angle. The distance between the field lines shows the strength of the magnetic field: the closer the field lines, the stronger the field.
In contrast to electrostatics, there are no charges in magnetostatics - true magnetic monopoles are conceivable, but all experimental facts speak against their existence. Thus the magnetic field is source-free . Magnetic field lines therefore have no beginning and no end.
The course of magnetic field lines can be made visible by aligning iron filings or a compass needle ; For three-dimensional demonstrations, the iron filings can be suspended in silicone oil , for example .
Hall probes are electronic sensors based on the Hall effect that can measure the strength and often the direction of magnetic fields.
North and South Pole
In the absence of other forces, a bar magnet on the earth's surface aligns itself in such a way that one of its ends points north, towards the Arctic magnetic pole , and the other towards the Antarctic magnetic pole. The north-facing end is called the north pole of the magnet. It was defined by definition that the field lines exit the magnet at the north pole of a magnet and enter it at its south pole. Therefore, in the case of electromagnets or permanent magnets, areas from which the field lines emerge are generally referred to as the north pole and the areas into which they enter as the south pole .
Since the north pole of the magnet is attracted to the arctic magnetic pole, the arctic magnetic pole is a south magnetic pole. The same applies vice versa for the south pole of the magnet and the Antarctic magnetic pole.
Magnetic force effects
The magnetic field exerts what is known as the Lorentz force on moving electrical charges . It is proportional to the speed , acts perpendicular to the field lines of the magnetic field and perpendicular to the direction of movement of the charge. It is the basis of electric motors and generators as well as the deflection of moving charged particles (e.g. with deflection coils ). No energy is exchanged with a static magnetic field.
The magnetic field also exerts forces on magnets and magnetizable bodies ( ferrimagnetism of certain non-metallic solids, so-called ferrites , and ferromagnetism of metals such as iron). Magnets and stretched specimens made of magnetizable materials are always aligned along the field lines or anti-parallel to them, i.e. the magnetic south pole of a test magnet is aligned along the field lines to the north pole of the generating field. This effect is used, for example, in the magnetic compass , in which the compass needle, a magnetic dipole, aligns itself with the earth's magnetic field . In addition, in inhomogeneous fields magnetizable bodies are drawn in the direction of increasing field strength, see gradient , applications are electromagnets and the reluctance motor . This also applies to magnets, which can freely align. In contrast, magnets oriented in the opposite direction are repelled.
The reason for these observations is that a lower energy state is assumed - the forces and torques always work in such a way that the total energy of the field decreases when the bodies follow them, whereby the binding energy is released as mechanical work . Conversely, work is done on the bodies when they are moved against the forces. The work lowers or increases the energy of the field. If coils are involved, electrical energy can also be supplied or removed.
Sizes and units

The strength of a magnetic field can be expressed by two different physical quantities, the magnetic field strength (unit: A / m, i.e. ampere per meter; in the CGS system of units there is the name Oersted for the corresponding unit) and the magnetic flux density (the so-called. "Magnetic Induction") ( Tesla unit ). In a vacuum, these differ only in one constant factor, the magnetic field constant :
- .
In matter, e.g. B. in permanent magnets, the relationship is more complicated: In this case is continuous across a transverse gap , across a longitudinal gap . Measurements with a magnetic field probe in the transverse and longitudinal gap can be significantly different. The size is always source-free, while the same applies to not (see below). While the magnetic field strength is advantageous for calculations with electrical currents or with ferromagnetic or ferrimagnetic material, the magnetic flux density is used to calculate induced voltages or the Lorentz force. The two field quantities are linked to one another via the material equations of electrodynamics , which in the simplest case can be expressed via a factor, the magnetic permeability ; in the general case, where the vector is referred to as the magnetization of the material applies instead . Freedom from sources and freedom from eddies - the latter only in the case of the absence of electrical currents - are expressed mathematically by the equations or . Here and are the differential operators for the divergence or the rotation , i.e. for the source or vortex density of a field.
