District heating
As district heating or district heating , heat supply for supplying buildings with space heating and hot water , respectively. The thermal energy is transported in a thermally insulated pipe system that is mostly buried in the ground, although overhead lines are sometimes used. District heating primarily supplies residential buildings with space heating and hot water by channeling the heat from the generator or collection point to the consumers. Local heating is also used for local development of individual buildings, parts of buildings or small residential areas with their own heat generation . As of 2013 there were around 80,000 district heating systems worldwide, around 6,000 of them in Europe.
Thermal power stations and block -type thermal power stations are often used as heat suppliers in district heating networks using fossil fuels , biomass or waste . These are operated in cogeneration and can thus achieve fuel savings compared to separate electricity and heat generation. In addition, waste heat from industrial processes, geothermal energy , solar thermal energy ( solar district heating ) and environmental heat made usable by means of large heat pumps is fed into district heating systems. District heating technologies are constantly being developed. So z. In Europe, for example, there is a trend towards more sustainable district heating systems with a higher proportion of renewable energy sources , less use of fossil fuels, more energy-efficient systems and lower emissions of greenhouse gases and air pollutants . District heating systems operated exclusively with renewable energies are, however, not very widespread as of 2017.
history
From archaeological sites from Roman times we know that hot thermal water was not only used for bathing purposes on site, but was also transported by pipes in pools and buildings for underfloor heating. Predecessors of district heating systems have existed for more than 2000 years. The first "real" district heating system was built in 1334 in the French town of Chaudes-Aigues . There, hot thermal water was fed into the village from a spring to supply several houses.
The idea of using district heating on a larger scale and commercially emerged towards the end of the 19th century. By reducing the number of fireplaces in the city centers, the risk of fires has been reduced and pollution from coal and ash has been halted. The first modern district heating systems were built in Lockport and New York in the 1870s and 1880s . In the 1920s, the first European district heating networks were installed in Germany; at the same time, there was a transition from inefficient steam systems to hot water. The Soviet Union began building district heating systems in the 1930s, and so did the People's Republic of China in the 1950s. In the 1970s, with the oil crises, interest in district heating increased sharply, whereupon some countries, especially the Scandinavian countries, built new third-generation district heating networks. As a result of the energy transition towards a sustainable energy supply, a fourth generation is now being researched and established in practice.
Technical process
A district heating system consists of various main components that together form the overall system. These include heat generation plants (often in cogeneration powered heating plants ), most of them with hot water powered district heating system, including pumping stations and household connections and the transfer stations, which emit the heat to the building heating system.
Because of the unavoidable heat loss over long distances, even with very good thermal insulation, and the high investment costs for the pipe system, district heating is often only suitable for dense buildings. District heating networks usually have star-shaped distribution structures with maximum line lengths in the range of a few 10 km. The longest district heating pipeline in Austria, with a length of 31 km, is located between the Dürnrohr power plant and the Lower Austrian capital St. Pölten . In Germany, the word “district heating unworthy” is used for normal suburban development when a district heating supply appears unprofitable. In Scandinavia, where district heating plays a central role in the heating market, district heating networks are also operated in the villa suburbs. For example, district heating in Denmark covers around half of Denmark's heating requirements (as of 2018). A further expansion to up to 65–70% is considered possible, while heat pump heating was seen as a cheaper option for sparsely populated areas.
Heat sources

District heating networks have the property of being able to use a large number of different heat sources very flexibly, which can be both centralized and decentralized. District heating is usually generated in large power plants with combined heat and power (CHP), smaller combined heat and power plants , in waste incineration plants or district heating plants . The various forms of coal , natural gas , biogas , oil, wood and wood products, solar thermal energy and waste in various compositions and processing forms are used as fuel. In a few countries, e.g. B. in Switzerland, district heating is also extracted from nuclear power plants . In Iceland, but also in Central Europe, district heating is generated in geothermal power plants. As far as possible, the high-temperature waste heat from industrial operations, for example from refineries or steelworks, is used as a heat source. The most important energy sources are currently still fossil fuels such as natural gas and coal, which in 2012 provided around 70% of the thermal energy in the European district heating networks.
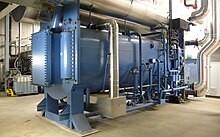
For a sustainable district heating system, however, renewable energies and the use of waste heat are the most desirable energy sources. As part of the energy transition and the associated conversion of the electricity and heat supply to renewable energies, additional heat sources are increasingly being used. So z. B. large heat pumps operated with wind or solar power that deliver thermal energy and at the same time help to smooth the fluctuating feed-in of these energy sources. This sector coupling between the electricity and heating sectors can play a key role in the energy system in the integration of variable renewable energies. At the same time, the use of heat pumps in district heating systems is considered to be one of the most promising ways to increase the energy efficiency of district heating networks and to achieve climate protection goals, not least because heat pumps are emission-free when using green electricity.
As heat sources for large heat pumps come u. a. Low-temperature waste heat from industry, supermarkets , waste water (e.g. from sewage treatment plants ), drinking, industrial and ground water, rivers, lakes and sea water are in question. There is great potential for large heat pumps in particular in the use of wastewater and surface water as a heat source, both of which also have the advantage that they usually occur in the vicinity of settlements and are available in the long term. A system based on sea water is e.g. B. has been used in Stockholm's district heating network since the 1970s, another example is the Drammen heat pump . Data centers are another powerful source of heat . Large data centers can have a permanent power consumption of 100 MW of electrical energy. The resulting waste heat can be used with large heat pumps and the thermal energy can then be fed into district heating networks. As of 2017 this will e.g. B. already practiced in data centers in Stockholm and Helsinki.
Short-term surpluses of green electricity can be converted into heat with electrode boilers and then fed into district heating networks or district heating stores. This technology is known as power-to-heat and is now spreading rapidly. Both electrode boilers and heat pumps are mature technologies that are available on the market. However, the application profiles differ significantly: While electrode boilers can be operated particularly flexibly, heat pumps are more suitable as a base load technology because they have high investment costs but low operating costs. Compared to heating rods and electrode boilers, heat pumps have a significantly higher energy efficiency , so that less electricity is required for the same heating energy. The electrical energy saved in this way is available for other purposes.
Be particularly advantageous for the integration of high shares of renewable energy at the same time very high overall efficiency is the combination of cogeneration and other in cogeneration considered fired power plants with heat pumps and heat storage. In such a system, heat pumps would cover the heat demand during periods of high electricity production from wind and / or solar energy and at the same time utilize any excess electricity, while the CHPs could remain switched off. With only low electricity production from renewable energies, however, the CHPs would provide both electricity and heat. By integrating heat storage systems into such a system, electricity and heat production can also be decoupled from one another, so that any losses due to heat from the CHPs that are temporarily not required are minimized.
It is also possible to build solar thermal systems on roofs or as an open-space system, which can feed directly into district heating networks as a supporting heat source or, in combination with seasonal storage tanks, cover the heating requirement all year round. This is known as solar district heating . As of 2017, there were large-scale systems with a collector area of some 10,000 m². Denmark is a pioneer, where large-scale systems including seasonal storage systems achieve heat production costs of 2–6 ct / kWh. Local solar heating systems are also possible, but cannot serve as the sole heat source.
Pipeline network
The pipeline network transports the transfer medium (mostly hot water, with the first systems also steam) to the consumers. The transfer medium is conveyed in thermally insulated pipes in a continuous cycle. To avoid corrosion and hard deposits on the inner surfaces of the pipes, the water used is at least softened in the circuit.
