energy transition

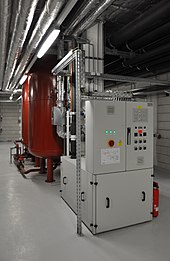

Energiewende is the German-speaking term for the transition from the unsustainable use of fossil fuels and nuclear energy to a sustainable energy supply using renewable energies . The term was culturally received after the book Energiewende - Growth and Prosperity without Petroleum and Uranium by the Öko-Institut published in 1980 and has already been adopted as a loan word in other languages (for example "The German Energiewende" or "A Energiewende alemã" ).
The aim of the energy turnaround is to minimize the ecological, social and health problems caused by the conventional energy industry and to fully internalize the external costs incurred, which have so far hardly been priced in the energy market . In view of the largely human-caused global warming , the decarbonization of the energy industry is particularly important today - by ending the use of fossil fuels such as oil , coal and natural gas . The finiteness of fossil fuels and the dangers of nuclear energy are also important reasons for the energy transition. The solution to the global energy problem is considered to be the central challenge of the 21st century.
The energy transition encompasses the three sectors of electricity , heat and mobility , as well as the perspective of turning away from fossil raw materials, with their material use in plastics or fertilizer production , for example . A coal and oil phase-out associated with the energy transition must also mean that significant amounts of the available energy sources must remain in the ground. Essential elements of the turnaround are the expansion of renewable energies, combined with the construction of energy storage systems , the increase in energy efficiency and the implementation of energy-saving measures . Renewable energies include bioenergy , geothermal energy , hydropower , ocean energy , solar energy ( solar thermal , photovoltaics ) and wind energy . Conceptually, the sector coupling plays an important role, in particular the electrification of the heating sector by means of heat pumps and the transport system by means of electromobility .
The transition from conventional fuels to renewable energies is under way in many countries around the world . The concepts for the energy transition, as well as the technologies required for it, are known. From a technical point of view, a complete global energy transition is considered feasible by 2030. Political and practical problems, however, make implementation possible by 2050, with the lack of political will seen as the greatest hurdle. Both on a global level and for Germany, studies have come to the conclusion that the energy costs in a regenerative energy system are at the same level as in a conventional fossil-nuclear energy system or would be cheaper.
Denmark is a pioneer in the energy transition , and in 2012 it already covered 30% of its electricity needs with wind energy. Denmark is aiming for a completely renewable energy supply in all three sectors by 2050. The German energy transition, which has received approval and imitators around the world, but also criticism and rejection, is also important. Although it is often wrongly linked to the second nuclear phase-out in 2011, the energy transition in Germany began in the 1980s with the expansion of renewable energies and the search for alternatives to nuclear power plants . While there is consensus in science about the fundamental need to expand renewable energies, increase energy efficiency and save energy , the specific measures are often politically controversial. The public discussion often reduces the concept of the energy transition to the electricity sector, which in Germany only comprises around 20% of energy consumption . Likewise, in the political and public debate, it is often not taken into account that the success of the energy transition requires not only the expansion of renewable energies and an increase in energy efficiency but also behavioral changes in the sense of energy sufficiency. H. Saving energy by changing consumption habits.
history
History of the energy transition
Historically, long before the modern endeavors, decentralized and centralized approaches were proposed for a move away from fossil raw materials to (from a modern point of view) alternative sources of energy , propagated for various reasons . Research into earlier transformations of the energy system has gained in importance against the background of today's upheavals.
Finiteness of fossil fuels and foundations of climate science
Individual documents indicate that even before the industrial revolution, the finiteness of fossil raw materials was known or suspected in isolated cases. In Great Britain, for example, in the 16th century, fears had been raised that coal supplies would soon be exhausted. Therefore, export bans on coal were debated in parliaments and in Scotland in 1563 they were actually passed. However, until the 18th century, the view was widespread that coal reserves were inexhaustible. From the late 18th century onwards there were again several, e.g. Partly also publicly held debates about the finiteness of coal reserves and their range, whereby these debates also radiated from Great Britain to the continent. Most economists of the early 19th century, such as B. Adam Smith , not from permanent economic growth, but from a steady state that is permanently imposed by natural circumstances .
The contribution of the English economist William Stanley Jevons (1835–1882) finally became significant . While previously made forecasts about coal consumption either continued the current annual coal consumption in the future unchanged or continued the absolute increase in a linear fashion, Jevons was the first to formulate in a paper published in 1865 that coal consumption would increase exponentially , with the growth rate being 3 .5% annually. From this he concluded that this exponential growth after a certain number of years would lead to such enormous numbers that any finite source of raw material would be exhausted after a while, no matter how large the supplies actually were.
In Germany from the end of the 19th century there was also a major debate about a possible lack of energy, and there was also discussion about the earth's resource capacity. Among other things, the physicist Rudolf Clausius , for example, expressed his concern about the finiteness of coal reserves in particular in his 1885 book About the energy reserves of nature and their utilization for the benefit of mankind . Out of these considerations, he urged “a wise economy to be introduced” and warned “that what we find in the ground as the legacy of earlier epochs and that which cannot be replaced should not be wasted”. The faster a turn begins, the better it is for the future. The thesis of wasteful use of coal reserves was widely shared.
Max Weber saw the end of fossil fuels as synonymous with the end of the modern economic order. Werner Sombart , on the other hand, considered a continuation of civilization based on solar energy to be likely after the end of coal . In 1909, Nobel laureate in chemistry , Wilhelm Ostwald , spoke about the coal-based part of the energy industry and demanded that "the sustainable economy should be based exclusively on the regular use of annual radiation energy [from the sun] ". The coal behaves " like an unexpected inheritance [...] which induces the heir to temporarily disregard the principles of a sustainable economy and to live into the day ." Even a more economical use of coal could not prevent its inevitable exhaustion, but only delay it. Oswald Spengler, on the other hand, rejected the downfall of civilization due to a lack of coal per se. Basically, the problem of the finiteness of fossil fuels was already known in the 19th century, but this knowledge did not yet lead to concrete changes in behavior.
In 1912, the Italian chemist Giacomo Ciamician gave a lecture, which was later published in Science , in which he pointed out the advantages of the direct use of solar energy through artificial photosynthesis compared to burning coal. The use of solar energy in sunny but underdeveloped countries in the south could even out the north-south divide between the rich countries in the north and poor countries in the south and bring the latter to flourish economically. In addition, society would not be adversely affected by this fact in a future in which coal ran out, since civilization could last as long as the sun existed. He closed his lecture with the words that it would not be detrimental to progress and human happiness if the “black and nervous coal society” were to be replaced by a “calmer solar energy society”.
In addition, the basis for today's climate research was laid as early as the 19th century . The greenhouse effect of carbon dioxide was discovered by John Tyndall in the mid-19th century . In 1896, in a sensational publication , Svante Arrhenius not only pointed out the climatologically relevant influence of carbon dioxide in the earth's atmosphere for the first time, but also understood that the use of fossil fuels should only be of a temporary nature due to the associated global warming. He recognized the physico-chemical fundamentals relevant for global warming and at the same time the necessity of an energy transition, although global emissions of his time were less than a tenth of those of the beginning of the 21st century and the climate change caused by them seemed centuries away.
The fossil age: Renewable energies as a niche technology
With industrialization in the course of the 19th century, coal increasingly pushed the previously used regenerative energies (mainly biomass in the form of firewood and animal feed ) into a niche position. Nevertheless, as early as the 19th century - parallel to the expansion of the use of coal - various efforts were made to use solar energy as a source of power. So z. For example, the history of solar thermal power plants goes back to the second half of the 19th century, when inventors such as William Grylls Adams , Augustin Mouchot , Alessandro Battaglia or John Ericsson built various systems for solar energy conversion such as solar cookers , solar-powered distillers , refrigeration machines and boilers for solar-powered steam engines . A first solar thermal power plant was finally built in Egypt in 1913. Mouchot also succeeded in building an efficient solar oven in 1860 and later constructed a functioning solar steam engine, which, however, turned out to be too unwieldy for practical use. It was not until 1945 that the first commercial solar cookers were manufactured by the Indian pioneer Sri MK Ghosh.
The wind energy was converted to electricity generation much faster. Already at the end of the 19th century, only a few years after the construction of the first coal-fired power station, which was then known as the "power station", the first electricity-generating windmills were built. These tied in with the decentralized tradition of windmills , which were still widespread at the time, and of water mills , which were the most important commercial sources of power during industrialization well into the second half of the 19th century and even before the more expensive steam engines . In fact, the height of the water wheels and windmills used as mechanical sources of power is only dated by historians in Germany to the 1880s. These decentralized energy sources persisted in niches, for example regions with poor transport links, until the 1950s.
The electricity-generating windmills based on these mechanical predecessors finally experienced in the early 20th century, especially in rural areas, which lagged significantly behind cities in terms of electrification . T. relatively large distribution. Denmark was the pioneer, but the systems were also sold in the USA and Germany; By the 1930s, around 3,600 windmills were built in Germany, some of which were used as pumps and some to generate electricity. In Denmark, on the other hand, where Poul La Cour had been driving the use of wind energy in a theoretical and practical way since the late 19th century, wind turbines were already supplying around 3% of the electricity requirement in 1918. It should be noted here that the electricity supply was decentralized well into the 20th century ; it was only with the first large power plants in the second third of the 20th century that the balance shifted towards centralized energy supply.