Examples of magnetic fields
The intergalactic magnetic field, expressed as the magnetic flux density in the unit Tesla (T), is estimated to be less than 0.1 nT (10 −10 T), that of the Milky Way to 30 nT. The Earth's magnetic field has a strength of 40 µT on the surface, which corresponds to 0.4 Gauss in the Gaussian system of units . The magnetic flux density of the sunspots is less than 1 mT. The saturation magnetization of iron is approximately two Tesla.
On the surface of neutron stars , such as For example, pulsars , on the other hand, typically have flux densities of 10 8 Tesla, with magnetars , a special type of neutron stars, even 10 11 Tesla.
The currently (2009) weakest magnetic field on earth with 1 nT can be found in a specially shielded cubic building of the Physikalisch-Technische Bundesanstalt in Berlin . The purpose of the cube is to measure weak brain waves and heart signals in people.
At the National High Magnetic Field Laboratory in Tallahassee (Florida) the currently strongest temporally constant magnetic field on earth is generated at 45T. Even higher magnetic fields can be achieved with electromagnets in short pulses. The world record for non-destructive magnet construction is currently (2012) held by the National High Magnetic Field Laboratory in Los Alamos, USA with 100.75 T.
With intense laser radiation , flux densities of up to 34 kilotesla can be generated - but only for around 10 ps .
High magnetic fields of, for example, 2800 T can be generated with current pulses if it is accepted that the coil will be destroyed (or self-destruct). An additional increase in the flux density can be achieved with simultaneous compression of the coil or the field by means of explosive charges; see also flow compression generator .
Magnetic energy
Every magnetic field contains energy . The energy density at any point in a magnetic field in a vacuum is given by
- .
This is the amount of magnetic field strength, the amount of magnetic flux density at the given point and the magnetic field constant or permeability of the vacuum .
The total energy of the magnetic field of a current-carrying coil is
- .
Here stands for the inductance of the coil and for the current strength .
Electromagnetism
Electromagnetism is understood to mean the diverse relationships between magnetism and purely electrical phenomena. Magnetic fields that are created by electrical currents can be interpreted by the special theory of relativity as a result of the electrostatic forces between the charges. The explanation is based on the fact that an electric current represents a relative movement of oppositely charged particles whose charge densities are influenced differently by the Lorentz contraction . Charged elementary particles, which have their own angular momentum (spin) , also have a magnetic moment and are therefore a. responsible for ferromagnetism . This is interpreted by the relativistic quantum mechanics .
Even with the effect of a magnetic field on a moving charged particle, there is an effect that can only be explained by quantum mechanics (see Aharonov-Bohm effect ). A spatially limited magnetic field influences the dynamics of a charged particle, even if it only moves in an area with a vanishing magnetic field.
Movements of charge carriers cause changes in the electrostatic and magnetic fields of their surroundings. Since these changes affect each other and spread in space, one speaks of electromagnetic waves . Light (whether visible or invisible) and radio are the most well-known forms of this phenomenon, but this form of electromagnetism is also used in metal processing ( induction furnaces ) and for heating even non-conductive substances ( microwave ovens ).
For an in-depth presentation and classification of electromagnetism see the article Electromagnetic Interaction .
Direction rules
The amount and sign of the moved charges as well as the amount and direction of their speed determine the strength and direction of the magnetic forces and the magnetic fields on which they are based. a. is possible with Hall probes .
For the relationship between the direction of the current and the direction of the magnetic forces or the magnetic fields on which they are based, a number of differently named rules and memory aids are in circulation, which initially differ according to whether they are from the "conventional" or "technical" direction of the current (against the electron flow) or the direction of the electron flow (colloquially also called "physical" flow direction) is assumed. If the former is the case, one speaks of right-hand or right-fist rules, otherwise of left-hand or left-fist rules, with the former traditionally predominating.