The pipelines from the heat source to the heat sinks are called the flow , those from the heat sinks back to the heat source are called the return . The pipes that are in direct contact with the medium are called carrier pipes. Depending on the medium temperature, the required flow rate and static requirements are used as piping systems plastic sheath composite pipes , steel jacket tubes , spiral seam (and various flexible pipe systems composite piping systems pipe systems, without the composite) are used. The transmission temperatures depend on the technical generations and have been continuously reduced over time in order to reduce transmission losses and increase energy efficiency . Usual operating temperatures in older heating networks of the second generation are mostly in the range of more than 100 ° C, while the third generation often uses less than 100 degrees hot water. The transmission temperatures in low-temperature systems of the fourth generation are even lower at approx. 30 to 70 ° C. Depending on the specific specifications of the various district heating systems, the energy losses in the pipe system are around 5 to 20%. In many European countries the values are in the range of 5–10%, while in Russia they are around 20% and individual systems there show significantly higher losses.
Special features in pipe construction

The expansion coefficient of the steel pipes laid as district heating lines leads to changes in the length of the pipelines that should not be neglected, given the large distances to be bridged and temperature fluctuations. Measures such as the characteristic U-shaped compensation legs (also called U-bends) or expansion joints must therefore be included in the route planning . The expansion joints have now proven to be weak points in district heating networks, which is why they are replaced by U-bends or Z-bends whenever possible, especially in new buildings. Since expansion joints are usually made of different materials than the carrier pipes, electrochemical corrosion occurs more intensely due to the material transitions, which is intensified by the continuous mechanical load. This often leads to the expansion joints failing before the desired minimum service life of the district heating network. Z-bends are also used to compensate, especially in the area of house connections or when offsets in the district heating pipe are necessary anyway.
Leak monitoring systems
Leak monitoring is often integrated in pipe networks that are built up with plastic jacket composite pipes or steel jacket pipes. Plastic jacket composite pipes have to be equipped with a leak monitoring system during production, which is now the norm. In the case of steel jacket pipes, operation with permanent vacuum in the annulus of the pipelines is required, which is common practice. In pipe networks that are built up with flexible composite pipes, leak monitoring is possible as with plastic jacket composite pipes. However, this is not used everywhere. Flexible composite pipes have to be equipped with a leak monitoring system during manufacture, which, depending on the pipe manufacturer, is only carried out at the customer's request with correspondingly large purchase quantities.
Quality requirements for the district heating water
To avoid hard precipitates on the inner surfaces of the pipes of the heat generator, the circuit water is at least softened. Since desalinated water causes significantly less corrosion than just softened water, the aim is to keep the residual salt content in the circulating water of modern district heating networks as low as possible. This is achieved by using deionized water as make-up water and, if necessary, additional partial flow desalination of the amount of water circulating in the system. In particular, a maximum chloride ion concentration of 50 ppm is aimed for, since chloride ions accelerate the corrosion of metallic components. In addition to the salt content, the oxygen content also plays an important role in the tendency of water to corrode. Therefore, the oxygen concentration in the district heating circuit water is also limited. Another parameter that has an impact on the rate of corrosion is the pH value. The pH of the circulating water should therefore be raised to above 9 using alkalizing agents in order to reduce oxygen corrosion. In addition to sodium hydroxide solution, trisodium phosphate is also used as an alkalizing agent.
Handover to consumers

The heat transfer to the consumer usually takes place with the help of a transfer station , also called a compact station, which can consist of various components. In most cases, the district heating circuit is hydraulically separated from the consumer circuit by a heat exchanger , in a few cases (large consumers) the district heating circuit is connected directly. A heat pump can also be used instead of a transfer station, especially in low-temperature networks. The heat pump uses the supply of the heating network as a heat source and raises the temperature there to the temperature level used in the building, so that buildings with higher heating flow temperatures can also be connected to district heating systems on a low temperature basis. Alternatively, heat pumps can also draw their energy from the return of the district heating system and thus lower the return temperatures. In this way, the efficiency and the thermal transfer capacity of the heating network can be increased.
In residential buildings in particular, the design of the transfer station is not primarily based on the heating demand, but on the heat demand for hot water preparation. When preparing hot water in conventional systems, a hot water temperature of more than 60 ° C must be maintained to avoid contamination of the hot water system with legionella , so that legionella is thermally killed. Other options for avoiding Legionella infestation in low-temperature systems include chemical or physical treatment or reheating of the hot water using a heating rod or hot water heat pump. There are three variants available for hot water generation, depending on requirements:
- With the flow system, the required hot water is heated directly in the heat exchanger of the transfer station. This requires a correspondingly large heat exchanger with a correspondingly large district heating connection. On the other hand, the risk of contamination with Legionella is very low and the return of the district heating water is reduced to a low temperature level. The flow-through system is suitable for customers with a relatively uniform hot water requirement and for customers with a very low district heating connection power anyway, since the transfer station cannot be designed as small as required within the technically sensible framework.
- In the storage system, water is heated in a storage tank (“the storage tank is being charged”) and taken from it when required. The district heating connection can be designed much smaller. Instead, the risk of contamination with Legionella increases, which requires special protective measures (regular thermal disinfection). In the worst case, the temperature in the hot water storage tank can almost rise to the return temperature of the district heating water, which is an unfavorable operating condition, as only a small amount of heat is transferred to the storage tank in this area, which slows down heating to the maximum desired temperature. In addition, the amount of hot water available is limited by the volume of the storage tank. After removing the available amount, you have to wait until the memory has been loaded again. The additional space required by the store and the heat losses of the store are also disadvantageous. Storage systems are suitable for consumers with strongly fluctuating hot water requirements, such as individual houses.
- The storage loading system combines the flow system with the storage system. The included flow system is only designed for an average hot water requirement, so the district heating connection can be designed to be smaller than with the pure flow system. Peak loads are covered by a hot water storage tank that is charged during off-peak times.
Technical generations
Even if district heating systems are structured differently from city to city, four generations can be distinguished with different technical characteristics. In general, there is a trend towards lower transmission temperatures, lower material costs and prefabrication in factories, which is associated with fewer personnel involved in building the networks.
First generation
The first generation was introduced in the United States from the 1880s and then found in other parts of the world such as B. Europe spread. These systems used hot, pressurized water vapor as the transmission medium, which was transmitted in steel pipes insulated on site. The steam was mainly generated in central coal -fired boilers and a few power plants with combined heat and power . The radiators in the apartments worked at high temperatures of 90 ° C and more. As a result, this system had several disadvantages. Due to the high steam temperatures, the heat losses in the network were high, and there was corrosion in the return flow, which further reduced the energy efficiency of the overall system. There were also dangerous explosions of the steam pipes from time to time. The main reason for the introduction was to avoid boiler explosions in the apartments and to increase the living comfort. Only such district heating systems were used until around 1930; today the technology is considered obsolete. As of 2014, such systems were still being operated in the district heating systems built early in New York and Paris . B. in Salzburg , Hamburg and Munich the district heating systems have meanwhile been converted to more modern technology.
Second generation
The second generation appeared around 1930 and was the predominant type in newly installed district heating systems until the 1970s. These systems used pressurized water with temperatures mostly above 100 ° C as a heat transfer medium, which was transported in steel pipes insulated on site and conveyed through central pumping stations. In the apartments, radiators with heating temperatures of approx. 90 ° C were used, which were fed directly or indirectly. These systems were built, among other things, in the large district heating systems of the former Soviet Union . There the technical quality was mostly poor; In addition, there was no possibility of temperature control in the apartments. Such systems of better quality were also used in other parts of the world, where some of them still serve today as the older core of existing district heating networks. The motivation behind these district heating systems was mostly the saving of primary energy through the use of combined heat and power and the increase in heating comfort. The hot water was mainly generated in coal and oil power plants with combined heat and power, supplemented by some (fossil-fueled) hot water boilers.