In the 1920s and 30s, the technical and physical foundations of modern wind energy use were finally laid. In addition to the mass of decentralized small systems, large systems with a capacity of up to 20 MW were also considered. However, at the beginning of the Second World War , no prototypes were built of these systems, which are enormous even by today's standards . However, in 1941, the Smith-Pullman plant, a wind power plant with already 1.25 MW, went into operation in the USA, which was plagued by major technical problems, but remained in operation for four years. At the same time, there were plans in Germany during the Nazi regime to supply energy to so-called military farmers and the like. a. to cover decentralized with wind energy. The Ventimotor company involved in this, whose chief designer was Ulrich W. Hütter , who later made important contributions to the development of today's wind turbine technology, only installed six prototypes in Weimar. There was no longer any series production.
Research and wind turbine construction were also promoted in other countries. In the USA, prior to nationwide rural electrification, the focus was on building decentralized small systems that were used to charge accumulators . As a result, tens of thousands of small wind turbines with an output of 1.8-3 kW were installed from 1920 to 1960. After electrification, the trend was towards large-scale grid-connected systems. In 1941, a plant with 1.25 MW and a rotor diameter of 53.4 meters went into operation in Vermont, but series production of this and even larger successor plants was not carried out.
The beginning of the energy transition
Worldwide development
The perception of the environmental and energy crisis from the 1970s
The debate about the global ecological, economic and social problems that were triggered by industrialization , globalization and the energy system has been going on in science and society since the 1970s; in Germany it began in 1973 during the first oil crisis . Before that, in the 1950s and 1960s, energy policy was seen primarily from the point of view of economic efficiency. After the Second World War there was a relative drop in energy prices in Europe, which led to a historically unprecedented increase in energy consumption. Between 1950 and 1973, energy consumption increased by 4.5% annually, with petroleum playing a particularly decisive role, which became the most important energy source in this period. Between 1948 and 1972, the consumption of crude oil in Western Europe increased by a factor of 15. At the same time, energy consumption was perceived as a central indicator of economic prosperity, which led to great fears of economic decline, since energy consumption in Western Europe did not grow as quickly as in Eastern Europe. Until the first oil crisis, the energy policy of the industrialized countries was characterized by the energy syndrome described by Leon N. Lindberg ; which led to a system failure in the energy sector. Characteristic elements of the energy syndrome were:
- the need for a steadily increasing energy supply
- the absence of a comprehensive state energy policy with the parallel dominance of energy producers
- the blockade of alternatives through bureaucratism and industrialism
The beginning of modern energy research
This syndrome began to resolve in the 1970s. At the same time, research on energy issues was intensified, with social scientists increasingly taking up the topic of energy. With Human Ecology , Annual Review of Energy and Energy Policy , internationally important interdisciplinary scientific journals were created , which laid the basis for the institutionalization of energy research , and various subjects have now also taken up the field in universities . In the USA, against the backdrop of the oil crisis under President Jimmy Carter, an early movement emerged that aimed to transform the energy system and expand renewable energies . In 1976, the American physicist Amory Lovins coined the term Soft Energy Path , describing a path from a centralized energy system based on fossil and nuclear fuels to gradually moving forward with energy efficiency and renewable energy sources, and finally to replace them completely. A year later he published what is now considered to be the landmark book Soft Energy Paths. Toward a Durable Peace , which appeared at a time when the energy policy of many industrialized countries was dominated by the massive expansion of nuclear energy.
However, Lovins was not the first to develop a scenario for a fully regenerative energy supply. As early as 1975, the Danish physicist Bent Sørensen had proposed a plan for Denmark to switch exclusively to wind and solar energy in the journal Science , which could be implemented by 2050. Driven by the enormous oil dependence of the Danish state, which in 1972 imported 92% of its primary energy in the form of crude oil and was hit hard by the oil crisis in 1973 when crude oil prices tripled, Danish politics took up many proposals: As early as 1974, taxes on petrol , Diesel and heating oil increased; In 1985, when oil prices fell, another tax hike followed. In 1982 a tax on coal was introduced, and in 1992 the production of carbon dioxide was levied. Combined heat and power plants based on natural gas and biomass (including waste and straw) have been built and now supply a large part of the state's heat demand and part of the state's electricity demand. In 1981, a feed-in tariff for renewable energies was set, as a result of which Denmark became the most successful wind energy country in the world in terms of its share of the electricity supply and per capita. The nuclear power plant projects initially planned to diversify the primary energy base were abandoned after strong protests and finally in 1985 the possibility of building nuclear power plants was legally excluded.
Climate protection and sustainability become political goals
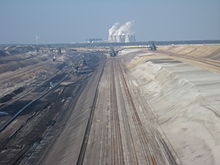
At the beginning of the 1990s, climate protection also became an important goal of global politics, after global warming had been predicted by science since the 1970s . In 1992, in Rio de Janeiro , the United Nations Conference on Environment and Development (UNCED) held to 154 countries in the Climate Change Convention of the United Nations committed themselves to dangerous interference with the climate system and to prevent the global warming to slow down and its consequences to mitigate. Other states later joined this convention. Other important results of UNCED were Agenda 21 , the Rio Declaration on Environment and Development , the “Forest Principles” and the Biodiversity Convention . Last but not least, the concept of sustainability was anchored in politics , even if its concrete implementation in political practice was only very limited ( see below ).
1997 was the Kyoto Protocol adopted, which entered into force in 2005 and the first time in international law binding targets for greenhouse gas emissions in industrialized countries commit. This protocol has been ratified by all states with the exception of the USA, but the goals set in it are considered to be less ambitious and inadequate for effective climate protection, especially since developing and emerging countries have no obligations.
Energy transition in research. From niche to mainstream
Also since the early 1990s, there has been an exponential growth in global scientific research on sustainable energy systems , both in relative and absolute terms . While the number of scientific publications on renewable energies was around 500 per year in 1992, in 2011 almost 9,000 new (English-language) publications were registered in the Web of Science . The most heavily researched area of research was solar energy.
In 1998 the first study on a largely regenerative energy system appeared, in which, for the first time, energy production and energy consumption were coordinated in two scenarios with 80 and 95% renewable energies, not only mathematically but also in the actual course of the year. After a series of similar work, Gregor Czisch presented in his dissertation in 2006 for the first time a work that demonstrated the feasibility of a completely renewable energy supply for the greater Europe and North Africa in hourly resolution. Between 2006 and 2009 Henrik Lund published several papers on a largely or completely regenerative energy supply for Denmark up to the year 2036 and at the same time analyzed what the mix of different regenerative energies should look like for the most expedient implementation of this goal. At the same time, further studies with similar objectives and results for different countries and regions of the world appeared worldwide.
A clear change in awareness took place around 2010. This year, nine very detailed papers on a complete regenerative energy supply for different countries of the world were published, whereby the feasibility of a complete or almost complete supply was for the first time not only assessed as realistic by specialist scientists but also by state advisory bodies and consulting firms such as PWC . In 2011, ten other similar studies followed, including a special report by the IPCC on renewable energies and the EU Commission's Energy Roadmap with a 97% scenario up to 2050. Also in 2011, Jacobson and Delucchi published a two-part paper in which they a Explain the scenario of a completely regenerative supply of the entire world economy, while Liu et al examined a 100% scenario for the People's Republic of China . In 2012 and 2013, the number of publications on similar scenarios increased further, demonstrating, among other things, the feasibility of very high renewable energies in various European countries (including Greece, Italy, Macedonia, Great Britain) as well as Australia, Japan and the USA.
The 2010s: Current Developments

A massive expansion of renewable energies is currently taking place in many industrialized and emerging countries ; however, the motivation in the individual states is of different nature. While in the industrialized countries the main focus is on the reduction of greenhouse gas emissions and the dependence on energy imports from politically unstable regions , all types of energy generation are being expanded in the emerging economies due to the strong economic growth, which in turn results in an increasing demand for electricity.
The V. a. However, the serious environmental damage associated with the operation of coal-fired power plants has led to a rethinking in China in recent years , which is expressed in stricter, state-imposed environmental protection measures and the promotion of renewable energies and energy efficiency. In 2013, China was the world market leader in the manufacture and use of wind turbines , solar cells and smart grid technologies; In addition, the state is both the largest investor in renewable energies and the world's most important green electricity producer.
Particularly after the "pollution shock" in 2013 and 2014, when hundreds of millions of Chinese suffered from heavy smog and fine dust pollution , and as a result of which air pollution was a major economic and social issue in the state, efforts were intensified and a series of measures aimed at making it more environmentally friendly Energy system initiated. Among other things, a plan was adopted to reduce particulate matter and smog pollution; In addition, a new construction ban for coal-fired power plants was issued in particularly heavily polluted regions and the introduction of the Euro 5 standard in the transport sector was set for 2015 in order to remove vehicles with particularly high levels of air pollution from the streets. Nationally, the Euro 5 standard will come into force in 2017. In addition, the share of coal in total energy consumption is to fall from 66.6% to below 50% by 2030, while the share of renewable energies is to be increased from just under 10% in 2012 to 25% in 2030. Although the measures were decided primarily to reduce environmental pollution, the achievement of these goals would also result in significantly lower greenhouse gas emissions.
In addition, saving fossil fuels plays a central role in the switch to renewable energies for many countries around the world, as this enables them to reduce energy imports and gain security of supply at the same time. At the same time, the risk of military conflicts over energy resources is reduced. The turning away from the "nuclear-fossil age" is seen as a reaction to a "multiple crisis" that is shaped by the nuclear disaster in Fukushima (which once again shows the vulnerability of nuclear energy generation after Chernobyl ), climate change, and food crises due to the production of agrofuels and speculation (see land competition and food price crisis 2007–2008 ), air pollution in large metropolises (see also transnational environmental pollution in East Asia ). This multiple crisis requires adjustments and solutions.
The transformation of the energy supply is advocated and supported by many institutions on a supranational level. The International Renewable Energy Organization IRENA was founded in 2010 to better coordinate the various paths . It sees itself as a “driving force” to promote the large-scale and increased use and sustainable use of renewable energies worldwide. In 2011 the United Nations founded the “ Sustainable Energy for All ” initiative . In December 2012, the General Assembly of the United Nations unanimously declared the 2014–2024 Decade to be the Decade for “Sustainable Energy for All”. In July 2014, UN Secretary General Ban Ki-moon published a report entitled Pathways to Deep Decarbonization , which, among other things, contains paths for sustainable development and decarbonization of twelve industrialized countries.
At the G7 summit at Schloss Elmau in 2015 , the G7 countries agreed to reduce global greenhouse gas emissions by 70% by 2050 and to completely decarbonise the global economy by 2100 . Subsequently, several states reported tightened climate targets. Among other things, the American President Barack Obama announced new guidelines aimed at reducing greenhouse gas emissions from American power plants by 32% by 2030 compared to the base year 2005. In the 2015 United Nations Climate Change Conference all agreed almost 200 UN member states in the Paris Convention on limiting global warming to well below 2 ° C. The aim is to achieve an increase of 1.5 ° C compared to the pre-industrial value; 1 ° C had already been reached at this point. The agreement entered into force on November 4, 2016. According to the 2017 United Nations report, the goal of achieving sustainable energy for all is being jeopardized by the world's population growth . The targeted, nationwide access to clean cooking facilities with electricity for 2030 threatens to fail. At the time of the UN report, more than three billion people were cooking with harmful fuels such as wood or dung.
Germany
The aim of the energy transition in Germany is to become largely greenhouse gas neutral by 2050. To this end, the federal government has set itself the goal of reducing greenhouse gas emissions in Germany by 40 percent by 2020, by 55 percent by 2030, by 70 percent by 2040 and by 80 to 95 percent by 2050 (each based on the base year 1990). This is to be achieved by expanding renewable energies and reducing primary energy consumption . As part of the nuclear phase-out, the last nuclear power plants in Germany are to be shut down in 2022.
Early phase

The origins of the German energy transition lie in both the environmental and anti-nuclear power movements of the 1970s. The effects of Lovins' "Soft Energy Path" were not limited to the English-speaking world. A German translation under the title “Sanfte Energie” came into bookshops in 1979, and it received a strong reception in the anti-nuclear movement, which had grown into an important political group by the mid-1970s. As a result, a scientific forecast by the authors Florentin Krause, Hartmut Bossel and Karl-Friedrich Müller-Reissmann on the complete abandonment of nuclear energy and energy from crude oil was published in 1980 by the Öko-Institut . She took up Lovins' theoretical considerations and applied them to German conditions. This work was entitled Energie-Wende. Growth and prosperity without oil and uranium , which is the first time the term energy transition was used. In the 1980s, the term was then picked up and propagated by various social currents. B. from the West German Greens , left-wing Social Democrats and the alternative press.
There was also a change in politics. With the entry of the Greens into the Bundestag in 1983, one party demanded an immediate nuclear phase-out . After the Chernobyl nuclear disaster , the SPD, which had previously been in favor of nuclear energy, as well as the trade unions joined the demand for a nuclear phase-out, whereby the SPD, in contrast to the Greens, committed itself to a nuclear phase-out after 10 years. Opponents of nuclear energy not only called for a nuclear phase-out, but also for a fundamentally new energy policy . While there have been a number of attempts by some SPD-ruled countries to shut down nuclear power plants, the conservative-liberal federal government maintained its nuclear energy-friendly course. However, the first funding measures for renewable energies were introduced as early as the late 1980s. A very important step for the energy transition in 1990 was the resolution of the Electricity Feed Act , which was introduced into the Bundestag by the two politicians Matthias Engelsberger (CSU) and Wolfgang Daniels (Greens) and which was approved by a large majority (CDU / CSU, SPD, Greens against FDP) was accepted.
Acceleration under red-green
The German energy transition experienced a significantly accelerated dynamic during the red-green federal government (1998–2005, Cabinet Schröder I and Cabinet Schröder II ). In the coalition agreement , a number of core elements of the energy transition were initially agreed with the introduction of the eco-tax on energy consumption, the 100,000 roofs program and, as a result, the introduction of the Renewable Energy Sources Act (EEG) , as well as the legally agreed nuclear phase-out , and finally by 2001 also implemented in applicable law. This was accompanied by a major change in the electricity mix . The share of renewable energies rose from 29 TWh in 1999 to 161 TWh in 2014, while electricity generation in nuclear power plants fell from 170 in 2000 to 97 TWh and coal electricity generation fell from 291 to 265 TWh. In addition, this coalition changed the perception of regenerative sources. While renewable energies were viewed under the previously ruling black-yellow coalition as a supplement to the existing power plant fleet, large parts of the red-green coalition viewed them as an alternative to the status quo, which would replace fossil-nuclear energy generation in the course of the 21st century should.
The current concept of the energy transition as the transition from fossil-nuclear energy sources to sustainable energy generation using renewable energies in its current form very likely dates back to the year 2002: On February 16, 2002, the specialist conference on energy transition - nuclear phase-out and climate protection took place in Berlin , organized by the German Federal Environment Ministry . At that time, the conservative and liberal side did not regard the energy transition as a worthwhile goal, but in the 2000s the basic resistance to the energy transition crumbled in the bourgeois parties as well, even though it was implemented in 2010 through the German extension of the term decided by the Merkel II cabinet Nuclear power plants was initially postponed into the future.
Term extension and second nuclear phase-out
This was revised with the nuclear disaster in Fukushima : On June 30, 2011, the Bundestag decided in a roll call with the votes of the CDU / CSU, SPD, FDP and the Greens the “13th Law amending the Atomic Energy Act ", which regulates the termination of the use of nuclear energy. In particular, the operating license for eight nuclear power units in Germany expired; the remaining nine blocks are staggered: the shutdown of the last nuclear power plants is scheduled for 2022. This de facto returned Germany to the status quo that had been agreed in 2000 under the Red-Green. Compared to the red-green nuclear phase-out, there were 8 more reactor operating years, and the year of the final nuclear phase-out remained the same at 2022.
All major German parties are now in favor of the energy transition, but there is still disagreement about the method of implementation and the speed of the process. This second nuclear phase-out received a lot of international attention, as a result of which the term energy transition or its translation became internationally known and has meanwhile found its way into the English language as Germanism .
Goals of the energy transition
Realization of a sustainable energy supply