The next distinction is whether one uses the thumb and the index and middle fingers spread apart at right angles to the previous one for the rule in question, or whether all fingers apart from the thumb are imagined to be closed in a fist.
While the first-mentioned rules are actually left or right- hand rules - also known as the three-finger rule , UVW rule or IBF or FBI rule - the direction of the Lorentz force on a moving charge carrier in a (specified ) show the external magnetic field, the rules mentioned in the second place - left or right fist rule, rule of containment, popularly also called screw rule or corkscrew rule - primarily serve to indicate the direction of the magnetic field that the moving charge carrier through its Movement itself generated, be it free-flying or in a straight or ring-shaped electrical conductor, e.g. B. a coil.
Examples: Right- hand rule or (right-handed) UVW rule
- Shows the splayed thumb of the right hand in the conventional or direction of current of a current-carrying ( U AUSE) conductor and the splayed at right angles to the thumb finger in the direction of the external magnetic field ( V determination), the right angle splayed to both middle fingers in direction of the charge carriers in the conductor (and himself) Lorentz force acting ( W MPACT).
- And vice versa: If an electrical conductor (by mechanical agitation U AUSE) towards the splayed thumb of the right hand in an external magnetic field ( V determination) brought whose direction is that of the splayed at right angles to the thumb index finger, then in the conductor a current flow ( W MPACT) in the technical direction induced into which the thumb to and shows forefinger vertically splayed middle finger.
Examples: right- fist rule or corkscrew rule
- If a current-carrying conductor is grasped with the right hand in such a way that the splayed thumb points in the direction of the conventional or technical current direction , the curved fingers point in the direction of the resulting magnetic field.
- For a circular current (e.g. that of a coil) the following applies accordingly: If the coil is held in the right hand so that the fingers are curved in the direction of the technical current direction , the splayed thumb points in the direction of the magnetic north pole.
Explanation of the phenomenon
Magnetism (similar to superconductivity ) involves specific quantum mechanical effects that are not easy to represent.
A successful model was developed in 1927 with the Heitler-London theory of the formation of hydrogen molecules, although this theory initially seemed to have nothing to do with “magnetism”. According to this theory, σ molecular orbitals arise , i.e. H. From the two atomic hydrogen functions u i (...) an orbital σ-molecular state is formed:
The last product results from the first because of the quantum mechanical principle of the indistinguishability of identical particles . It means: The first electron r 1 can not only be in the first atomic nucleus , but just as well in an atomic hydrogen orbital in the second atomic nucleus, while the second electron is in the first atomic nucleus. This results in the “ exchange interaction ”, which plays a fundamental role in the creation of magnetism and is stronger by factors of 100 to 1000 than the phenomenological terms described by electrodynamics .
For the spin function χ ( s 1 , s 2 ), which is responsible for magnetism, the complementary behavior then applies because of the Pauli principle
d. This means that not only must u i be replaced by α and β (the former means " spin up ", the latter " spin down "), but also + by - and z. B. r 1 by the two discrete values of s 1 , namely by ± ½. The following applies:
- and
- .
So, d. H. with the minus sign in (1b) results in a singlet spin function. That means: the spins are anti-parallel ; for solids this means antiferromagnetism and for diatomic molecules diamagnetism .
The tendency to molecular bonding , according to the above location function, that automatically results in the already mentioned Singulettsymmetrie in spin state due to the Pauli principle; whereas the Coulomb repulsion of the two electrons would lead to a singlet position function and complementarily to a triplet spin function, i.e. that is, "the spins would now be parallel ".
The latter effect predominates with iron , cobalt and nickel ; these metals are ferromagnetic . In the case of diatomic molecules, it also predominates in the case of oxygen , which, in contrast to the other diatomic molecules, is not diamagnetic but paramagnetic . The first-mentioned effect, however, predominates with the other metals such as sodium , potassium , magnesium or strontium , which are non-magnetic , or with manganese , which is antiferromagnetic .