Third generation

The third generation came up in the 1970s, then from the 1980s onwards, most district heating systems were built on this stand and many previous systems were converted to this stand. Since many manufacturers of components for such systems come from Northern Europe , this generation is z. T. called "Scandinavian district heating technology". Technically, this system is also based on hot water, which mostly reaches a temperature of less than 100 ° C. The water is transported through central pumping stations. In contrast to previous generations, the transport pipes, like most of the other components, are no longer insulated on site, but prefabricated and pre-insulated in factories and can then be sunk directly into the ground on site. The distribution stations are compact compared to their predecessors and the heat exchangers are made of stainless steel. In the apartments, radiators with average heating temperatures of approx. 70 ° C are used, which are supplied directly or indirectly; partly also underfloor heating . The heat is generated in central heating power plants with combined heat and power, in decentralized combined heat and power plants , by waste-to-energy plants and biomass power plants , supplemented by peak load boilers . Sporadically, geothermal and solar thermal energy is also fed into such networks as a supplement. After the oil crises in the 1970s, the main motivation for the development was to increase energy efficiency and replace crude oil with other energy sources such as coal , biomass and waste .
Fourth generation
The fourth generation (often also referred to as Heat Network 4.0 , 4th Generation District Heating or 4GDH ) is currently being researched / tested and will be used on a large scale from around 2020. This generation is mainly shaped by the requirements of climate protection and sustainable energy generation and the associated energy transition towards largely or completely renewable energy systems.
Renewable energies or waste heat from industrial processes should serve as a heat source; in addition to (biomass-fired) CHP systems, large heat pumps, among other things, should play an important role, resulting in a strong link with the electricity sector. By combining electricity and heat supplying CHP systems, heat pumps and heat storage , district heating systems of the 4th generation should also offer a lot of flexibility for energy systems with a high proportion of variable renewable energies such as wind energy and solar energy and thus compensate for their fluctuating energy supply; For example, by operating the heat pumps when there is a surplus of green electricity or, alternatively, the CHP systems when the green electricity is not produced to meet demand. This flexibility of the heating sector can play a key role in the integration of fluctuating renewable energy sources in sector-coupled energy systems. District heating systems of the 4th generation are also considered to be a key technology for the use of district heating systems in buildings that have only a low heating requirement due to good thermal insulation . In Denmark , the switch from third to fourth generation is already in progress (as of 2018).
According to the state of research, district heating systems play an important role in a sustainable energy economy, but further improvements are necessary for this. Among other things, district heating systems of the 4th generation in a sustainable energy system must u. a. have the following skills:
- Delivery of low temperature heat to new or energetically renovated buildings
- Transport of heat with low energy losses
- Absorption or recycling of low-temperature heat (e.g. waste heat from industrial processes with or without heat pumps ) and the ability to integrate renewable heat sources such as geothermal and solar thermal energy
- Ability to operate intelligently within the framework of integrated energy systems, d. H. energy systems based on sector coupling
Well-insulated, prefabricated pipes that work with water temperatures of 30 to 70 ° C in order to keep energy losses low and to enable high energy efficiency of the overall system are considered to be the core components of such systems. The circulation of hot water in the district heating network is to be carried out both centrally and decentrally to further improve flexibility. In addition, refurbished buildings in urban areas with a heat consumption of 50 to 150 kWh / m² and new buildings with less than 25 kWh / m² heat consumption per year are to be supplied, while heat pump heating systems are more energy-efficient and therefore more practical in sparsely populated areas . The heating in such low-temperature district heating systems should be done primarily with surface heating systems with a low flow temperature such as underfloor heating systems and with low-temperature radiators (50 ° C) with indirect supply. Hot water should either be supplied via heat exchangers at 40–50 ° C, or in low-temperature district heating systems with a flow temperature of only 30 ° C via a hot water heat pump. Lowering the flow temperature is an important factor in increasing the energy efficiency of the district heating system and building sustainable district heating networks. CHP systems can generate more electricity, waste heat from industry and geothermal energy can be fed in better and heat pumps can be operated with a higher coefficient of performance, and transmission losses also decrease. As a result, heat pumps can easily be integrated into 4th generation heating networks, while this is only possible with restrictions with systems of the 2nd and 3rd generation.
4th generation district heating systems are also gaining in importance as a flexibility component for the electricity market, which is increasingly characterized by fluctuating renewable energies, for example by operating heat pumps when there is a surplus of green electricity or, alternatively, the CHP systems when green electricity is not produced to meet demand. So far, the coupled generation of electricity and heat in CHP plants has been the most important link between the heat and electricity sectors. Central CHP plants allow an increased electricity yield due to economies of scale. Such flexible generation capacities fit well with the fluctuating electricity generation from renewable energies. In the future, heating networks can make an additional contribution to making the energy system more flexible by integrating flexibly controlled large heat pumps or various power-to-heat technologies. Heat storage systems should also offer flexibility for energy systems with a high proportion of variable renewable energies such as wind energy and solar energy and thus compensate for their fluctuating energy supply. Such systems, in which CHP systems, heat pumps and solar district heating are combined, are already available in some municipalities in Denmark and have been expanded for some time to include large heat storage systems with a capacity of over 100,000 m³, so that the right technology can be used depending on the prevailing weather conditions can.
Current research also includes new multi-line systems for district heating, which can offer more flexibility with regard to the feed-in of renewable energies. In two-wire district heating networks, consumers are primarily supplied with heat from the flow. Its temperature is inevitably based on the type of consumer in the network with the highest temperature level. In contrast, a multi-conductor heating network is designed with different conductor temperatures. As a result, the temperature level in the heating network is no longer determined solely by the consumer with the highest temperature requirements, but can be optimally adapted to the existing consumer and producer structure. There is no division into forward and reverse with this concept. The multi-conductor approach leads to a lowering of the average network temperature. This means that the network can also be characterized as a low-temperature heating network. The lower temperatures make it possible to use sources that deliver low-temperature heat and cannot be integrated in networks with high flow temperatures. Possible heat sources are solar thermal energy, waste water heat, environmental heat as well as industrial and commercial waste heat.
Special designs
Cold district heating
A special type of district heating network is the so-called "cold district heating", which was first used in the Arzberg power station . In this case, no hot steam is diverted into the turbine of a power plant, which causes a slight loss of efficiency in the generation of electricity, but instead the uncooled cooling water is taken after the turbine condenser. Since the temperatures in the district heating network are lower than in conventional heating networks, the flow temperature is instead raised to the required level by means of a heat pump . This design has several advantages over classic systems: Such a system can be retrofitted in any existing power plant without great structural effort, and the heating network can be built inexpensively with simple, uninsulated plastic pipes, since simple river water that is not under pressure is used. Under certain circumstances, a return to the power plant can be dispensed with if the water can be directed into a river after it has given off heat. The heat pumps also work very efficiently because the temperature difference to be bridged between the cooling water temperature (25–35 ° C) and the heating flow temperature is low. In this way, this system can be an economical alternative to conventional district heating systems. The prerequisites for this are not too long transport distances and little incline, as otherwise the pumping effort would be too great. Smaller systems that can get their heating energy instead of power plant cooling water from a large number of low-temperature heat sources such as groundwater wells , solar thermal collectors , industrial waste heat or agrothermal energy are referred to as so-called cold local heating systems.