The main goal of the energy transition is the realization of a sustainable energy supply in the three sectors of electricity, heat and mobility. According to Alfred Voss , sustainable development is understood to mean a way of life that makes it possible “ to satisfy the needs of people living now without impairing similar needs of people living in the future. [...] The preservation of the natural foundations of life, or in other words, the non-exceeding of the regenerative and assimilation capacity of the natural material cycles is therefore an essential condition for sustainable development . ”The definition of the term sustainability goes back to the Brundtland Commission , who made this definition 1987 coined and demanded an economic growth in order to solve the urgent environmental problems , in which " social and ecological aspects have to be spatially and temporally integrated into the economic consideration ".
According to a generally recognized definition , energy in a sustainable energy system should be " sufficiently and - according to human standards - permanently provided so that as many people as possible now and in the future have the chance for a decent life, and substances that cannot be recycled into the processes of change should be deposited in this way that the foundations of human existence are not destroyed now and in the future. “With the implementation of the concept of sustainability, an improvement in the sustainability triangle economy - society - ecology should be created and at the same time a global and intergenerational solidarity should be achieved. In the academic sustainability discourse, however, it is controversial to what extent the sustainability triangle with equally weighted sectors is an appropriate premise or whether ecological sustainability should not enjoy priority. Criticisms of the equal weighting are in particular the resulting difficulty in optimizing the overall system due to conflicting goals between the three individual aspects and the equal weighting itself, since the preservation of livelihoods through ecological sustainability is a basic requirement for social and economic sustainability and must therefore be prioritized.
According to Eichelbrönner and Henssen, future energy systems are characterized by nine different requirements . It should be noted that the order does not include any evaluation and that none of these requirements should be understood as an exclusion criterion. The basic requirements of future energy systems are therefore:
- Providing a sufficient amount of energy
- Demand-based quality of use and flexibility
- Energy security
- Resource conservation
- Inherent low risk and forgiveness
- Environmental sustainability
- International compatibility
- Social acceptability
- Low cost
Nuclear phase-out and climate protection
So far, the goal of the energy transition has been reduced to completing the nuclear phase-out and climate protection; In some cases, all three terms are even used similarly or synonymously . Even if both nuclear phase-out and climate protection are important sub-goals of the energy transition, reducing the energy transition to these aspects is a misleading shortening. So is z. For example, an exit from the use of nuclear energy by replacing it with fossil fuels is comparatively easy, without the need for a further system conversion. Climate protection, on the other hand, is in principle also possible by replacing today's fossil fuel power plants with nuclear power plants and, with some restrictions, also with fossil power plants with carbon dioxide separation. However, this path would not be feasible in the long term and would also involve great risks, which is why neither nuclear energy nor CCS technology are considered sustainable strategies for solving the current energy and environmental crisis. A switch to nuclear power plants and fossil power plants with CCS technology could avoid some of the environmental problems of today's energy system, but the fundamental problem of finite fossil and nuclear energy carriers would remain unsolved.
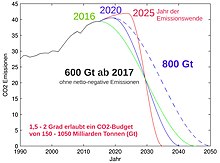
Since there is an approximately linear relationship between the cumulative total amount of greenhouse gases emitted and the resulting increase in temperature, the cumulative amount of greenhouse gases emitted must be limited (i.e. capped) for effective climate protection. Therefore, only some of the currently known fossil fuels may be used. According to data from the IPCC, a maximum of between 870 and 1,240 gigatons (billion tons) of carbon dioxide may therefore be released in the period from 2011 to 2050 if the two-degree target is to be achieved with a probability of more than 50%. Converted to the reserves, this means that in the global context around a third of the oil reserves, half of the natural gas reserves and more than 80% of the coal reserves must not be burned. Due to the imbalance between known reserves of fossil energy and carbon, which can still be burned, there is a risk of the so-called carbon bubble bursting , which would represent a major loss in value for energy companies in the fossil fuel business. At the same time, the exploration of new deposits that can no longer be exploited could prove to be a serious bad investment in the long term . The value of fossil energy reserves is estimated at approximately 27 trillion US dollars . A business-as-usual policy, on the other hand, would lead to the carbon budget for the two-degree target being reduced after 20 to 30 years, i.e. H. between 2035 and 2045, would be depleted.
In order to achieve the two-degree target internationally agreed in the Paris Agreement with a high degree of probability, global carbon dioxide emissions of around 40 billion tons per year must be halved every decade. Among other things, this requires a doubling of renewable energy generation every 5–7 years by 2050. On the other hand, coal combustion must be completely stopped between 2030 and 2035 and oil combustion between 2040 and 2045, and the use of natural gas must be greatly reduced by 2050, even if technologies for CO 2 capture and storage are available. Overall, the entire energy base of mankind must be fully decarbonized . To achieve this, humanity has only a window of a few years to decades in which to minimize the severe to catastrophic effects of climate change, which can last for thousands to tens of thousands of years.
Carl-Jochen Winter names the following necessary ecological criteria for the transformation to a sustainable energy system:
- the avoidance of a nuclear or climatic disaster
- the way away from the use of energy resources and towards conversion technologies
- the optimization of the metabolism system of mankind towards a minimal consumption of materials and closed material cycles
- the use of solar forms of energy
Social and ethical goals
Apart from technical and ecological criteria, future energy systems must also meet social and ethical criteria, as detailed above , in order to be considered sustainable. These include B. to find a solution for the current lack of distributive justice in fossil energy use, both with regard to distributive justice today (e.g. between the inhabitants of rich industrialized countries and poor developing countries) and intergenerational distributive justice .
Improving public health

Another goal of the energy transition is to improve public health. Around the world, around seven million people die prematurely each year from air pollution caused by short-lived climate-relevant pollutants such as methane , soot and ozone , which lead to respiratory and circulatory diseases . This effect will be intensified in the future by climate change and the heat island effect, especially in cities. In addition, air pollution increases illnesses such as asthma and cancer , increases the number of stays in hospitals and intensive medical care, and increases the number of days absent from work and school children due to illness, which in turn has economic and social consequences for society and the economy. Air pollution is mainly caused by the combustion of solid, liquid and gaseous fuels, which is why the solution to this problem can only be achieved through a fundamental change in the energy system.
A 2015 review article in The Lancet concluded that climate change has the potential to reverse health advances through economic development. However, combating it could be the 21st century's greatest opportunity for improving public health around the world. Many climate protection measures would also help directly against damage to health, poverty and global inequality , which would enable states to strengthen public health and the well-being of the population and, last but not least, to reduce health care costs.
The main source of air pollution in industrialized countries is the burning of coal in power plants and oil in the heating and transport sectors , while the use of natural gas hardly contributes to air pollution. Another important source is the combustion of solid and liquid biomass such as B. wood or biofuels . Open hearths in buildings are particularly problematic , as they are mostly used with wood in most developing countries. Around 2.8 billion people worldwide (41% of the world's population ) use such stoves without a smoke vent or chimney . The number of annual deaths from this use is estimated at around 1.4 million.
It is almost certain that a large number of health and environmental problems could be greatly weakened or eliminated with the widespread use of renewable WWS energies (wind, water and solar energy). In addition to the use of renewable energies, the electrification of the energy system with electric vehicles would also help to reduce air pollution. By halving emissions of air pollutants by 2030, Schmale et al. Avoid approximately 40 million premature deaths by 2040. However, this would require much more effort than before; the measures implemented so far would only avoid around 2 million deaths. In addition, avoiding damage to health can reduce the costs of an ambitious climate policy, e.g. T. clearly overcompensate. Research for the USA has shown that the economic health benefits resulting from the replacement of fossil energy exceeded the wind energy subsidies in the form of the Production Tax Credits by approx. 60%. In addition, the health benefits through reduced air pollution, in contrast to climate protection, which has a global and only long-term effect, have a local and short-term effect.
Combating energy poverty in developing countries

Access to energy is currently very unevenly distributed around the world. This goes hand in hand with energy poverty among broad sections of the population that is rampant in many developing countries, which is further intensified by the growth of emerging countries and the associated increase in global demand. With the accompanying rise in the price of fossil fuels, which are usually traded in hard currencies such as the US dollar, developing countries with only limited foreign currency reserves are making access to energy sources, especially oil , increasingly difficult and poverty is further exacerbated.
For example, around 2010 the G8 nations , which make up 12% of the world's population, were responsible for around 50% of the world's primary energy consumption , while the poorest 25% of the world's population only account for 3% of primary energy consumption. This is accompanied by other social problems: Low access to energy correlates with poor access to food resources , high child mortality and low life expectancy . However, if the basic energy requirements are met, which is given from an annual per capita primary energy consumption of approx. 2.6 tons of oil equivalent , additional energy consumption does not result in any further improvement in these values. It is therefore an important goal of development policy to supply 1.2 billion people with electricity and 2.8 billion people with reliable and clean energy for cooking purposes .
Intergenerational justice
Distributive justice between individual generations is also of great importance. The use of fossil fuels by current or past generations affects two aspects of intergenerational justice : On the one hand, future generations can no longer use resources that were consumed by previous generations and are therefore restricted in their right to development in the sense of strong sustainability . On the other hand, they are negatively affected by climate change as a result of the burning of fossil fuels, as they have to cope with negative climatic conditions that they did not cause themselves. The current generation, on the other hand, benefits because they do not have to pay for the ecological and economic consequences of their use, but can transfer the solution to the problems they cause to the next generation. Generational justice, on the other hand, presupposes that each generation must be able to freely decide how it wants to produce and distribute goods. However, this freedom of future generations is inadmissibly severely restricted by the current generation through actions that cannot be reversed promptly; for example through human-made climate change and its consequences, the overexploitation of raw materials and (fossil) energy sources or the destruction of animal and plant species . The main difficulty of this conflict is the fact that future generations cannot participate in coping with it, but that this task can only be carried out by the state or its institutions.
Other aspects
In addition, a number of other aspects for the implementation of the energy transition are mentioned in the scientific, public and political discourse; An expert survey on the German energy transition revealed a total of 14 different goals. These goals include a. political, social, economic or ecological nature; some examples are listed in the following in note form.
- Democratization of production and distribution structures, feasible e.g. B. in the form of energy cooperatives or energy autonomous regions
- Reduction of the economic risks of an energy shortage or an energy crisis (e.g. oil crisis ) through practically unlimited primary energy
- Avoidance of military conflicts over energy resources
- Economic advantages through a long-term cheaper energy supply
- Economic added value through the production and export of climate protection technologies
- Creation of new jobs because the use of regenerative energies is more labor-intensive than conventional energy generation
- Increasing domestic added value by reducing energy imports
- Reduction of water consumption by reducing the cooling water consumption of conventional power plants (currently in Germany about 0.9 to 1.33 m³ / MWh for coal-fired power plants and 1.44 to 2.12 m³ / MWh for nuclear power plants)
- Energy saving through lower power plant own requirements of regenerative compared to fossil power plants
Motivation for the transformation