From the Heitler-London model, the basic Heisenberg model of magnetism emerged through generalization (Heisenberg 1928).
The explanation of the phenomenon is ultimately based on all the subtleties of quantum mechanics, including its mathematical structure , in particular on the spin described there and the Pauli principle, while electrodynamics rather describes phenomenology.
Magnetism of matter
Magnetic moment of elementary particles
All fundamental charged elementary particles have a characteristic magnetic moment . It is linked to their spin via the gyromagnetic relationship .
Elementary particles | designation | |
---|---|---|
electron | ||
Muon | ||
proton | ||
neutron |
Magnetic moment of atoms
The magnetic moment of an atom is made up of the contribution of the electron shell (shell moment) and the generally much weaker core contribution (nuclear moment).
The orbital moment, which is linked to the orbital angular momentum of the electrons , and the spin moment determined by the electron spin contribute to the shell moment. The sum of the magnetic moments of the electrons of a doubly occupied atomic orbital is zero, so that atoms that do not have half occupied orbitals do not have a permanent shell moment.
The nuclear moment is very small, but it can not only be detected ( Zeeman effect , Stern-Gerlach experiment ), but also used in practice (e.g. NMR spectroscopy ( Nuclear Magnetic Resonance ), MR Tomography ).
Magnetism of solids
Magnetism of solids is a cooperative phenomenon . The macroscopic magnetization is made up of the contributions of the individual building blocks ( atoms , ions , quasi-free electrons ) that make up the solid . With many materials, the individual building blocks already have a magnetic moment. However, even of the materials whose building blocks carry such magnetic moments, only a few show a macroscopic magnetization. As a rule, namely, the various moments add up to the total moment zero. Only if this does not happen, i.e. if their contributions do not cancel each other out, is a macroscopic magnetization the result.
Five types of magnetism can occur in solids. The naming of the magnetic as well as the electric field is done analogously by using the appropriate prefix:
magnetism | Explanation | illustration |
---|---|---|
Diamagnetism | If a substance is brought into a magnetic field, this induces a current in the electron shells of the atoms, the magnetic field of which, according to Lenz's rule , is opposite to the external one. Diamagnetism leads to a weakening of the magnetic field in the substance. In materials whose atoms, ions, or molecules do not have unpaired electrons, diamagnetism is the only form of magnetism. | |
Paramagnetism | If the atoms, ions or molecules of a material have a magnetic moment, they align themselves parallel to the external magnetic field. This increases the magnetic field in the material. In the case of an ideal paramagnet, the individual magnetic moments are isolated from one another. This is why the internal magnetic field collapses after the external magnetic field is removed due to the thermal movement of the particles. Correspondingly, the paramagnetism decreases with increasing temperature. |
![]() |
Ferromagnetism | In ferromagnetism, the magnetic moments of individual particles are not independent of one another, but spontaneously align themselves in parallel. The coupling of the magnetic moments does not extend over the entire material, but is limited to small areas, the Weiss areas. Typical length scales are ten nanometers to a few micrometers. The alignment of the Weiss districts is statistically distributed so that the entire body appears non-magnetic. An external magnetic field can be used to align the districts in the same way. This rectification is retained even after the external field has been removed, so that permanent magnetization is obtained. The magnetization can be destroyed by heating above the ferromagnetic Curie temperature . |
![]() |
Ferrimagnetism | In ferrimagnetism, too, the magnetic moments of individual particles are not independent of one another. But there are two types of magnetic centers. The spin moments of similar centers are aligned parallel and those of different ones are anti-parallel. This leads to a partial extinction of the magnetic moments. Otherwise they are similar to ferromagnets. |
![]() |
Antiferromagnetism | Even with antiferromagnetism, the magnetic moments of individual particles are not independent of one another, but rather align themselves spontaneously in an anti-parallel manner. Therefore the ideal antiferromagnet shows no magnetic behavior to the outside. As the temperature rises, the heat movement disrupts the arrangement, so that the antiferromagnet increasingly behaves like a ferrimagnet. When heated above the Néel temperature , the antiferromagnet only behaves paramagnetically (compare Curie temperature for ferromagnets). |
![]() |
In addition, there are forms of magnetism that are characterized by the non-magnetic or non-linear behavior of the five types of magnetism:
- Metamagnetism
- Metamagnetic materials (e.g. iron (II) chloride ) show vanishingly small magnetizations with very small external magnetic fields (antiferromagnetic), with increasing field strength the magnetization increases disproportionately strong and steadily and approaches a saturation value. This behavior can be described in such a way that the crystal behaves antiferromagnetic for small fields and ferromagnetic for strong fields.