District cooling
A large part of the available heat is only required in the cold winter months. That is why we are looking for ways to use the energy in summer too. District cooling is a sensible area of application . As in winter, the customer is supplied with hot water, which generates cold on site with the help of absorption chillers . This method is currently used for facilities with high cooling requirements, for example hospitals or shopping centers. In Chemnitz there is a central cold storage facility that supplies the city's facilities. It is also possible to couple district heating networks with cooling networks, so that the waste heat generated during cooling can be used for heating purposes. This can be done directly or via heat storage.
business
When designing and operating district heating networks and selecting the operating mode, numerous factors must be taken into account:
- Summation of the time-dependent customer requirements with regard to heat and mass flows taking into account simultaneity;
- Determination of the temperature curves in the pipe network taking into account the transport times in the pipe sections;
- Possibilities of heat storage in the network with the possible aim of reducing the peak load at the feed-in or, in the case of thermal power stations, to enable a limited priority for electricity generation;
- Pressure losses in the network sections;
- Design of pressure maintenance systems (e.g. dynamic medium pressure maintenance or static suction or final pressure maintenance) depending on the network geometry, the geodetic differences along the routes and the required volume equalization flows, as well as fixing the static pressure and classifying the maximum operating pressure curve in so-called pressure diagrams;
- Optimal dimensioning of the pipe diameter and pipe insulation with the aim of minimizing total annual costs;
- Optimal driving curves.
Legal situation in Germany
Definition by the Federal Court of Justice
The Federal Court of Justice defines the legal term district heating as follows:
"Produced from a not owned by the building owner heating system by a third party according to company economic point of its own heat and other delivered , it is district heating. The proximity of the system to the building being supplied or the availability of a larger pipeline network is irrelevant. "
Legal basis
The basis for the supply of district heating is a heat supply contract. The bases for this include the BGB and the ordinance on general conditions for the supply of district heating ( AVBFernwärmeV ) in the currently applicable version.
District heating and home ownership
The contracting party is usually the owner of the apartment or building, at least the person who has the authority to dispose of the house connection ( Section 2 (2) AVBFernwämeV).
District heating cartels
The number of cable manufacturers is relatively small. In the 1990s, for example, prices, market shares and offers to be submitted for major projects were determined in advance by a cartel. An example of this is the Leipzig-Lippendorf heating network. In the final bidding round, VEAG, which clearly felt it was a disadvantage that the six manufacturers did not want to bid against each other (prices were between 33 and 34 million ECU), asked Powerpipe to submit an offer. After receiving the Powerpipe offer for around DEM 26 million, VEAG decided on March 21, 1995 to award Powerpipe the contract. Annual price increases of 15% became common. Competitors outside the cartel were disadvantaged by low prices in their own market and by repression and threats from upstream suppliers. This prevented Powerpipe from fulfilling its obligations for the Leipzig-Lippendorf district heating network as a non-member and being able to deliver on time. The investigative commission found these agreements, some of them in writing. Members of this cartel were European manufacturers, some of which still exist today, e.g. B. Logstor, isoplus, Starpipe, Brugg, Ke-kelit. In addition to Germany, the cartel was also active in Austria and Switzerland and later also operated internationally. The managing directors met as an "elephant group" and determined the quotas and future market shares of the respective members. The decision of the court upheld the activities of the cartel members. The damaged company Powerpipe did not survive the process. Pipe manufacturers who focused on pure plastic pipes always remained outside the cartel. The cartel continued even after the prosecution's investigations, which was also recorded in the judgment.
District heating in individual states
Denmark
In 2013, 62% of all households in Denmark were heated with district heating; in urban areas there are almost exclusively district heating connections. In total, there were around 1.6 million households in Denmark with district heating, compared with around 400,000 households with natural gas heating and 314,000 households with oil heating. Around 30,000 households used heat pumps installed in their homes. Since the beginning of 2013, gas or oil heating may no longer be installed in new buildings in Denmark. The ban has been in effect for existing properties since 2016. Since the ban, sales of heat pumps have increased significantly. To finance the Danish energy transition, consumers have been paying a "security of supply fee" since the beginning of 2013. It is due on almost all fuels and increases gradually until 2020. In the end, each household should pay 175 euros more for heating.
At the moment, the district heating networks are mainly heated with biomass and fossil fuels, for which mainly combined heat and power plants and larger combined heat and power plants are used. In the course of the goal set by the Danish government to supply the country completely with renewable energies by 2050, fossil fuels will no longer be available in the future, while biomass is to be used primarily in the transport sector. Therefore, in the future, the district heating networks will mainly be fed with large heat pumps, which in turn will get their energy from wind energy and photovoltaic systems . In addition to supplying the district heating networks, these heat pumps should play an important role in balancing out the fluctuating electricity production from these energy sources. ´For several years there has been a strong expansion of large heat storage facilities in Denmark with a capacity of over 100,000 m³ in order to be able to operate the district heating systems more flexibly. In combination with CHP systems, large heat pumps or solar thermal systems, these enable variable operation of the producers, so that, depending on the current demand for electricity, CHP systems can supply electricity and heat or, alternatively, heat pumps or power-to-heat systems draw excess electricity from the grid and feed in heat or can save them temporarily.
Denmark is also a pioneer in solar district heating . As of September 2016, at least 26 larger systems with a cumulative collector area of 1,000,000 m² had been installed. By 2050, Denmark is aiming to generate around 40% of Danish heating requirements through solar thermal systems . 80% of this energy is to be obtained through solar district heating.
Germany

Around 9% of the total heat demand in Germany is now covered by heating networks and 14% of the demand for residential buildings. In 2017, district heating networks delivered 161 TWh of energy, minus 7% heat losses in the networks, 149 TWh reached customers. The most important customers were private households ahead of industry, which together demanded two thirds of the energy. Around half of the energy was obtained from natural gas, around a quarter from coal, renewable energies accounted for 7% and the trend is rising. The length of the German district heating network in 2017 was a total of 26,400 km. The share of district heating in the energy market is much higher in the eastern federal states than in the western federal states.
The largest German district heating networks can be found in Berlin , Hamburg and Munich . Flensburg is one of the cities with the highest market share for district heating (> 90%). The model for Flensburg were those Danish cities on the Baltic Sea that have roughly the same district heating density.
The market share of electricity from combined heat and power (CHP) was 7% of gross electricity generation in 2006 and in 2013 96 TWh of electricity were generated in combined heat and power (CHP); the share was thus around 16%.
1992 | 2005 | |
---|---|---|
Companies | 216 | 240 |
Network length [km] | 14,136 | 19,284 |
House transfer stations | 218.841 | 324,531 |
Heat connection value [MW] | 55,336 | 52,729 |
Heating network feed [TJ / a] | 349,413 | 313.902 |
Heating network feed-in [GWh / a] | 97.060 | 87,857 |
Share of CHP [%] | 66 | 83 |
Share of heating plants [%] | 37 | 16 |
Share of waste heat utilization [%] | 2 | 1 |
Total electricity generation from CHP [GWh] | k. A. | 35,604 |
of which from own systems [GWh] | 22,700 | 31,103 |
In existing heating networks, 83% of the thermal energy passed through is obtained from CHP systems; the vast majority of these are fossil fuels. Combined heat and power reduces emissions of the greenhouse gas carbon dioxide (CO 2 ). The Arbeitsgemeinschaft für Wärme und Heizkraftwirtschaft (AGFW) puts the CO 2 savings through the use of district heating for 2002 at 7.5 million tons. Biomass thermal power stations have a particularly favorable CO 2 balance .
The RE share in the district heating supply is currently around 10% and is based on the combustion of biomass, the share of which is currently around 4%, and above all on waste heat from waste incineration plants, which, due to the biogenic share of waste, is assessed to be 50% renewable becomes.
An expansion of district heating, which is generated in thermal power stations, still offers significant potential for reducing emissions, especially CO 2 . The district heating networks have been expanded in the last ten years in terms of network length and the number of transfer stations. Nevertheless, the connection values and the delivery quantities are declining because the improved thermal insulation of the buildings is reducing demand. In Germany, large power plants are still operated without district heating extraction, although this would be technically possible with the systems. The three convoy-type nuclear power plants ( Isar 2 , Emsland , Neckarwestheim 2 ) should be mentioned here in particular . In older district heating networks in particular, the situation is often encountered in which mainly large customers are connected, but not residential buildings along the district heating lines. Above all, obstacles under private law, but also the associated investment costs, make the subsequent connection of these residential buildings more difficult, whereas the existing line capacities usually do not represent an obstacle due to the generally falling heat demand. In new building areas developed with district heating, municipalities can impose compulsory connection and use for the use of district heating for all properties for reasons of climate protection. This compulsory connection and use is legal with a judgment of the Federal Administrative Court .