The background and motivation of the energy turnaround are the increasing ecological and social problems associated with the use of fossil and nuclear energy sources. A number of negative side effects are associated with energy consumption by industry and end consumers , the consequences of which have been increasingly becoming a part of social and political awareness since the 1970s. These include u. a. the emission of the greenhouse gas carbon dioxide, air, land and water pollution , the production of radioactive waste , geopolitical conflicts over resources, the shortage of energy sources and rising food prices. Other important reasons for switching to a regenerative energy supply are to ensure (long-term) energy security , the health risks from burning fossil fuels and socio-economic aspects such as B. the democratization of the energy supply, the expansion of citizen participation and the creation of jobs.
Until the beginning of the 1970s, security of supply and the price of energy were in the foreground, but from the 1970s onwards the perspective changed. With the oil crises , the debate on the use of nuclear energy and the environmental debate, there were fierce social disputes over energy, environmental and technology policy in many countries and, as a result, fundamental changes in the reality of energy policy and the energy industry. The study The Limits to Growth , published in 1971/72 by the Club of Rome , was also important here , the forecasts of which suddenly became acute with the first oil crisis in 1973. Today, the environmental impact of burning fossil fuels is particularly important. This manifests itself on the one hand in environmental damage from air pollutants , which at the same time cause diseases such as skin and respiratory diseases, allergies and cancer and result in high economic costs, but in particular through the emission of greenhouse gases and the associated global warming .
In order to achieve the goal of a more sustainable energy supply, the move away from the fossil-nuclear energy system is propagated and a transition to a new “solar age” is called for. Solar forms of energy play the role of backstop technology . This change is justified - in addition to a number of other positive effects - mostly with the fact that renewable energy sources have fewer negative environmental and climate effects than the conventional energy industry. In addition to the much lower greenhouse gas emissions from renewable energy sources, switching to technologies such as wind power and solar systems can also significantly reduce environmental pollution such as water pollution , eutrophication and fine dust emissions. Although the material requirements for these technologies are higher than for the construction of conventional power plants, the environmental impact due to the higher material requirements is low compared to the direct emissions from fossil-fired power plants. In parallel, the mining of fossil fuels such as. B. in crude oil extraction , natural gas extraction , hard coal and lignite mining or when extracting uranium occurring environmental damage or pollution is significantly reduced or even avoided.
Global warming
Global warming and its consequences are one of the greatest challenges of the 21st century and beyond. The main cause of global warming is humans, which is why climate protection is the most important aspect for the restructuring of the energy supply today. If the current emission level for greenhouse gases is extrapolated according to the Fifth Assessment Report of the IPCC by 2100, a probable global temperature increase between 3.7 and 4.8 ° C (uncertainty range: 2.5–7.8 ° C) compared to the pre-industrial level can be expected. If climate change is to be limited to a tolerable level, the global use of fossil fuels must be greatly reduced.
The most important driver of global warming is the release of greenhouse gases through the burning of fossil fuels. About 80% of the anthropogenic, i.e. H. Man-made greenhouse gas emissions are attributable to energy use. The greenhouse effect was discovered as early as the 19th century; In the meantime, the theoretical predictions on the effect of the greenhouse effect have been confirmed experimentally by modern long-term studies directly in nature. With the climate change caused by greenhouse gases, there are various negative secondary effects such as the melting of the ice caps , the rise in sea level , changes in the water cycle , the frequent occurrence of climate extremes and unpredictable effects on biodiversity , but also the acidification of the atmosphere caused by a higher carbon dioxide content Seas . Global warming is also accelerating the extinction of species. If no measures are taken to combat climate change, 16% of all species worldwide are threatened with extinction, according to a review study published in Science in 2015. The individual values on which this work is based assumed extinction rates of up to 54%. If the two-degree target is met, this rate could be reduced to 5.2%.
The most important anthropogenic greenhouse gas is carbon dioxide , which is mainly released in large quantities when fossil fuels are burned. It is true that a great deal of carbon dioxide is released through natural processes, for example through the breakdown of biomass , but this is offset by an equally large amount of natural fixation by plants; the material cycle is closed. By burning fossil fuels and other human interventions such as B. the clearing of forests , however, additional carbon dioxide is released into the atmosphere, which increases the proportion in the atmosphere. A large part of the carbon dioxide released by human activities remains in the atmosphere for tens of thousands to hundreds of thousands of years, thereby reducing the climatic effects of carbon dioxide emissions such as carbon dioxide emissions. B. the sea level rise not only over a few decades or centuries, but over geological time .
In addition to carbon dioxide as a combustion product, the energy industry is also responsible for the emission of large amounts of methane . Methane is the second most important greenhouse gas. It escapes from the seams during coal mining as well as from the wells during oil production and is also released during natural gas transport. Around 30% of methane emissions in Germany come from the energy industry.
Conventional energy sources are finite
The finite nature of fossil-nuclear energy carriers , which are only available for a limited time (depending on the energy carrier, a few decades to centuries), also plays a central role in the transformation of the energy system from an energy management perspective . Regardless of other aspects such as climate change, a transition to other types of energy supply will inevitably be necessary in the long term. In addition to their use as energy sources, fossil raw materials, above all crude oil , are also very important basic materials for use in petrochemicals and the starting point for a large number of products, which must also be taken into account when considering the finite nature of these resources.
The irretrievable depletion of non-renewable resources (such as fossil fuels) is a problem that has not yet been solved in economic theory . Fossil fuels are based on solar energy that has been stored over the course of millions of years. These reserves are used up with use, so that the industrial-fossil energy system cannot be a permanent system, but rather represents a “phenomenon of transition”.
According to the English economic historian Edward Anthony Wrigley , humanity is therefore in a phase in which new solutions must be found. Access to fossil fuels has brought unprecedented prosperity to three continents and is rapidly changing two more. Since these are consumer goods, they would be depleted. Although the extent of the resources in coal, oil and gas is the subject of many studies and remains unclear for the time being, it is unlikely that they will last longer than two to three generations to meet future energy needs, especially if this continues to rise. Continuous dependence on fossil fuels would therefore lead to a catastrophe.
The finiteness of fossil fuels is also closely linked to long-term energy security , since fossil fuels are more difficult to find and mine and their prices are rising. Energy insecurity as well as the rising price trend for fossil fuels are considered to be a major threat to the political and economic stability of states. Historically, the OPEC countries in particular showed during the oil crisis that energy resources can also be misused as a means of political power; Nowadays, Russia in particular is seen as a state that could abuse its market power in the field of fossil fuels for geopolitical goals. This is why the EU is striving for greater independence from exporters of fossil fuels and uranium exporters for economic, political and geopolitical reasons.
For example, according to the Federal Institute for Geosciences and Natural Resources in Germany, the net import dependency in 2013 was almost 100% for nuclear energy, 98% for mineral oil , 88% for natural gases and 87.0% for hard coal . The costs for these energy imports amounted to 99.4 billion euros, about 70% of the total raw material import costs. Russia supplied 34.8% of the oil, 34.1% of the natural gas and 24.8% of the hard coal imports. In 2015, renewable energies were the most important domestic energy source with a share of 40.9% of domestic primary energy generation, followed by lignite with 39.4% and by a large margin ahead of natural gas with 6.6%. All in all, Germany imports fossil fuels each year with around 2800 TWh, for which around 90 billion euros have to be spent. In the period 2000–2013 Germany spent a net 833 billion euros on energy imports; the EU countries import energy carriers worth around 350 billion euros every year.
Nuclear energy problem

In principle, according to the current state of science, only renewable energies or nuclear energy including nuclear fusion are able to meet mankind's energy needs in the long term. In the case of nuclear energy, however, it must be taken into account that there are currently neither nuclear fusion power plants nor breeder reactors , which would be necessary for a long-term secure fuel supply for nuclear fission power plants. Breeder reactors are technically very difficult to control; With one exception, all previously built breeder reactors were shut down after technical incidents. Commercial nuclear fusion power plants, on the other hand, will not be deemed operational until 2050, which would be too late to solve the current problems (particularly global warming).
The question of the final disposal of spent fissile material and the endangerment of the population in the event of accidents in nuclear power plants are associated with nuclear energy generation , while the chances of using nuclear fusion from both a technical and an economic point of view (high electricity production costs) are questionable. Moving away from nuclear energy could also exclude its risks. These include B. the environmental pollution during the mining of uranium ores, the transport and (final) storage of radioactive waste , as well as the risk of core meltdowns with uncontrolled release of radioactive material such. B. in the reactor disasters of Chernobyl and Fukushima . In addition, nuclear energy is characterized by a number of great uncertainties and unsolved problems and dangers with regard to health, environmental compatibility, sustainability, social stability and international relations. It is therefore the state of research that in the medium to long term “new concepts for a safe and sustainable energy supply must be found”.
Due to the growth in world energy demand with a simultaneous widespread stagnation of nuclear energy, its share in world energy production decreases annually. After strong growth in the 1970s and 1980s, which led to a total output of 330 GW in 1990, the global output of nuclear energy has only increased slowly since 1990 to 376 GW in 2010, while the share of electricity generation from 18 % decreased in 1993 to 13.5% in 2009. Political changes in several states after the Fukushima disaster led to a decline in electricity production. In 2013, nuclear energy supplied 2.359 TWh of electrical energy, 10.8% of the world's electricity demand. In 2008, nuclear power plants around the world had fed in 2,731 TWh and thus covered 14% of the world's electricity demand. In relation to global final energy consumption , the share of nuclear energy is comparatively low; in 2008 it was 2.3%. The reasons for the low growth were rising costs, energy-saving measures, the limited use of fuel, the accidents at Three Mile Island and Chernobyl, criticism of the environmental movement, risks from the proliferation of nuclear material and from terrorism , technical and economic risks from the dismantling of old nuclear power plants and above all the still unsolved final disposal over a period of tens of thousands to hundreds of thousands of years.
Because of its comparatively low carbon dioxide emissions, nuclear energy is touted by proponents as a means of combating climate change, while opponents reject it because of the risks mentioned above. At 9–70 g CO 2 / kWh, the CO 2 emissions from nuclear power plants are higher than from wind power, solar thermal and hydropower plants, but at a similar level to photovoltaic systems and significantly lower than from all fossil power plants including coal-fired power plants with CO 2 - Deposition and storage . Under the hypothetical assumption that for the purpose of decarbonising the energy system, the entire expected energy demand of the earth would be covered by nuclear energy by 2030, around 15,800 reactors with a capacity of 850 MW each would have to be built worldwide. On the other hand, if only 5% of the world's energy needs are supplied by nuclear energy, the number of reactors would have to be doubled compared to 2010.
After the Fukushima disaster, several states accelerated the shutdown of their oldest nuclear power plants, while others review existing expansion plans. Due to the large amount of capital required, long construction times and an anti-nuclear mood in many countries, it is unlikely that nuclear energy can make any significant contribution to climate protection.
concept
From a purely technical point of view, a complete global energy transition would be possible by around 2030. For practical, economic and political reasons, however, a longer period is necessary, with implementation by 2050 being considered possible. The expansion of fossil and nuclear energies is to be stopped by 2030 and then gradually the switch to a regenerative energy system will take place by 2050. The main argument against this scenario of a rapid energy transition, which is described as an enormous challenge, is the lack of political will to actually want to achieve this goal. The later the conversion of the energy supply is started, the more expensive it is and the more necessary it becomes to use high-risk technologies. Foregoing climate protection, which would lead to global warming of 4 ° C and more by the end of the 21st century, is in turn associated with risks that cannot be assessed .
However, the specific design of the energy transition is still controversial. Central questions here include: a .:
- Which conventional energies should be used until a full regenerative supply is achieved?
- Which renewable energies should the focus be on and how can individual technologies complement each other?
- What is the storage requirement? (Also depending on the choice of regenerative techniques chosen)
- Should the energy transition be decentralized or centralized?
- Which political concepts are decisive for the implementation of the energy transition? Should the focus be local, national or international?
- Which actors can force and accelerate the energy transition? Which actors are interested in slowing down?
- What is the role of companies in the conventional energy sector?
- Can the energy transition be implemented quickly enough to be able to cope with the imminent problems of the conventional energy system in good time? How can the development towards a sustainable energy supply be accelerated?
Core elements
The core elements of the energy transition are the expansion of renewable energies , the increase in energy efficiency and the saving of unnecessary consumption . In this way, today's economy, which is largely based on the combustion of fossil fuels, is to be transformed. The phase-out of coal and the phase-out of burning oil and gas with the aim of decarbonising the economy are therefore central elements of the energy transition , along with the much better known nuclear phase-out . While the expansion of renewable energies, especially hydropower and wind energy, but increasingly also solar energy, has already led to significant increases in the work performed worldwide, it is precisely the increase in energy efficiency and energy saving that have been neglected aspects of the energy transition.
Various key technologies are necessary for the implementation of the energy transition. These include the power sector in particular wind and solar energy , the transport sector electric cars and in the heating sector energy savings , heat pump heating , remote - and district heating systems and large heat storage . Other important technologies are biomass gasification and systems for the separation and use of carbon dioxide , for water electrolysis and for the production and storage of electric fuels .
Renewable energy
Renewable energies are energy sources that are practically inexhaustible according to human time standards. In addition, secondary energy sources such as electricity, heat and fuel obtained from renewable energies are often imprecisely referred to as renewable energies. Energy carriers are solar energy including its indirect forms such as B. wind energy, geothermal energy , currents and tidal forces . Important technologies for their use are photovoltaic systems and solar collectors , wind turbines , hydropower plants , as well as systems for the use of bioenergy and geothermal energy. The most important energy suppliers in a largely or completely regenerative energy system are wind and solar energy. Other renewable energies such as hydropower and geothermal energy can only play the dominant role in some countries with particularly suitable site conditions.
Due to the scarcity of conventional energy sources and the environmental problems caused by their emissions, efforts are being made worldwide to increase the share of renewable energies in the energy mix. Renewable energies have significantly lower specific pollutant and greenhouse gas emissions than conventional power plants . The mean carbon dioxide equivalent of wind power plants per kilowatt hour is 9.4 g CO 2 , for hydropower plants 11.6 g CO 2 , for photovoltaic systems 29.2 g CO 2 , for solar thermal power plants 30.9 g CO 2 and for geothermal power plants 33.6 g CO 2 , while combined cycle gas power plants emit approx. 350 to 400 g CO 2 and hard coal power plants around 750 to 1050 g CO 2 per kWh. When it comes to emission values for renewable energies, it must be taken into account that these are current values that reflect the current energy mix. However, as the energy transition advances, emissions will automatically decrease, as the majority of the emissions are caused by burning fossil fuels during the manufacture of the systems.
In 2015, renewable energies (RE) covered 19.3% of the world's final energy demand . In the electricity sector , renewable energies provided 24.5% of the electrical energy produced worldwide in 2016. There are expansion targets for renewable energies in at least 176 countries around the world, and in a large number of these countries there are also various funding measures for their dissemination.
Energy efficiency