"Amagnetism"
With Amagnetismus, non-magnetic, non-magnetic or non-magnetic is meant "not ferromagnetic" usually, for example, as a property of austenitic steel, in contrast to ordinary steel . There are no substances on which a magnetic field has no effect at all. In the case of very high magnetic field strengths, even with "amagnetic" materials, there may be attraction or, to a lesser extent, repulsion effects, albeit much weaker than with ferromagnetic materials. The term amagnetic is not used uniformly.
Methods of measuring magnetism in solids
Various macroscopic and atomic-microscopic methods are used to investigate the different types of magnetism and their temperature dependence. One of the most sensitive macroscopic methods is based on the Josephson effect and is used in the SQUID , which in materials research is usually combined with a regulated cryostat . The Hall effect is also a macroscopic method and is also used in many simple technical applications, e.g. B. in car engines .
On the atomic scale, atomic nuclei are used using hyperfine interaction to measure the respective nucleus size of the magnetic field with atomic nuclei in the crystal lattice at the location. Known methods are Mössbauer spectroscopy , disturbed gamma-gamma angle correlation and NMR .
Magnetism in Biology
Because every nerve activity also consists of electrical currents, our nerve tissue and especially our brain constantly produce magnetic fields that can be received with sensitive detectors.
Alternating magnetic fields can induce electrical currents in the tissue and thus have a (weak) influence on the nervous system. The motor cortex can be stimulated with the help of transcranial magnetic stimulation (TMS) in such a way that involuntary muscle contractions occur. The nerves in the muscles themselves can also be stimulated in this way.
So-called magnetophosphenes (optical sensory perceptions) occur in correspondingly strong fields (for example in a magnetic resonance tomograph ) . Furthermore, it has long been known that alternating magnetic fields can influence the secretion of hormones (for example melatonin ).
Many birds, sea turtles and far-away fish have a magnetic sense and can use the earth's magnetic field to orient themselves.
The doctor Franz Anton Mesmer developed a theory, which was tested and rejected by the French Academy of Sciences in 1784 , according to which a fluid that Mesmer called magnetism animalis could be transmitted from person to person and in hypnosis and certain healing processes ( Mesmer deletions ) should play a role. In addition to animal magnetism, which was popular at the time, the doctor and magnetizer Louis Joseph Jules Charpignon in Orléans also dealt with magnetism in a more general sense around 1845.
See also: Magnetotaxis , Magnetospirillum gryphiswaldense , Magnetospirillum magnetotacticum , Magnetosom
Dangers to people
Effects or dangers of direct magnetic fields on humans are not known. The pulsed fields in magnetic resonance imaging are also generally harmless. On the other hand, there are dangers in strong fields in the following cases:
- Force effect from ferromagnetic or ferromagnetic parts on or in the body
- Flying ferromagnetic or ferromagnetic parts
For this reason, safety rules apply in magnetic field laboratories and on magnetic resonance tomographs, which ensure that no ferromagnetic parts get into the vicinity. The following damage is still relevant:
- Disruption or failure of pacemakers
- Disturbance of non-“amagnetic” clocks and other mechanical devices
General dangers
Pulsed fields can influence or destroy all electronic and electrical devices through electromagnetic induction , cf. also electromagnetic pulse .