The Federal Cartel Office subjected the district heating market to a so-called "sector investigation" after indications of abusively excessive prices. The authority does not determine an overall excessive price level in the district heating sector. However, it suspects the suppliers behind at least eleven district heating networks with a pipeline length of more than one kilometer of improperly excessive prices and checked in 2012 whether formal proceedings should be initiated. It was unclear which suppliers and networks are affected. Stiftung Warentest had made specific inquiries and sees the authority as obliged to name the companies concerned.
Berlin
The Berlin district heating network is considered the largest in Western Europe and is only surpassed by Warsaw and Moscow in Europe. With its 1,875 km of pipeline it supplies 1.2 million residential units. In addition to central heating and power stations, Vattenfall alone operates at least 50 decentralized combined heat and power plants and 235 other supply systems in Berlin. The fuel mix in the eleven Berlin thermal power stations shows the following distribution: 50% hard coal, 31% natural gas, 14% lignite and 5% other energy sources such as wood. The share of renewable energy sources in 8,500 GWh of heat sales is therefore currently relatively low. Depending on the end customer, temperatures of up to 135 ° C can be reached, with the pipes having to withstand up to 16 bar. The maximum diameter of the lines is 1.22 m. The network is expanded by around 25 km annually through constant expansion.
Greifswald
The Lubmin nuclear power plant (also: Nuclear Power Plant North) built by Greifswald in Lubmin , 20 km away , covered around 10% of the GDR's electricity needs and, until 1990, also served primarily to supply the southern new development areas of Greifswald with district heating. The aim was to be the first city in the GDR to be free of chimneys . After the power plant blocks were shut down, the missing district heating was provisionally generated by oil boilers and, from 1995, mostly by natural gas-powered combined heat and power plants and a gas turbine system, all directly in Greifswald.
Flensburg
In the 1960s, the self-help building association began to supply houses in the Fruerlund district with district heating (see there ). Finally, in 1969, the Stadtwerke Flensburg began building the municipal district heating network, to which that of the self-help building association was connected. The municipal power station was converted into a thermal power station with 170 MW electrical and approx. 800 MW thermal output by 1971. Hard coal is used as the primary energy, in 2004 approx. 300,000 t. Since 2008, substitute fuels and wood chips have also been burned; their share can make up up to 25% of the fuel. Four reserve heating plants are integrated into the network. The supply area includes the city of Flensburg , the city of Glücksburg , the municipality of Harrislee and the Danish border town of Padborg . From 2010 the community of Wees (1 km east of Flensburg) will be connected to the district heating network.
The pipeline network covers 577 km. This network supplies over 15,000 connections in the supply area with approx. 1 billion kWh, that is approx. 1,000 GWh, per year (as of 2004).
The share of district heating in the heating market (space heating and hot water preparation) in the Flensburg supply area is around 98%. This high rate is based primarily on the city's mandatory connection and use.
Kiel
In Kiel, 60 percent of municipal utility customers are supplied through the 1368 meter long Kiel district heating tunnel, which runs below the Kiel Fjord . The heat supply is largely provided by the Kiel joint power plant, which will be replaced in 2019 by a gas engine power plant currently under construction .
Austria
In the 1950s in Austria the district heating supply in the cities of Baden , Klagenfurt and Wels ; began. In 1980, 83,000 apartments were heated with district heating, ten years later 226,000 and in 2010 already 758,000 apartments. In 2012, 22% (2010 21%) of Austrian apartments were heated with district heating. In Austria, around 14.9 TWh of district heating was consumed in 2003, especially in large urban areas. In 2013, biomass and household waste , natural gas and heating oil , as well as 4% coal and 7% mineral oils , were predominantly used as fuels of over 40% . The use of natural gas is declining: if the use of natural gas for district heating production was over 50% in 2007, it fell to 39% in 2013. Outside of Vienna and the city of Salzburg, renewable energy sources have prevailed, especially in the smaller district heating networks in rural regions , thanks to appropriate financial support.
Lower Austria

In Lower Austria, in addition to the large biomass cogeneration plants in the metropolitan areas, there are also smaller, predominantly biomass-operated district heating plants. These are operated by large energy suppliers, municipalities and businesses, but also by cooperatives. In 2005, 271 biomass heating plants were operated with wood and wood waste, another nine with straw. These 280 systems achieved a total output of 322 MW. The construction of such systems is funded with up to 40% of the net investment costs. In 2006, the output of the biomass heating plants and the thermal power plants rose to 589 MW with 345 installed systems, in 2007 it was already 684 MW with 371 systems. The largest district heating networks operated with renewable raw materials include the Baden district heating network and the district heating network in the Mödling area .
Upper Austria
In Linz , 90% of all public buildings and around 55,000 apartments are supplied with district heating by Linz AG ; the market share of district heating is 65%. Since 2005, Linz has been using a biomass thermal power station with a thermal output of 21 MW to generate heat ; From 2012, waste incineration will also feed into the network, so that around half of the heat in Linz is renewable. The (heating) power plant of voestalpine Stahl GmbH in Linz is also operated with coke oven gas , furnace gas , crucible gas (created when oxygen is blown into crucibles during steel production ) and natural gas and generates electricity and superheated steam; it also supplies waste heat as district heating to private households in the City area of Linz. In Mauthausen , Gunskirchen and Schärding , new biomass district heating networks were set up from 2010. The Energie AG operates district heating networks in Kirchdorf an der Krems , Riedersbach , Vöcklabruck , Aschach on the Danube and Steyr , which is powered by a second biomass power plant since 2012 found.
Salzburg
In the city of Salzburg around 25,000 customers are supplied from an approx. 250 km long district heating network, which is largely fed by fossil-fuel cogeneration. By 2006, 67 biomass heating plants with an installed capacity of around 150 MW were already in operation in the entire state, and the CO 2 savings achieved as a result amount to over 125,000 t per year. In the area of Lofer and St. Martin bei Lofer there is more than 50% supply of biomass district heating in the total heat generation.
Styria
Between 1985 and 2012, over 150 local and district heating networks were built in Styria that are operated with biomass.
In 2012, the district heating supply in the provincial capital Graz was supplemented with the supply from the coal-fired power plant Mellach with the new fossil-fueled gas-fired combined cycle power plant Mellach. Around 80% of Graz's district heating requirements are covered by this power plant location. Around 30% of Graz households are supplied with district heating. Due to the significantly changed market conditions on the electricity exchanges, the Mellach gas-fired combined cycle power plant has mostly only been used to support the grid since 2013.
Currently (as of 2016) Energie Steiermark and other companies are planning to cover part of the district heating with solar thermal energy . For this purpose, a solar park consisting of solar collectors with a size of 15 to 45 ha and an associated seasonal heat storage facility is to be built. If the 45-ha variant is implemented, the system should deliver around 230 GWh of thermal energy, which corresponds to around 20% of Graz's district heating requirements. The associated storage facility for seasonal compensation would have a capacity of around 1.8 million cubic meters; the cost of this variant is estimated at around 200 million euros. In terms of the size of the area, there is only room for it outside the city of Graz.
Before this system was built, a 7,750 m 2 (= 0.77 ha) solar collector was installed on the grounds of the Graz-Süd district heating plant, Puchstrasse 51. The system built by SOLID International is the largest solar thermal system in Austria and is also being scientifically investigated (as of September 5, 2016).