Energy efficiency is understood to mean the more rational use of energy. Optimized processes are intended to minimize “the quantitative and qualitative losses that arise in the individual conversion, transport and storage of energy” “in order to achieve a given (energetic) benefit with decreasing primary or final energy use”. Thanks to technical improvements, more efficient devices with the same service life and the same usage behavior result in energy savings compared to less efficient devices. Increases in efficiency are possible, for example, by improving the efficiency of household appliances, (car) engines, better power plant technology or better thermal insulation of houses. Even with the lighting efficiency lamps can be achieved by use of very simple energy savings. While ordinary incandescent lamps only have an efficiency of around 2.2% (15 lumens / watt ), compact fluorescent lamps have around 70 lumens / watt. LED lamps with 100 lumens / watt, which are also free of mercury and also have a very long service life of up to 50,000 to 100,000 hours, are even more economical .
There is great potential for increasing efficiency, especially when it comes to heating requirements in the building sector. Buildings are responsible for around 40% of primary energy consumption worldwide and cause around a third of carbon dioxide emissions. In Central European countries like Germany, heating accounts for around 80% of total energy consumption in private households ; DHW heating accounts for 12% and electrical energy for 8% . It is assumed that an increase in the building renovation rate can halve the heating requirement within around 30 years. At the current European renovation rate of 1.4% per year, energy consumption in the building sector would be reduced by around 40% between 2005 and 2050. With an increase to 2%, energy savings of 74% would be possible. In Germany about 90% of the existing buildings are poorly insulated. Particularly large savings can be achieved in new buildings, where low energy house or passive house standards allow large energy savings compared to the current building stock. Passive houses only need about 5% of the energy of an existing building on average. The best passive houses achieve consumption values of 10 to 15 kWh per m² and year, while the building stock averages 220 kWh per m² and year. Low-energy buildings are in between with around 70 kWh per m² and year. Plus-energy houses , on the other hand, deliver more energy per year (in terms of balance sheet) than they need in the same period (for example through very good insulation and the installation of a photovoltaic system ). How high the energy requirement per square meter of energy reference area may be per year is specified in the so-called energy standard.
The so-called rebound effect often occurs after energy efficiency measures have been implemented , i.e. increased use of the technology, which reduces or even eliminates the energy-saving effect of the efficiency measures. The literature assumes that the energy savings resulting from the efficiency measure are on average 10% lower, with the values of individual studies fluctuating between 0 and 30%.
Energy saving

While energy efficiency measures usually require investments, energy saving or energy avoidance are measures that are achieved through individual behavioral changes and can therefore be implemented immediately and at the same time do not incur any costs. These include B. the waiver of the use of unnecessary functions, such as a standby function of a household appliance . Energy savings are e.g. B. possible through lowering the room temperature, less use of automobiles, especially on short journeys, an energy-saving driving style or vehicles with less fuel consumption ( three-liter car instead of 15-liter SUV ) or the conscious use of heating and lighting.
Also effective is z. B. Timely and needs-based heating so that only rooms actually used are heated, as well as effective ventilation (i.e. intermittent ventilation instead of continuous ventilation). In addition, by lowering the room temperature by 1 ° C, about 5% of the heating energy can be saved. In addition to behavior-related measures, energy savings can also be achieved through organizational measures. This includes the (better) maintenance of devices and vehicles, e.g. B. by optimizing the engine setting and air pressure in tires, the waiver of the air resistance increasing structures such. B. roof racks , the removal of unneeded goods from vehicles to save weight and the increased use of rail and public transport instead of the road.
The specific usage behavior has a z. T. very large influence on the energy consumption of a good. In the building sector z. For example, the energy consumption of two identical houses, depending on the respective behavior of its residents with an identical construction, decreases by approx. 35% from the mean. Conscious behavior can significantly reduce the energy consumption of a house, while counterproductive actions such as B. incorrect ventilation can cause significant additional consumption.
Sector coupling and electrification of the energy system
For an ecologically sustainable and affordable energy system, synergy effects of a stronger coupling of the sectors are considered necessary, i.e. extensive electrification of the heating and transport sector , because the most important renewable energy technologies, solar and wind energy, primarily provide electricity, which is also very much via electricity networks can be easily distributed. The sector coupling also offers great potential for energy savings compared to fossil energy systems, whereby the effects actually achieved depend heavily on the correct design of this electrification. Heat pump heating systems are more efficient than electrical resistance heating systems. In addition to saving energy, the electrification of the heating and transport sector is also beneficial from an ecological and health point of view, since heat pump heating systems and electric vehicles do not release any exhaust gases and thus no pollutants such as soot , fine dust or nitrogen oxides at the point of use . For example, especially when using green electricity, electric vehicles can help to reduce the environmental and health pollution caused by the transport system and at the same time improve air quality .
The increased use of combined heat and power also creates a stronger network of the electricity and heating sectors. On the one hand, due to this expanded demand, a higher electricity consumption can be expected in the future energy system than today, while the primary energy requirement would decrease due to the use of regenerative sources and the resulting increased energy efficiency in electricity generation. Jacobson and Delucchi point out that in a completely regenerative energy system by switching from combustion engines to electric motors in the transport sector, very clear efficiency advantages could be achieved, while the generation of hydrogen from excess electricity would result in additional losses compared to the status quo. Overall, they come to the conclusion that 30% of energy consumption can be saved in a regenerative energy system compared to a conventional energy system. Mathiesen et al. determined in three different energy transition scenarios for Denmark in each case about a halving of the primary energy requirement compared to a largely fossil reference scenario.
Heating sector
In the heating sector, district heating systems and heat pump heating are seen as the most promising heating options.
Heat pumps
Of all the individual technologies currently available on the market, heat pump heating is considered to be the one that could possibly make the greatest contribution to global greenhouse gas reduction. The IEA assumes that the use of heat pumps alone can reduce global greenhouse gas emissions by 8% annually if 30% of the buildings are heated with heat pumps instead of fossil-fueled heating systems. According to Valentin Crastan , heat pump heating systems are considered to be "by far the best heating" in terms of sustainability . Heat pump heating systems offer great potential for increasing efficiency in the heating sector, which they can only fully exploit if the electrical energy required for their operation is obtained from renewable energy sources. Another advantage of them is their significantly higher efficiency compared to systems based on direct electrical heating, such as B. the case with the simpler, but also cheaper to purchase electrode boilers .
With the large-scale expansion of geothermal heat pumps and the parallel decarbonization of the energy supply, around 60% of the primary energy and 90% of greenhouse gas emissions in the heating sector could be saved in the EU in 2050 compared to gas heating. At the same time, heat pumps can improve the integration of variable renewable energies into the energy supply system by storing temporary excess electricity as thermal energy. For this purpose, heat storage systems integrated in the heat pump heating system as well as the heated building itself can be used. The use of future green electricity surpluses to operate heat pumps ( power-to-heat ) has the greatest environmental benefit of all power-to-X concepts in terms of greenhouse gas reduction and saving fossil fuels. At the same time, the coupling of electricity and the heating sector is considered to be particularly promising because energy conversion and heat storage are possible at comparatively low costs.
It should be noted that the energy efficiency of heat pumps depends on the technology used, e.g. T. can clearly differentiate. Air-water heat pumps, which remove the heat energy from the ambient temperature, have, in particular at cold outside temperatures, the lowest coefficients of performance and thus also come to lower annual coefficient as a heat pump with heat sources. Geothermal heat pumps, on the other hand, work independently of the outside temperature and can achieve annual performance factors between 3 and 5, i.e. H. provide 3 to 5 kWh of heat using one kWh of electrical energy; the highest value given in the literature is 5.2-5.9. The efficiency of geothermal heat pumps can also be increased by coupling them with thermal solar collectors .
On the other hand, because of their inefficiency, electrical building heating systems such as night storage heaters or radiators , in which the electricity is converted directly into heat by means of heating resistors, are unsuitable for the energy transition. Compared to fossil-fired building heating systems, these heating systems have a significantly higher primary energy consumption. Comes from z. B. the electrical energy required to operate such a heater from a coal-fired power plant, then the primary energy consumption is 2.4 times that of a conventional fossil-fueled heater. With completely regenerative power generation, for example from hydropower plants, the primary energy consumption is the same as with fossil heating, but also significantly higher than with heat pump heating.
Sustainable district heating systems
In addition, the district heating supply is seen as an important pillar of a renewable energy system, especially in densely populated urban regions. There is a special focus on district heating systems of the fourth generation , which are specially designed for the requirements of a renewable energy system. Renewable energies such as geothermal energy, solar thermal energy ( e.g. in the form of solar district heating ) or previously unused waste heat from industrial processes should serve as the heat source for this system . In addition to (biomass-fired) CHP systems, large heat pumps, among other things, are to play an important role, resulting in a strong link with the electricity sector. By combining electricity and heat supplying CHP systems, heat pumps and heat storage , district heating systems of the 4th generation should also offer a lot of flexibility for energy systems with a high proportion of variable renewable energies such as wind energy and solar energy and thus compensate for their fluctuating energy supply; For example, by operating the heat pumps when there is a surplus of green electricity or, alternatively, the CHP systems when the green electricity is not produced to meet demand.
Be particularly advantageous for the integration of high shares of renewable energy at the same time very high overall efficiency is the combination of cogeneration and other in cogeneration considered fired power plants with heat pumps and heat storage. In such a system, heat pumps would cover the heat demand during periods of high electricity production from wind and / or solar energy and at the same time utilize any excess electricity, while the CHPs could remain switched off. With only low electricity production from renewable energies, however, the CHPs would provide both electricity and heat. By integrating heat storage systems into such a system, electricity and heat production can also be decoupled from one another, so that any losses due to heat from the CHPs that are temporarily not required are minimized. In the district heating system, too, the use of heat pumps in district heating systems is considered to be one of the most promising ways to increase the energy efficiency of district heating networks and to achieve climate protection goals, not least because heat pumps operate emission-free when using green electricity. At the same time, large heat pumps allow low-temperature sources such as environmental heat or industrial waste heat to be used to a large extent for heating purposes.
Transport sector