Irregularities in the flow of particles from the sun ( solar wind ) lead to so-called magnetic storms on earth which, through induction, can endanger telephone and landline lines, cable systems and also metal pipelines.
Magnetic fields can erase recordings on magnetic data carriers such as audio tape , video tape or hard drive .
If a magnetic field suddenly collapses as a result of an incident - line interruption in conventional electromagnets or quenching in superconducting magnets - very high electrical voltage pulses can result from induction. If these lead to current flow, the magnetic fields generated in turn can e.g. B. Forcibly pull objects into the magnet. Therefore, experiment setups in the immediate vicinity of the magnet must not contain any closed conductor loops - for example in any kind of racks; this is achieved by inserting insulating spacers.
The two ring-shaped permanent magnets from the magnetron of a microwave oven attract each other so strongly that you can painfully pinch and injure a fine fold of the skin between them.
Magnetism in everyday language and everyday life
Misunderstandings often arise from confusing the terms “magnetic” (in the sense of ferromagnetic ), “magnetized” and “magnetizable”.
In colloquial language, magnetism is almost exclusively understood to be ferromagnetism, because this is common and familiar in everyday life: holding magnets on a metal sheet, the operation of a compass, etc. The other types of magnetism (diamagnetism, paramagnetism, etc.), on the other hand, are in everyday life inconspicuous. With "magnetic" is usually meant "ferromagnetic". Most people correctly associate the term magnetism very strongly with the materials iron and steel. What is less well known is that nickel and cobalt are also ferromagnetic.
Wrong ideas about magnetizability are common and can also be found in some books and other sources. For example, an object made of simple steel is ferromagnetic and therefore magnetizable, but only magnetically “soft”, which means that it loses its magnetization again very quickly. A temporary compass needle cannot be made from just any steel wire by brushing it over with a permanent magnet; a magnetically soft steel wire is not suitable. If you touch a magnetically soft steel wire with a permanent magnet, it will be attracted, but not permanently magnetized. A magnetically “hard” steel needle, on the other hand, can be magnetized permanently, which means that it only loses its magnetization over a long period of time and could therefore function as a makeshift compass.
You can easily check whether an object is “magnetic” (in the sense of ferromagnetic) by touching it with a permanent magnet. If you feel a force, then the object is ferromagnetic. Whether an object is "magnetized" - that is, is itself a permanent magnet - can be checked using a very light piece of non-magnetized steel (e.g. a staple or paper clip): If the staple gets stuck on the object, then it is he magnetizes.
Magnetization of tools, for example, may be desirable in practice (e.g. some screwdrivers are intentionally magnetized to make handling of small iron screws easier). The magnetization can, however, also be undesirable because it constantly causes small iron filings or the like. stick to the device.
Apple notebooks sometimes have a magnetically coupling power supply socket. This MagSafe connection is released in most cases if the cable is accidentally pulled and can thus prevent the device from falling from the table to the floor. The flat plug has a ferromagnetic frame that is attracted by the magnet in the slightly recessed area of the socket. If the device is put into a backpack without a cover, which is also used to transport hand tools, the socket can attract iron and rust particles and become clogged. These particles can be pulled out using strong adhesive tape.
shielding
The shielding of electrotechnical devices, facilities and rooms is used to keep electrical and / or magnetic fields away from them or, conversely, to protect the environment from the fields emanating from the facility. Magnetic shields are used e.g. B. used in CRT monitors and oscilloscopes with cathode ray tubes , since magnetic interference sources can cause picture interference. Permanent magnets of loudspeakers in television sets with picture tubes are often magnetically shielded.