For the first use of waste heat from the paper mill in Gratkorn , a few kilometers north of the city of Graz, a district heating pipe has been laid in the ground since summer 2016. The Marienhütte steelworks, located a little south of the main train station, is to provide waste heat as district heating for the adjacent urban development area Reininghaus . Year-round heat storage is to be installed in grain silos.
In Bad Aussee , a biomass district heating network was set up by Wien Energie in 2002 with an annual output of 23 GWh and 13 MW connected load, which was acquired by the local state energy supplier in 2009.
Tyrol
A district heating network has existed in Kufstein since 1978, which supplies around 4,000 households over a length of 29 km; In 2003, the heat generation from block heating engines and natural gas boilers was converted to a biomass cogeneration plant. A large district heating network was set up in 2011 in the Wattens, Innsbruck Neu-Rum and Olympische Dorf area, which is fed with waste heat from industry.
Vienna
Austria's largest district heating network exists in Vienna and is operated by Wien Energie . In the 2004/2005 financial year, 5,163 GWh were sold, 1,602 GWh of which to a total of 251,224 private apartments and 3,561 GWh to a total of 5,211 major customers. A small part of 22% is generated in the three large waste incineration plants, Spittelau , Simmeringer Haide and Flötzersteig , which produce around 116 GWh of electrical energy and around 1,220 GWh of district heating. 50% of the district heating generated comes from fossil caloric power plants such as the Simmering power plant , where it is obtained from the primary energy source natural gas. The remaining 20% of the district heating is generated by large peak-load heating plants from natural gas. In addition, there is Simmering, the largest biomass power plant in Vienna as of 2010, which supplies around 1% of the district heating and supplies 12,000 households with district heating.
In addition, there is a smaller network for district cooling in Vienna . In 2014, the city built another natural gas-fired district heating plant in the Arsenal with an output of 340 MW and can already generate more than 1300 MW district heating from gas in boilers.
Carinthia
On August 12, 1947, the foundation stone was laid for Austria's first district heating plant in Klagenfurt, which went into operation in March 1949. The first expansion stage had an output of 10 MW and a network flow temperature of 180 ° C. In 1953, the network's peak heat output was already 33 MW. In 2012, around 450 GWh of district heating was fed into the network every year. In recent years, many biomass heating plants have been built in Carinthia (e.g. Rennweg am Katschberg , Klagenfurt- Emmersdorf) to improve the CO 2 balance .
Sweden
District heating systems are very important in Sweden , almost every municipality has a district heating network. In total, approx. 57% of the heating requirements of private households and businesses are covered by district heating. Until the end of the 1970s, the heat was generated almost exclusively by oil firing , after which the systems were quickly converted to alternative fuels as a result of the oil crisis. As of 2014, biomass is the most important fuel; waste is also converted into electricity in waste-to-energy plants and excess heat is fed into the district heating network. Fossil fuels, on the other hand, are hardly ever used in district heating. Since the 1980s a number of large heat pumps have also been installed to feed the heating networks. Overall, large heat pumps with a heating capacity of 1523 MW were installed between 1981 and 2013, of which a large part (approx. 80%) was still in operation in 2013.
Stockholm has a large district heating network that covers an annual heating requirement of more than 12 TWh and supplies around half of the households in the region with heat. It has its origins in the 1950s, then it was expanded and it grew together from different cells. Even today it still consists of two independent systems operated by different companies; a technical merger of the two networks is being planned. Overall, the network has an installed thermal output of 4.8 GW. In addition to several thermal power stations with an electrical output of 556 MW, the Stockholm heating network is one of only a few district heating systems in the world to be supplied with heat by a series of large heat pumps. These get their heat u. a. from seawater and waste water from sewage treatment plants and deliver a thermal output of 660 MW with an electrical output of 200 MW. The COP of these plants is between 3.3 and 3.5. Electric boilers with an output of 300 MW are also installed.
Switzerland
In Switzerland, the share of district heating in the total heat supply is 3.5%.
Basel
The district heating network in Basel is 198.2 km (as of 2004) long. According to the network operator IWB (Industrielle Werke Basel), a few kilometers are added every year. In addition to hospitals, public buildings, industrial and commercial enterprises, around 40,000 apartments are connected. The district heating supply area was established in 1979. In this area there is a connection obligation for all houses. In 2004, 1,003 million kWh of district heating was produced in Basel, 55% of which came from natural gas, sludge and oil and 45% from garbage.
The district heating network in Basel has been in operation since 1942. It is a hot water system . Today the district heating system has three centers (district heating power station Voltastraße, waste recycling plant and heating power station SBB station). In the Voltastrasse district heating power station, natural gas is converted into heat and electricity. The heat gained is distributed underground through a ring-shaped, well-insulated pipeline network throughout the district heating area and the electricity produced is fed into the network.
Lower Aare Valley
In the lower Aare valley there is a relatively extensive district heating network (Regional Fernwärme unteres Aaretal-REFUNA), which is mainly supplied by heat extraction from the Beznau nuclear power plant . The total length of the district heating network is 134 km, with the main network accounting for 36 km. 11 municipalities are connected to the district heating network, a total of around 15,000 residents are supplied with 170 million kWh of heat annually.
The construction of the district heating network began in the early 1980s in response to the oil crisis, and in the winter of 1983/84 the Paul Scherer Institute near the nuclear power plant was the first to be connected to the network.
For district heating in the NPP, steam is fed after the high-pressure turbines at 127 ° C into a heat exchanger, where it heats the pressurized water in the district heating network to 120 ° C. Both blocks of the NPP can feed out district heating so that it is also available when a block is under revision. There are four oil-fired heating plants to further increase availability. With maximum heat extraction, the electrical output of the NPP is reduced by up to 7.5 MW.
Eastern Europe

In the area of the former Eastern Bloc countries, there are extensive district heating networks in many cities. One of the reasons for this is that in the former Eastern Bloc countries there were no barriers under private law to the expansion of district heating. The pipes were mostly of very poor quality due to the use of inferior raw materials. In particular, the thermal insulation made of glass or mineral fiber wool was often characterized by a disproportionately high thermal conductivity and a short service life. In severe winters, this often led to the pipes freezing, which also meant that all connected heating systems failed. The freezing of the pipelines was favored by the fact that overhead lines were laid more frequently, which, unlike underground pipelines, are exposed to the weather. Many district heating networks are currently being refurbished in the Eastern European countries and brought up to today's standards, which is why plastic jacketed composite pipes are now also manufactured there in accordance with the current European standard (EN 253), close to the market.
In Russia, in 1983, the cities of Voronezh and Gorky (now Nizhny Novgorod ) began to build the Voronezh and Gorky nuclear heating plants in order to supply the cities with district heating with the heat generated in the reactor. Both projects were abandoned. Plants in operation that generate district heating from nuclear energy in Eastern Europe include the Bilibino nuclear power plant in Russia and the Bohunice nuclear power plant in Slovakia with its V2 plant.
See also
Web links
- Ordinance on general conditions for the supply of district heating (AVBFernwärmeV) on juris.de (PDF; 37 kB).
- AGFW Energy Efficiency Association for Heating, Cooling and KWK eV
- Bundesverband Fernwärmeleitungen eV
- Swiss District Heating Association (VFS) .
- Vacuum Super Isolation (VSI) district heating pipes (PDF file; 264 kB).
- District heating research institute: history of district heating. In: fernwaerme.de. May 18, 2009, archived from the original on July 12, 2007 ; Retrieved June 6, 2009 .
- Jürgen Falliano: Cold local and district heating. In: Energy-Control-System.com. January 13, 2014, accessed January 13, 2014 .
Individual evidence
- ^ A b c Sven Werner: International review of district heating and cooling . In: Energy . tape 137 , 2017, p. 617-631 , doi : 10.1016 / j.energy.2017.04.045 .