Since the energy turnaround without reconstruction of the transport sector can not be played by traffic turning an important role for the success of the energy transition. Therefore, the expansion of electromobility in the form of electric cars , pedelecs , electric trucks and the expansion and conversion of local public transport z. B. an important function with battery buses . It is believed that electricity stored in a future regenerative energy system will be the most efficient fuel in the transportation sector . The expansion of electromobility is intended to reduce oil consumption and carbon dioxide emissions in particular, thereby making transport more sustainable overall. By using electric vehicles compared to the vehicles with internal combustion engines that have been used up to now, which have only a low degree of efficiency, the energy consumption of the transport system could be reduced significantly, but only if the electricity is produced using renewable energies.
Despite the higher energy consumption for the production of the batteries , electric cars perform better than vehicles with internal combustion engines when considering their entire life cycle, both in terms of fuel consumption and greenhouse gas emissions . Only under the assumption that electricity from coal-fired power stations is used to operate the electric vehicle and that the batteries are also manufactured in a technologically less advanced factory, the greenhouse gas balance of electric cars was higher than that of vehicles with combustion engines. However, if the total environmental damage caused by pollutant emissions etc. is considered, the environmental balance of an electric vehicle is more favorable than that of a fossil-fueled vehicle even when using coal-fired electricity, even if all other types of electricity generation have significantly lower environmental effects. Using the average European electricity mix of 2009, battery electric vehicles emit 44 to 56% or 31 to 46% less CO 2 than vehicles with internal combustion engines, depending on the approach used (simplified well-to-wheel analysis or full life cycle analysis) .
However, chemical fuels would be required for significantly longer in areas where electrification is more difficult to achieve. These include B. air traffic , heavy goods vehicle traffic and ship transport . In addition to the use of biomass, the use of synthetic fuels such as methanol , dimethyl ether or methane , which were previously produced from renewable electricity using power-to-liquid or power-to-gas technologies, can also be used for this. Another possibility is the use of fuel cell vehicles , which, however, have a poorer energy balance compared to battery electric cars with the concepts used so far. The energy requirement of fuel cell vehicles that are operated with regenerative electrolysis hydrogen is lower than that of vehicles with internal combustion engines, but it is also around 130% higher than that of electric vehicles, which means that they require more than twice as much energy as electric cars.
The increased use of electric trains instead of individual means of transport can also increase the energy efficiency of the transport sector and at the same time promote the necessary electrification. That is why changes in behavior are also important, such as buying lighter and weaker motorized automobiles or restricting air travel, implementing new, smoother mobility concepts and using electrified local means of transport, e.g. B. battery buses or electrically operated rail-bound transport.
In addition, the expansion of electromobility offers systemic advantages for electricity generation. Among other things, electric vehicles could be actively integrated into the electricity system by means of smart grids , where they could play a role in balancing out fluctuating feed-in from wind and photovoltaic systems. By providing system services and control power , electric vehicles enable these variable renewable energies to be more closely integrated into the electricity system, which can improve both the greenhouse gas balance of the electricity and the transport sector. However, by integrating electric cars into the electricity system, further measures (such as expanding the grid or building an energy storage structure) will in all probability not become superfluous. From an ecological point of view, the use of future electricity surpluses to operate battery electric vehicles has the second-best environmental balance after use in heat pumps and before electricity storage.
Phases of the energy transition
The transformation of the energy system as part of the energy turnaround is a process that takes several decades and can be divided into different phases. There are various transformation models in the scientific literature, each of which has to deal with different tasks. While z. B. Lund et al. present a three-stage model that consists of the introductory phase, the large-scale system integration and the 100% phase, subdivide Henning et al. and Fischedick the system integration in two sub-phases and thus come to a total of four different phases.
While at the beginning of the energy transition the focus is primarily on raising awareness, developing and launching the necessary technologies ( renewable energies , energy efficiency technologies , etc.) and reducing their costs, in later phases the focus increasingly shifts towards system integration in the electricity sector as well the displacement of fossil fuels from the heating and transport sectors. The individual sectors are increasingly disintegrating and instead become integrated into a single cross-sector networked energy system.
It is also important to make electricity production and demand more flexible, with the first measures being the optimization of the operation of conventional power plants and the expansion of the electricity grid. Measures in the further course of the energy transition include the introduction of demand-side management, and the establishment of short-term memory, the development of electric heat pump heating systems and cogeneration operated heating networks and long-term production of synthetic fuels by chemical long-term memory. In parallel to the four phases, an increase in energy efficiency will be necessary over the entire period, both in the use of electricity and in the heating sector.
Phase 1: Development of renewable energies
In the first phase of the energy transition, which ends with around 25% renewable energies in the electricity mix, the focus is on the development and market launch of basic technologies . These include in particular renewable energies such as photovoltaic and wind power plants , the capacity expansion of which in this phase has hardly any effect on the power supply system. In addition, rapid cost reductions through mass production and economies of scale are achieved in this phase . This phase has now been completed in Germany.
Phase 2: system integration
The second phase of the energy transition begins with a share of around 25% renewable energies in the electricity mix and ends with a share of around 60%. System integration of renewable energies is necessary in this phase. In this phase, the more flexible operation of conventional electricity production plants, the flexibilization of electricity consumption by means of smart grids and load control measures as well as a stronger integration of the heating and transport system through heat pumps and electric vehicles gain importance . The load shifts necessary for security of supply range from minutes to several hours, so that the use of short-term storage systems such as battery storage power plants or pumped storage power plants in combination with buffering by electric vehicles is sufficient.
Phase 3: Synthetic Fuels

The third phase begins with around 60–70% renewable energies in the electricity mix, although a later start is also possible if there is significant electricity imports from solar thermal power plants in North Africa. In this phase, there will be increasingly greater surpluses in regenerative electricity production, which will make the use of chemical long-term storage ( power-to-gas ) necessary. Possible storage media are, for example, hydrogen , methane or also methanol , which can be produced by electrolysis of water with excess green electricity and, if necessary, subsequent methanation or methanolization. Instead of being converted back into electricity with a loss of efficiency, these would initially be used in the transport sector, where they would directly replace fossil fuels. Both fuel cell vehicles and gas vehicles are conceivable here, which can be fueled with artificial methane, biomethane and fossil natural gas. The phase ends with the electricity sector being fully supplied with green electricity.
Phase 4: Complete regenerative energy supply
In the fourth phase, there will finally be a complete displacement of fossil fuels (especially natural gas ) in the heating and transport sector. In Germany, it is currently not foreseeable whether this complete displacement will take place exclusively through domestic renewable energies, or whether energy imports will also be used, for example through synthetic fuels obtained from green electricity. Although Germany has the potential to be supplied entirely from domestic regenerative energies, the partial import of energy reduces storage requirements and increases energy security .
In this phase, the individual components of the regenerative energy system, i. H. the individual renewable energies, efficiency measures, storage, etc. no longer compete with conventional energy, but with each other. It is important here to coordinate the individual technologies with one another, both qualitatively and quantitatively , in order to enable the most effective overall system possible. Due to the large proportion of fluctuating renewable energies, maintaining system stability is particularly important.
Integration of regenerative producers in the energy system
The production of electricity by wind power -, photovoltaics - and to a much lesser extent also by hydropower plants is determined by the weather and is therefore volatile and does not depend on demand. In addition, photovoltaic systems can only supply electricity during the day and are subject to pronounced seasonal fluctuations, while solar thermal power plants with heat storage can in principle also supply electricity at night. The production of wind turbines is also subject to strong fluctuations due to the weather, but the variation over the year is significantly less and they can supply electricity both during the day and at night. With wind power, a secured output in the range of 5 to 6% of the nominal output is expected.
To ensure security of supply, other measures must be used than in an energy system that is dominated by base-load power plants. Since regulating volatile producers does not make sense and, accordingly, interventions in their generation behavior offer practically no advantages, the adjustment of production to demand must be balanced out by other components of the energy system. There are a number of options for this, which can be used individually or together: B.
- Linking variable producers in geographically distant regions through network expansion
- The combination of different regenerative energies to smooth the feed-in
- The addition of variable producers with base load-capable power plants (e.g. biomass or geothermal power plants )
- The implementation of smart grids to adapt demand to fluctuating generation through load control
- The expansion of electricity storage systems in the energy system or directly at the point of generation
- The use of thermal storage for heat generation ( power-to-heat )
- The oversizing of regenerative power plants combined with the production of hydrogen from temporary surplus production
- The storage of electrical energy in electric vehicles
- The planning of energy production according to solar and wind power forecast
In principle, the integration of renewable energy sources can therefore be divided into two phases: If the proportions of variable renewable energies are low, their integration into the existing electricity system is not a problem, as their fluctuating power output can initially be offset by the existing base- load-capable power plant fleet. Only with higher proportions of wind and solar power do additional measures such as grid expansion or the construction of storage power plants have to be taken. The principle here is that long-distance transmission using HVDC is generally economically superior to the storage of electricity and should therefore be preferred as far as possible.
activities
Network expansion
Before the expansion of renewable energies, the power grid was tailored to the operation of relatively few large thermal power plants. Electricity was produced in large power plant blocks, stepped up to 220 kV or 380 kV, in high-voltage lines transported to the centers of consumption, in substations transformed down in high-voltage (110 kV) and regionally distributed. The distribution to the end customer finally took place in the medium and low voltage level , for some large industrial consumers e.g. Sometimes also directly through high-voltage lines. Electricity flowed almost exclusively from high voltage levels to lower ones, where it was used. With the expansion of renewable energies, the lower network levels, which were originally designed as (almost) pure distribution networks, increasingly became feed-in networks. Local or regional network reinforcements or the installation of controllable transformers are necessary so that these networks are still able to cope with increased current flows without increasing voltage .
The need to expand the grid results in particular from the expansion of wind energy. Wind farms are often built in regions where there was originally no high electricity demand and accordingly the distribution networks were only weakly dimensioned, for example in the predominantly rural coastal areas of northern Germany, far from the consumption centers in the Ruhr area and southern Germany. There the grids must be reinforced accordingly in order to be able to absorb the increasing wind power feed-in. The same applies to the transmission grids, whereby, in addition to the expansion of wind energy, the European electricity trade , which is already aimed at with the liberalization of the electricity market , is creating a need for expansion. As a result of these two aspects, the networks are now confronted with loads for which they were not originally designed.
Regional balancing effects, which occur with wind energy and, to a lesser extent, with solar energy, play an important role in grid expansion within the framework of the energy transition. Compared to a single wind turbine , the feed-in of a wind farm is more constant; However, major compensatory effects only arise through the connection of more distant regions in different countries with different weather zones . By expanding the grid, the feed-in of renewable energies can be stabilized and the storage requirement and the need for control and balancing energy can be significantly reduced. A power grid linked across Europe thus enables a more simple, renewable full supply than a purely national approach through supra-regional balancing effects and is cheaper than an energy system that makes heavy use of power storage systems with lower efficiency levels. This means that the need for more expensive and more lossy energy storage can be postponed, but with very high proportions close to full supply, this cannot be completely replaced. In order to achieve even greater balancing effects, even global power grids are sometimes proposed, which should work using HVDC technology . According to Quaschning, this type of power transmission results in losses of less than 14% over transport distances of 5,000 km and a voltage of 800 kV. The investment costs for the power lines themselves are forecast at 0.5 to 1 ct / kWh. Chatzivasileiadis, et al. indicate transport losses of 3% per 1000 km, which means that with today's technology, even with a transmission distance of 6,000 km, lower losses occur than with storage in pumped or compressed air storage power plants.
Making the energy system more flexible