Soft magnetic materials are used to shield static magnetic fields and low frequency magnetic fields, i.e. H. ferromagnetic materials of high permeability and low remanence. Magnetic shielding also has an electrical shielding effect if it is sufficiently conductive. High-frequency, electromagnetic alternating fields ( electromagnetic waves ) can only be completely shielded with electrically conductive, all-round closed envelopes of sufficient thickness. Gaps or openings reduce the shielding attenuation and make it impossible if their largest dimension reaches or exceeds the order of magnitude of the wavelength to be shielded.
See also
- Magnetic tension or flux (physical quantity)
- Elementary magnet (model presentation to explain certain properties of a magnetizable substance)
- Magnetocaloric effect (temperature change when the magnetic field changes)
- Bohr-van-Leeuwen theorem (magnetism is a purely quantum mechanical effect in solids)
Web links
- Magnetism in great detail in Welt der Physik
Remarks
- ↑ The freedom from sources always applies - strictly speaking - to the magnetic flux density , but not to the magnetic field strength , see below.
- ↑ Expressed mathematically: Magnetic fields are always vortex fields , while electrostatic fields are always gradient fields (each vector field can be split into a gradient part (eddy-free part) and a vortex part (source-free part)).
- ^ PJ Morrison: Magnetic Field lines, Hamiltonian Dynamics, and Nontwist Systems. Physics of Plasmas, Vol. 7 No. 6, June2000, pp. 2279-2289 . The article shows that in three-dimensional arrangements without particular symmetry, closed field lines even occur comparatively rarely. This also suggests the following paradox: A bar magnet made of flexible material is being considered, into which someone has knotted a simple loop before magnetizing. Its field lines run inside from the S pole to the N pole along the node and close outside the magnet in free space. Now the magnet is slowly untied. Its field lines are always closed. Since a knot in a closed loop cannot disappear for topological reasons, the field lines surrounding the stretched bar magnet would now have to contain a knot, which is not possible. The paradox can be resolved by giving up the idea of closed field lines.
- ↑ Magnetic field measurement on the human heart with small sensors at room temperature (PTB press release of December 11, 2009).
- ↑ Magnetic field researchers target Hundred-Tesla goal ( Memento from September 28, 2012 in the Internet Archive ), press release of the Los Alamos National Laboratory from March 22, 2012.
- ↑ Presentation of the TU Dresden (PDF file, 8.2 MB, accessed on June 30, 2011)
- ↑ According to the Pauli principle, the complementarity consists in the fact that with diatomic molecules a symmetrical position function (sign +) has to be multiplied by an antisymmetrical spin function (sign -), and vice versa.
- ↑ For the theory of magnetism see z. BU Krey, A. Owen, Basic Theoretical Physics - A Concise Overview , Springer, Berlin 2007.
- ↑ CODATA Recommended Values. National Institute of Standards and Technology, accessed July 21, 2019 . Magnetic moment of the electron.
- ↑ CODATA Recommended Values. National Institute of Standards and Technology, accessed July 21, 2019 . Magnetic moment of the muon.
- ↑ CODATA Recommended Values. National Institute of Standards and Technology, accessed July 21, 2019 . Magnetic moment of the proton.
- ↑ CODATA Recommended Values. National Institute of Standards and Technology, accessed July 21, 2019 . Magnetic moment of the neutron.
- ^ AF Holleman , E. Wiberg , N. Wiberg : Textbook of Inorganic Chemistry . 101st edition. Walter de Gruyter, Berlin 1995, ISBN 3-11-012641-9 , pp. 1300-1310.
- ↑ Gerhard Fasching: Materials for electrical engineering . 3. Edition. Springer, Vienna 1994, p. 384.
- ↑ Louis Jules Charpignon: physiology, medicine et métaphysique you magnetisme. Brussels 1851.
- ↑ Sabine Kleine: The rapport between animal magnetism and hypnotism. In: Würzburg medical history reports. Volume 13, 1995, pp. 299-330; here: p. 314 f.