- ↑ a b c d e f g h i j Henrik Lund et al .: 4th Generation District Heating (4GDH) Integrating smart thermal grids into future sustainable energy systems . In: Energy . tape 68 , 2014, p. 1–11 , doi : 10.1016 / j.energy.2014.02.089 .
- ^ MA Sayegh et al .: Trends of European research and development in district heating technologies . In: Renewable and Sustainable Energy Reviews . tape 68 , no. 2 , 2017, p. 1183–1192 , doi : 10.1016 / j.rser.2016.02.023 .
- ↑ a b c d Abdul Rehman Mazhar et al .: A state of art review on district heating systems . In: Renewable and Sustainable Energy Reviews . tape 96 , 2018, p. 420-439 , doi : 10.1016 / j.rser.2018.08.005 .
- ↑ a b c d e M.A. Sayegh et al .: Heat pump placement, connection and operational modes in European district heating . In: Energy and Buildings . tape 166 , 2018, p. 122-144 , doi : 10.1016 / j.enbuild.2018.02.006 .
- ↑ a b Bjarne Bach et al .: Integration of large-scale heat pumps in the district heating systems of Greater Copenhagen . In: Energy . tape 107 , 2016, p. 321–334 , doi : 10.1016 / j.energy.2016.04.029 .
- ^ Rasmus Lund, Urban Persson: Mapping of potential heat sources for heat pumps for district heating in Denmark . In: Energy . tape 110 , 2016, p. 129-138 , doi : 10.1016 / j.energy.2015.12.127 .
- ^ Andrei David et al .: Heat Roadmap Europe: Large-Scale Electric Heat Pumps in District Heating Systems . In: Energies . tape 10 , no. 4 , 2017, p. 578 ff ., doi : 10.3390 / en10040578 .
- ↑ a b Fabian Levihn: CHP and heat pumps to balance renewable power production: Lessons from the district heating network in Stockholm . In: Energy . tape 137 , 2017, p. 670-678 , doi : 10.1016 / j.energy.2017.01.118 .
- ↑ Jon Gustav Kirkerud, Erik Trømborg, Torjus Folsland Bolkesjø: Impacts of electricity grid tariffs on flexible use of electricity to heat generation . In: Energy . tape 115 , 2016, p. 1679–1687 , doi : 10.1016 / j.energy.2016.06.147 .
- ↑ Matthias Koch et al .: Model-based assessment of network expansion in the European network and flexibility options in the German electricity system in the period 2020–2050 . In: Zeitschrift für Energiewirtschaft . tape 39 , 2015, p. 1-17 , doi : 10.1007 / s12398-015-0147-2 .
- ^ Brian Vad Mathiesen et al .: Smart Energy Systems for coherent 100% renewable energy and transport solutions . In: Applied Energy . tape 145 , 2015, p. 139-154 , doi : 10.1016 / j.apenergy.2015.01.075 .
- ↑ See Henrik Lund : Renewable Energy Systems: A Smart Energy Systems Approach to the Choice and Modeling of 100% Renewable Solutions , Academic Press 2014, chapter 5, especially p. 96.
- ↑ See Viktor Wesselak , Thomas Schabbach , Thomas Link, Joachim Fischer: Handbuch Regenerative Energietechnik , Berlin / Heidelberg 2017, pp. 419–422.
- ↑ planning manual of the company isoplus, chapter project planning
- ↑ a b AGFW guideline, FW 510 requirements for the circulation water of industrial and district heating systems, as well as instructions for their operation; 11/03
- ↑ Quality requirements for district heating water VGB-M 410 N 1994-02
- ↑ Requirements for the circulation water in industrial and district heating systems as well as instructions for their operation, VdTÜV bulletin 1466, 1989-02
- ↑ Dietrich Schmidt et al .: Low Temperature District Heating for Future Energy Systems . In: Energy Procedia . tape 116 , 2017, pp. 26–38 , doi : 10.1016 / j.egypro.2017.05.052 .
- ↑ Drinking water heating ( Memento from April 3, 2011 in the Internet Archive )
- ↑ Martin Pehnt et al. Heating networks 4.0, final report. Short study on the implementation of the measure "Model project for renewable energies in highly efficient low-temperature heating networks" . Institute for Energy and Environmental Research Heidelberg . Retrieved March 24, 2018.
- ^ Henrik Lund et al .: The status of 4th generation district heating: Research and results . In: Energy . tape 164 , 2018, p. 147–159 , doi : 10.1016 / j.energy.2018.08.206 .
- ↑ Xiaochen Yang et al .: Energy, economy and exergy evaluations of the solutions for supplying domestic hot water from low-temperature district heating in Denmark . In: Energy Conversion and Management . tape 122 , 2016, p. 142–152 , doi : 10.1016 / j.enconman.2016.05.057 .
- ^ Henrik Gadd, Sven Werner: Achieving low return temperatures from district heating substations . In: Applied Energy . tape 136 , 2014, pp. 59-67 , doi : 10.1016 / j.apenergy.2014.09.022 .
- ↑ a b Dunkelberg, Elisa; Bachmann, Max; Faster, Andreas; Schröder, Sabine; Bach, Nicolas: Framework conditions for the feasibility of LowEx multi-conductor heating networks . Ed .: Hermann Rietschel Institute. Berlin 2017.
- ↑ a b Cf. Michael Sterner , Ingo Stadler: Energy Storage - Demand, Technologies, Integration . Springer, Berlin 2014, pp. 133f.
- ^ Leonhard Müller: Handbook of the electricity industry: Technical, economic and legal bases . Berlin / Heidelberg 1998, p. 266f.
- ↑ District cooling concept: cooling from the thermal power station . In: Spiegel Online. Retrieved November 3, 2008.
- ↑ Dietmar Schüwer Conversion of the heat supply structures . In: Energy industry issues of the day 11/2017. Retrieved January 25, 2018.
- ^ BGH judgment of October 25, 1989, NJW 1990, 1181.
- ↑ AVBFernwärmeV
- ↑ so also Landgericht Frankfurt am Main RdE 1989, p. 165 f.
- ^ Judgment of the Court of Justice (Grand Chamber) June 28, 2005
- ↑ a b Danes forbid oil and gas boilers . In: Badische Zeitung , February 28, 2013. Retrieved February 17, 2017.
- ^ Warmth turnaround "There are no majorities for hard instruments" . In: Tagesspiegel , January 9, 2017. Retrieved February 17, 2017.
- ↑ Viktor Wesselak , Thomas Schabbach , Thomas Link, Joachim Fischer: Handbuch Regenerative Energietechnik , Berlin / Heidelberg 2017, p. 419.
- ↑ Schneller, Andreas; Leonard Frank; Walter Kahlenborn: Heating Networks 4.0 in the Context of the Heating Turnaround - Political Recommendations for Action for Decarbonising the Pipeline Heat Supply . Ed .: adelphi. Berlin 2018, p. 5 .
- ↑ In 2017, network operators provided 161 TWH district heating . In: Euwid Neue Energie , November 9, 2018. Accessed November 10, 2018.
- ↑ Stumbling blocks in series . In: vdi nachrichten , March 21, 2019. Retrieved March 24, 2019.
- ↑ a b c AGFW Industry Report 2006 ( Memento from February 15, 2009 in the Internet Archive )
- ↑ Federal Ministry for Economic Affairs and Energy, Public Relations Department: Potential and cost-benefit analysis of the possible uses of combined heat and power (implementation of the EU Energy Efficiency Directive) and evaluation of the KWKG in 2014. In: www.bmwi.de. Retrieved April 23, 2016 .
- ↑ 2003 work report of the AGFW Working Group for Heat and Power Management .