With an increasing proportion of variable producers in the electricity system, the flexibilization of consumption and demand as well as the integration of the heating and transport sector into the electricity system play an important role. The flexibilization of the energy system comprises a large number of individual elements, whereby the overall systemic approach, i. H. The consideration of the entire energy system offers more and better possibilities than measures that only have one-sided focus on the electricity sector . Compared to the expansion of storage power plants, the flexibility is significantly cheaper and technically more efficient, so that it should have priority over the construction of storage facilities. Individual measures to increase flexibility are e.g. B. the development of intelligent power networks (smart grids), the introduction of vehicle-to-grid structures to link the electricity sector and electric vehicles on both sides, and the development of combined heat and power systems with power-to-heat technology and heat storage for flexible and decoupled use of electricity and heat generation. Load control in the form of demand-side integration , which enables both the provision of control power and the shifting of loads at more favorable times, is also becoming increasingly important . The practically feasible potential for such measures is estimated in Germany in the household and commercial sector at around 8 GW, around 16% of the maximum electricity demand.
Linking the electricity sector with the heating and transport sectors offers great advantages. A study carried out for the Helsinki metropolitan area showed that by integrating the electricity and heating sectors using power-to-heat wind turbines, up to. 60% of the region's annual electricity demand and 30% of the heat demand could be covered without a larger storage requirement. Heat storage systems therefore play an important role in making the energy system more flexible . Heat storage systems can be built in different sizes, ranging from decentralized small systems to large central storage systems, are available as short-term as well as seasonal storage systems and, depending on the design, can absorb and release low-temperature heat for space heating and high-temperature heat for industrial applications; It is also possible to store cold for air conditioning or for commercial purposes. A distinction is made between storage for sensible heat , latent heat storage and thermochemical heat storage . In particular, large central heat storage systems in district heating networks are very cost-effective and, by means of power-to-heat and (large) heat pumps, enable both the effective integration of large amounts of wind power and the variable operation of combined heat and power plants, which can create a very energy-efficient energy system. In addition, such heat accumulators are state of the art and have great potential for load management , while at the same time they have much lower costs than other (electrical) storage systems.
Underground heat storage in the rock is also an option for domestic heating requirements. The rock is initially heated by heat sources ( e.g. solar thermal energy in summer or excess electricity from renewable energies). During the heating season, the stored heat can be made available again either directly or with heat pumps. Cold can also be stored in the same way. Such a system is used e.g. B. in the Drake Landing Solar Community in Canada .
Use of storage power plants

In the public debate, the position is often taken that electricity storage systems are necessary even with small proportions of renewable energies; an opinion that is wrong. In fact, the scientific literature assumes that there is only a greater need for storage systems from an annual share of around 40% renewable energies in electricity generation; occasionally a share of 70% is also mentioned.
Below 40% of renewable energies, compensation through thermal power plants and a slight reduction in generation peaks from renewable energies (around 260 GWh per year or 1 per mille of the forecasted green electricity generation with a 40% share) represent an economically more efficient option for compensation The reason for this is that in this case storage facilities would largely be used to improve the utilization of lignite-fired power plants operated at base load at the expense of less emission-intensive power plants, which increases greenhouse gas emissions instead of lowering them as intended. At the same time, the costs of building new storage facilities significantly exceed the benefits of a more uniform power plant operation. It should also be taken into account that the expansion of the electricity grid is more economically expedient than the expansion of storage facilities; however, storage systems often find greater support among the population than new networks.
If there is a flexible power plant fleet and a favorable mix of wind and photovoltaic systems, (day) storage systems are only required in Germany when the proportion of these two energy sources reaches around 50%. Seasonal long-term storage based on power-to-gas technology will be necessary from around 80%. It is also important that the use of power-to-gas only makes sense in terms of energy and saves emissions if green electricity is used. If, on the other hand, electricity from fossil fuels is used, storage has a counterproductive effect and emissions are multiplied. For example, if electricity is used from a lignite power station , which emits 1161 g CO 2-eq. / kWh, with 60% efficiency each for the storage process and reconversion in the combined cycle power plant, total emissions would be 3225 g CO 2 -equiv. / kWh; about eight times the amount of electricity from a fossil-fuel natural gas power plant.
Pumped storage power plants , battery storage power plants and compressed air storage power plants can be used as day or short-term storage , as well as decentralized solar batteries . As of 2015, pumped storage power plants accounted for 99% of the world's installed storage power plant capacity, but their expansion potential is limited for geographical reasons. Therefore, more storage technologies as well as various Power-to-X technologies that aim to use electricity outside the electricity sector for heating or transport applications are increasingly moving into the focus of research. While pump storage is a technology that has been tried and tested for decades, there are still relatively few battery and only two compressed air storage power plants around the world. Research-driven major technological advances have been made particularly in battery technology, both for stationary (energy storage) and for mobile applications ( electromobility ). If lithium-based accumulators have been predominantly used in both applications up to now, the trend for stationary applications is towards cheaper storage technologies that are uncritical in terms of resource requirements, such as B. sodium ion accumulators , organic redox flow batteries or aluminum ion accumulators , the z. T. also promise significantly higher storage cycle numbers.
As long-term or seasonal storage, apart from a few seasonal hydropower plants in advantageous locations, v. a. Chemical energy storage provided, for example in the form of hydrogen , methane or dimethyl ethers , for which only a number of prototypes and test systems exist so far. The technology required for this has long been known in principle. For example, the Danish wind power pioneer Poul la Cour started operating a wind power plant with an attached electrolyzer as early as 1895, which supplied oxyhydrogen for the gas lighting of the school in Askov. However, the large-scale production of hydrogen, which is necessary for the energy transition and adapted to the changing electricity supply, by means of water electrolysis using wind or solar energy and, if necessary, the subsequent methanation of the hydrogen is currently still at the beginning of its testing, so this process has yet to be brought to series production. Above all, it is important to increase efficiency and reduce costs so that the technology can then be used with a green electricity share of approx. 70%. The functionality of power-to-gas, on the other hand, has already been demonstrated by several existing prototypes. The negative aspect of conventional power-to-gas systems is the low level of efficiency compared to other storage technologies. Since the energy chain electricity - hydrogen / methane - electricity is therefore associated with quite high energy losses, which in turn leads to an increased need for wind power and photovoltaic systems, a future energy system should be designed in such a way that there is only a low long-term storage requirement. However, research results indicate that, in the future, through the use of reversibly operated solid oxide fuel cells and an optimized waste heat concept, electricity-to-electricity efficiencies of just over 70% are possible even with power-to-gas.
How a regenerative energy system works
While in a conventional energy system energy production of the energy demand will continue to adjust and thus a balance between production and consumption can be made, it will also come in a renewable energy system by the variability of the main producers of wind energy and solar energy alternately to both coverage than shortfall in electricity demand . A full regenerative supply accordingly requires a different approach in order to be able to guarantee security of supply at all times. In the scientific literature, there is a large number of publications that deal with balancing out fluctuations. In 2014, Palzer and Henning published a work that models the conditions of a full regenerative supply in the German electricity and heating sector in 2015 using an hourly time series . The goal was u. a. to examine and better understand the interactions between the individual system components such as producers, consumers and storage facilities over the course of the year. Depending on the season, there are different optimal strategies for action.

In winter , there is an excess of electricity during times of high wind power feed-in. The heating energy is largely provided by electrical heat pump heating . For reasons of efficiency, the excess electricity that occurs is initially stored in the pumped storage and battery storage power plants, which have a high degree of efficiency. When these are fully charged, the excess production is channeled into power-to-gas systems that use electrolysis to produce synthetic fuels. Further surpluses are fed into local and district heating networks using power-to-heat . During times of low green electricity production, when production cannot cover electricity demand on its own, the short-term storage (pumped storage and batteries) are initially discharged. Whose capacity is not sufficient, in addition come in cogeneration powered combined cycle power plants and cogeneration plants are used, which are fired with previously generated wind or solar gas. When the feed-in from renewable energies increases again, the short-term storage systems are then initially charged again.
In spring , during the day, electricity production through high photovoltaic feed-in usually exceeds demand, so that no stored electrical energy is required during this time. At the same time, the heating requirement is low during the day, so that, in contrast to winter, electric heat pumps do not have to be used or only rarely. As in winter, excess production occurring during the day is initially stored in short-term storage systems, then in power-to-gas systems and in thermal storage systems . At night, combined cycle power plants and CHP units fired primarily with RE gas are used to cover the electricity demand; the short-term storage units are also discharged, while heat storage units cover the higher heat requirements at night.
In summer there are occasionally very strong surpluses of electricity during the day, which are used to charge short-term and long-term storage. In addition, they flow into long-term thermal storage, which is used to cover the heat demand during winter. If these are also charged after some time around autumn, renewable generators may be curtailed during periods of high electricity production; Henning and Palzer assume that in Germany approx. 5.3 TWh or 1% of the green electricity production cannot be used in this way. This does not take into account possible capacity bottlenecks in the domestic German distribution, which, according to the authors, can presumably lead to an increase in this value.
Decentralized or centralized energy transition?
In principle, the primary goal of the energy transition, the realization of a sustainable energy supply without dependence on fossil and nuclear fuels, can be achieved both decentrally and centrally. A clear separation into centralized models and decentralized energy structures is also not even possible because the boundaries between the systems are fluid. Historically, the energy transition began in Denmark as well as in Germany in the 1970s and 1980s as a decidedly decentralized concept that was driven forward in deliberate delimitation from the conventional energy industry, which was perceived as the cause of ecological problems and was almost exclusively centrally shaped . By contrast, operators of small and decentralized systems, especially regenerative systems, experienced strong resistance in Germany until the 1990s from the established energy suppliers , who at that time were still acting as regional monopolists , and (economic) policy.
As a result, with the expansion of regenerative systems, which was mainly driven by private individuals, citizens' energy cooperatives , etc., there was a major change in the electricity market and its decentralization, especially since the four large German energy supply companies had only invested small amounts in renewable energies. In 2010 their share in the installed regenerative capacity was only 6.5%. With the advancement of technology and larger projects, particularly in wind energy, which also require higher investment sums, there has since been a greater mixing of decentralized and centralized structures. Today, most of the concepts are based on the use of decentralized as well as centralized structures, whereby the advantages of both forms of energy generation (such as low costs, low storage requirements and strong public participation ) should be combined as far as possible. However, it is still controversial to what extent the future energy system should be centralized or decentralized and how strongly an optimal energy system should be networked. For example, complete decentralization, including regional energy self-sufficiency, is unrealistic due to the high storage requirements of such considerations; Purely economically and technically optimized scenarios, on the other hand, underestimate the social components of the energy transition and the risk of path dependencies , especially due to the strong influence of the energy suppliers defending their previous market position in these scenarios.
The catalysis researcher Robert Schlögl thinks the energy revolution not succeeded, if often merely the replacement of fossil power plants would be understood by renewable energy sources, energy revolution and was being held at the premise of exclusive self-sufficiency in renewable energy and derived decisions. Striving for energy self-sufficiency would be "nonsensical", it would be "simply impossible in terms of size alone". In the transport sector, electric cars have better overall efficiency levels (see also well-to-wheel ), but batteries still have a very small capacity in relation to their weight, a very limited service life and would be expensive. Schlögl therefore suggests the production of synthetic fuels in sunnier countries and their import based on the DESERTEC concept .
Decentralized concept components

In general, in contrast to the fossil fuels available at certain points in the mining districts and at the extraction points, renewable energies are distributed over the whole country and can thus be used decentrally almost everywhere. Especially in the case of biomass plants, decentralized firing systems such as heating plants and combined heat and power plants result in environmental advantages compared to centralized use, since in this way the fuels such as wood and straw do not have to be transported over long distances. In such systems, a better fuel utilization through combined heat and power is possible than in large power plants that are often located outside of consumption centers, which often extract no or only small amounts of district heating . The decentralized photovoltaic feed, in turn, enables transmission and distribution networks to be relieved of fluctuating demand, whereby large installed photovoltaic capacities in areas with only weakly dimensioned networks can have the opposite effect.
While the variable feed-in of wind and sun must be balanced out to ensure security of supply, the inevitably decentralized generation of electricity due to the expansion of renewable energies has a positive effect on grid stability, which can increase security of supply. The reason for this is that decentralized structures are less prone to large-scale failures than structures dominated by large power plants. However, since some of the renewable energy producers feed fluctuatingly into the power grid, measures such as overhead line monitoring and the introduction of a smart grid may be necessary to keep the voltage of the power grid stable. Also Virtual power plants in which various renewable energy producers and, where appropriate customers are intelligently linked, contribute to security of supply. In addition, renewable energies, especially photovoltaics, can step in when conventional power plants have to be throttled or shut down completely in summer due to excessive river warming due to the cooling water released, an effect that has been known for a long time and which will occur more and more frequently as global warming increases .
In addition to these ecological and technical aspects, political and economic reasons for expanding decentralized energy structures are also given. For example, Jürgen Karl sees the main reasons for the increasing expansion of decentralized energy structures in the low willingness to invest in the construction of large-scale fossil-fuel power plants, resulting from the liberalization of the electricity market , the necessary electrification in the emerging countries outside of the booming centers as well as the low acceptance of large-scale power plants in industrialized countries. Furthermore, decentralized concepts are mostly propagated by representatives of the left political spectrum as an essential part of a more comprehensive social transformation towards a more equal and more democratic society . Representatives of this trend particularly emphasize the social dimension of sustainability and see energy self-sufficiency and the supply by means of many small local cooperatives as an opportunity to make large infrastructures and thus also energy companies superfluous. For the most part, proponents of a rapid energy transition strive for a more decentralized approach.
Proponents of the decentralized approach emphasize that through participation models such as community wind parks , community solar parks and community energy cooperatives, many citizens can be directly involved in the generation of energy, while photovoltaic systems can even be built by individuals. Last but not least, the decentralized construction of renewable energies enables added value in the region and a strengthening of rural areas, so that capital outflows from the region can be minimized. With this justification, renewable energies play an increasingly important role in municipal energy policy and are often promoted by local politics.
Central concept components