- ↑ Schneller, Andreas; Leonard Frank and Kora Töpfer: Heating Networks 4.0 in the context of the heating transition. Analysis of the regulation and funding landscape for innovative heating network systems. Ed .: adelphi. Berlin 2017, p. 12 .
- ↑ Maaß, Christian; Sandrock, Matthias; Schaeffer, Roland: District heating 3.0. Strategies for a future-oriented district heating policy. Study on behalf of the Bundestag parliamentary group Bündnis 90 / Die Grünen. Ed .: HIR Hamburg Institut Research gGmbH. Hamburg 2015, p. 18 .
- ↑ BVerwG judgment of January 25, 2006, Az. 8 C 13.05 ( Memento of July 30, 2012 in the web archive archive.today )
- ↑ Bundeskartellamt: Final report on the district heating sector investigation margin no. 6 ( Memento from October 31, 2013 in the Internet Archive )
- ^ Stiftung Warentest: District heating supplier under suspicion
- ↑ State Energy Program Berlin 2006–2010. ( Memento of the original from March 4, 2016 in the Internet Archive ) Info: The archive link was inserted automatically and has not yet been checked. Please check the original and archive link according to the instructions and then remove this notice. (PDF).
- ↑ Vattenfall - District heating for Berlin (PDF).
- ^ Greifswald district heating producers, as of October 17, 2010 ( Memento from February 17, 2010 in the Internet Archive )
- ↑ Flensburger Tageblatt : Housing in Flensburg: The days of the high-rise are numbered , from March 14, 2017; accessed on: March 14, 2017
- ↑ greenco2ncept . Website of the Stadtwerke Flensburg. Retrieved January 28, 2012 ( Memento January 15, 2012 in the Internet Archive )
- ↑ Century building lasted 17 years. In: Kieler Nachrichten, March 13, 2009 ( Memento from January 28, 2013 in the Internet Archive )
- ^ Chronicle, Stadtwerke Klagenfurt Group, accessed on May 19, 2012 ( Memento from October 15, 2011 in the Internet Archive )
- ^ Chronicle of Elektrizitäts-Werk Wels AG, accessed October 2012 ( Memento from September 16, 2009 in the Internet Archive )
- ↑ a b Mag. Michael Mock; Fachverband Gas Wärme ( Memento of the original from January 12, 2014 in the Internet Archive ) Info: The archive link was automatically inserted and not yet checked. Please check the original and archive link according to the instructions and then remove this notice. Retrieved May 19, 2012.
- ↑ Fachverband Gas Wärme: Figures for 2012 ( Memento of the original from November 9, 2014 in the Internet Archive ) Info: The archive link was inserted automatically and has not yet been checked. Please check the original and archive link according to the instructions and then remove this notice. (PDF).
- ↑ a b Bioenergy in Salzburg ( Memento of the original from June 9, 2012 in the Internet Archive ) Info: The archive link was automatically inserted and not yet checked. Please check the original and archive link according to the instructions and then remove this notice.
- ↑ Local heating from biomass in Lower Austria
- ↑ Basic data 2011 bioenergy (PDF; 7.2 MB). ( Memento from April 25, 2012 in the Internet Archive )
- ↑ Lower Austria Energy Report 2007.
- ↑ LINZ AG district heating network
- ↑ Linz AG: Press conference 40 years of district heating, 2010 ( Memento from November 21, 2012 in the Internet Archive )
- ↑ Linz AG, biomass cogeneration plant
- ↑ linzag.at
- ↑ voestalpine environmental declaration 2007 ( memento of the original dated December 8, 2015 in the Internet Archive ) Info: The archive link was inserted automatically and has not yet been checked. Please check the original and archive link according to the instructions and then remove this notice. (PDF; 2.6 MB).
- ↑ Linz - KWG's largest district heating network ( Memento from November 18, 2011 in the Internet Archive )
- ↑ Gunskirchen market town: Opening of the B3 energy biomass heating plant Gunskirchen
- ^ Energie AG Oberösterreich Wärme: Locations
- ^ Energy Contracting Steyr GmbH
- ↑ Fernwärme Steyr - your partner for environmentally friendly and sustainable district heating
- ^ Salzburg AG: District heating
- ↑ Map: Local biomass heating in Salzburg ( memento of the original from March 2, 2009 in the Internet Archive ) Info: The archive link was automatically inserted and not yet checked. Please check the original and archive link according to the instructions and then remove this notice.
- ↑ State of Styria: Expansion of biomass district heating ( memento of the original from November 9, 2014 in the Internet Archive ) Info: The archive link was automatically inserted and not yet checked. Please check the original and archive link according to the instructions and then remove this notice.
- ↑ District heating for Graz from the Mellach gas-fired combined cycle power plant ( Memento of the original from May 22, 2012 in the Internet Archive ) Info: The archive link was automatically inserted and has not yet been checked. Please check the original and archive link according to the instructions and then remove this notice. ; Retrieved May 19, 2012.
- ↑ ORF: composite mothballed a power plant Mellach
- ^ ORF: Mellach power plant: Opened in 2011, mothballed in 2014
- ↑ Big Solar. The world's largest solar storage system is supposed to heat Grazern. In: Small newspaper . February 27, 2016. Retrieved February 28, 2016.
- ↑ Information Company SOLID International, Graz, September 5, 2015.
- ↑ Wirtschaftsblatt ( Memento from January 15, 2013 in the web archive archive.today )
- ↑ Construction and Environment Office of the City of Kufstein: Kufstein Environment 2009. ( Page no longer available , search in web archives ) Info: The link was automatically marked as defective. Please check the link according to the instructions and then remove this notice. (PDF; 2.7 MB).
- ↑ Bioenergy Kufstein
- ↑ orf.at: The largest district heating network in Tyrol is being planned.
- ↑ My Bezirk.at: district heating expanding the network further from 25 October 2011.
- ↑ Wien Energie yearbook 2013 (PDF).
- ↑ Wien Energie: Forest Biomass Power Plant ( Memento from June 23, 2013 in the Internet Archive )
- ↑ ARSENAL DISTRICT HEATING PLANT, VIENNA
- ↑ energyagency.at (PDF).
- ^ Energie Klagenfurt GmbH: Warmth. (PDF; 135 kB). ( Memento from January 16, 2014 in the Internet Archive )
- ↑ Rennweg am Katschberg: Guided tours of the biomass district heating supply system Katschberg ( Memento from June 23, 2010 in the Internet Archive )
- ↑ Kleine Zeitung: Klagenfurt-Emmersdorf: Biomass heating plant "burns" !, Nov. 15, 2007 ( Memento from January 14, 2013 in the web archive archive.today )
- ^ Lorenzo Di Lucia, Karin Ericsson: Low-carbon district heating in Sweden - Examining a successful energy transition . In: Energy Research & Social Science . tape 4 , 2014, p. 10-20 , doi : 10.1016 / j.erss.2014.08.005 .
- ↑ Kerstin Sernhed et al .: Synthesis of recent Swedish district heating research . In: Energy . tape 151 , 2018, p. 126–132 , doi : 10.1016 / j.energy.2018.03.028 .
- ↑ Helge Averfalk et al .: Large heat pumps in Swedish district heating systems . In: Renewable and Sustainable Energy Reviews . tape 79 , 2017, p. 1275–1284 , doi : 10.1016 / j.rser.2017.05.135 .
- ↑ District heating in new ways . Invitation to a specialist conference of the Association for District Heating Switzerland (VFS) in 2008, accessed on June 28, 2008 (PDF) ( Memento of the original from October 5, 2015 in the Internet Archive ) Info: The archive link has been inserted automatically and has not yet been checked. Please check the original and archive link according to the instructions and then remove this notice.
- ↑ Home. Regional district heating Lower Aare Valley (REFUNA), accessed on September 7, 2015 .