Another trend, on the other hand, sees the energy transition as a purely technological project that should be designed as efficiently as possible by utilizing maximum economies of scale and highly centralized structures. Representatives of this trend speak out in favor of the concentration of wind farms near the coast as well as the construction of offshore wind farms , a strong expansion of the electricity grid including high-performance transcontinental lines and the import of electricity from North Africa.
At the same time, it is emphasized that the integration of different production rooms in a large-scale network offers advantages over a purely decentralized system, since the fluctuations in the generation of wind and solar energy can be reduced by exchanging electricity over regions further away and thus the storage requirement can be reduced. While three-phase power lines are to be used primarily in the national context, power lines based on high-voltage direct current transmission technology are planned to link more distant areas and are to be combined to form so-called supergrids . In contrast to AC power lines, HVDCs can be designed as overhead lines, as underground power cables or as submarine cables and, thanks to their very low transmission losses , enable transport distances of several 1000 km; Even global networks are conceivable.
Since the variability of renewable energies decreases with increasing distance, such supergrids are considered to be very important for a cheap grid integration of renewable energies. Some supergrids around the world have been proposed or are already in the planning phase. This includes the coupling of Europe to Africa, the construction of an offshore supergrid in the North Sea and the networking of Australia and Tasmania.
In addition, there are various projects and visions that are considering massively expanding electricity production from controllable solar thermal power plants in southern Europe, North Africa and the Middle East and exporting surpluses that are not needed in these regions for self-sufficiency. At the same time, jobs would be created in the exporting countries, while dependence on fossil fuels could be reduced in Europe. The special characteristics of solar thermal power plants, which are equipped with inexpensive heat storage systems and can continue to produce electricity at night, would also reduce storage requirements in Europe. There is also the possibility of hybridization, i. H. heat generation by natural gas or, in the future, by synthetic fuels , hydrogen or biogas , which means that solar thermal power plants as well as conventional power plants are fully capable of base load . The best- known representative of this project is the Desertec project , the implementation of which is now questionable.
Economic consideration
Economic prognoses for the energy transition are fraught with great uncertainties, since the energy transition is a process that extends over several decades, and at the same time a number of technological, economic and social variables must be considered in the forecast, the development of which can only be partially estimated is. These include, for example, the development of the electricity production costs of conventional and regenerative producers, the price trend of energy raw materials and, if applicable, pollution rights , changes in demographics and energy consumption or the selected future energy infrastructure (more central or more decentralized?). Additional cost factors compared to conventional energy supply arise from the restructuring of the energy infrastructure, such as the expansion of the grid and the integration of energy storage systems, which is necessary as part of the energy transition, while the avoidance of greenhouse gas emissions, the effects of climate change and damage to health as a result of pollution from the combustion of fossil fuels result in economic cost savings . Depending on the weighting of the different factors, z. Sometimes different results, which are sometimes controversial in the public debate in Germany.
Economic consideration
Energy source | ct / kWh |
---|---|
Brown coal | 8.7 |
Hard coal | 6.8 |
Heating oil | 6.1 |
natural gas | 3.9 |
Photovoltaics | 0.8 |
Hydropower | 0.4 |
Wind energy | 0.1 |
Electricity mix 2005 | 5.8 |
When generating energy, there are both internal (i.e. business ) and external, economic costs. While the internal costs essentially consist of the construction, operation and dismantling of power plants as well as the procurement of fuel, which are mostly subject to market mechanisms, external costs manifest themselves primarily in the form of environmental pollution , health and climate damage , which is not caused by those responsible but by the general public be worn. The actual full costs of energy generation are therefore difficult to determine; In some cases, the social and ecological costs of conventional energy generation even exceed the end customer prices of electricity consumers.
A number of studies on external costs are available for the USA. Shindell, for example, puts the environmental damage from electricity production in the USA at 330–970 billion US dollars per year, most of which can be attributed to the burning of fossil fuels. Machol and Rizk came to the conclusion that the damage to health caused by burning fossil fuels alone causes a total economic damage of approx. 362 to 887 billion US dollars per year. This would result in health follow-up costs of 14 to 35 US cents / kWh, which would significantly exceed the local end customer prices for electricity. Jacobson et al. on the other hand, examined the external costs of the entire energy sector (electricity, heat and transport) and quantified the external costs saved in the event of a complete energy transition by 2050. According to this, a switch in the USA to a 100% renewable energy supply in 2050 would amount to approx Save $ 600 billion in healthcare costs and approximately $ 3.3 trillion in climate damage.
If a full cost calculation is made that also includes external costs for the respective technologies, many renewable energies are already cheaper than conventionally generated electricity. Although these also incur external costs, they are significantly lower than with fossil fuels. However, since the external costs of conventional energy generation have not yet been included in the prices of fossil fuels, in practice there is a market failure in favor of the conventional energy industry. The market principle thus leads to a suboptimal use of energy resources under the current economic framework: fossil fuels appear cheaper than they are economically due to external costs that are not taken into account. However, if the market is to find the most economically efficient mode of production , as is the aim of liberalization , then it is imperative that all factors that distort competition are avoided and that costs are true by internalizing all external factors. If this does not happen, the efficiency advantages of a liberalized market can be negated by negative effects on the environment. Ways to produce this true cost in relation to global warming are incentive taxes such as B. a CO 2 tax or a functioning emissions trading system .
So far (April 2014) these external effects have only been internalized to a small extent; full internalization is not foreseeable. So z. For example, the annual report on energy consumption in Germany in 2013 by the AG Energiebilanzen came to the conclusion that “the incentives intended with emissions trading for emission-reducing behavior at such certificate prices [of approx. 5 euros / ton] are not to be expected”. The lack of internalization of external costs is seen as a decisive obstacle to the progress of the energy transition.
In addition, the electricity production costs are heavily distorted by subsidies for individual technologies, with conventional energy sources receiving subsidies that are several times higher than renewable energies, which lose competitiveness as a result. In 2011, fossil energies were subsidized worldwide with 523 billion US dollars, while renewable energies were subsidized with around 100 billion dollars. Including external costs, fossil fuel subsidies this year amounted to approximately 1.9 trillion US dollars, according to Jiang and Lin. The International Monetary Fund , however, gives higher numbers. According to this, the subsidies, including the economic costs of environmental and health damage, totaled 4.2 trillion US dollars in 2011 and 4.9 trillion US dollars in 2013. For 2015 the subsidies are estimated at 5.3 trillion US dollars, which corresponds to 6.5 percent of the world gross national product and is thus higher than the global expenditure in the health sector. At the same time, these subsidies for oil, coal and gas would keep energy prices artificially low, slow down the expansion of renewable energies and increase climate-damaging emissions by 17%. Based on these figures, the production of one tonne of carbon dioxide in the energy sector was subsidized by more than US $ 150 in 2013.
Business consideration
Energy source | Electricity generation costs in ct / kWh | ||
---|---|---|---|
Data origin: Fraunhofer ISE 2018 | |||
Brown coal | 4.59-7.98 | ||
Hard coal | 6.27-9.86 | ||
Natural gas CCGT | 7.78-9.96 | ||
Natural gas gas turbine power plant | 11.03-21.94 | ||
Wind / onshore | 3.99-8.23 | ||
Wind / offshore | 7.49-13.79 | ||
Biogas (without heat extraction) | 10.14-14.74 | ||
Small photovoltaic system roof | 7.23-11.54 | ||
Large photovoltaic system roof | 4.95-8.46 | ||
Large photovoltaic power plant open space | 3.71-6.77 |
If you ignore the external costs of conventional and alternative energy generation and only consider the economic costs, then most renewable energies currently (2018) in Germany have roughly similar electricity production costs as conventional energies. An exhibition of the electricity production costs of individual technologies can be found in the table on the right.
Particularly in the case of photovoltaics and onshore wind energy , a continuous decrease in the cost of electricity can be observed. This was triggered by a strong expansion of production and the resulting economies of scale , strong competition and technical developments (' learning curve '), e.g. B. by improving the efficiency. The cost of electricity is expected to fall further, while the opposite effect is observed with conventional energies.
However, for a comparison of fossil and regenerative energy systems, costs for a stronger grid expansion and electricity storage must also be taken into account, which are also incurred in a regenerative energy system. Assuming that electricity to exchange regional fluctuations is primarily distributed via a cross-state, intelligent supergrid , Jacobson and Delucchi come to the conclusion that these additional costs will probably not exceed 2 US cents / kWh. Given that the costs of fossil fuels will continue to rise in the long term, it is therefore assumed that, viewed in the long term, the energy costs in a regenerative energy system are at the same level as in a fossil-nuclear energy system or will be cheaper than in this.
Hydropower has been an integral part of the electricity mix since the start of electricity production due to its low production costs. For a few years now, wind turbines at good onshore locations have also been competitive with conventional power plants without subsidies . It is expected that in the future there will be competitiveness with coal-fired power plants even in poorer locations and that wind energy will develop into the cheapest form of electricity production. In addition, more and more older wind turbines are reaching an age at which they are written off or are no longer subject to statutory subsidies and can then produce electricity more cheaply for this reason. However, this constellation is only possible if the service life of a wind turbine exceeds the funding period.
The levelized costs of electricity have also fallen sharply worldwide, especially for wind turbines and photovoltaic systems; a trend that, according to Walwyn and Brent, should continue until at least 2030. In the best locations, the electricity production costs of wind turbines are now around 40-50 US dollars / MWh (33.6-42 euros / MWh), although these are also heavily dependent on the quality of the location and the financing conditions. In the USA, onshore wind power plants are already the second cheapest power plants after gas-fired combined cycle plants. The same applies to photovoltaics, for which module prices fell by 60% between January 2011 and December 2012 and for which a further price decline is expected. Overall, the module prices fell by 6-7 USD / watt in 2000 to $ 0.5-0.6 / watt in 2013. In photovoltaics is now in the industry of Swanson's law spoke, after which the price of solar modules with the doubling of the modules delivered falls by 20%.
Photovoltaic systems, solar collectors and, in some cases, wind turbines and biogas systems can be installed as small power plants directly at the end user. Because of the loss of transport costs and taxes due to self-consumption , such power plants do not have to compete or only partially compete with wholesale prices; instead, the profitability is determined by the end customer prices for electricity and possibly heat. In many countries, for example, grid parity for private households, municipalities and most commercial enterprises was achieved in photovoltaics a few years ago , even if the electricity production costs are still higher than those of conventional power plants. In Germany, grid parity for photovoltaic electricity was achieved in 2012. It is assumed that the falling prices of solar power will result in major changes in the electricity sector in the future.
In many countries around the world, photovoltaic island systems with battery storage are also the cheapest form of electricity supply for individual buildings or settlements in rural areas . From an economic as well as ecological point of view, such systems perform better than the alternatives diesel generator or electrification through connection to a power grid.
Space requirement
The replacement of fossil and nuclear power generation technologies by renewable energies is leading to a comprehensive change in land use. A large area is required to provide fossil fuels, in particular lignite, but in many parts of the world also hard coal is extracted in open-cast mining or the mountain top removal mining that is often practiced in the USA . Through the mountain top mining alone, an area of 1.4 million acres (approx. 5700 km²) was completely redesigned and around 2000 miles (approx. 3200 km) of running water were buried under spoil. These serious encroachments on the environment and nature, which occur when coal is mined, can only be partially reversed. The landscape is also being massively and permanently changed. Often people also have to be relocated. In Germany z. For example, around 2,300 km² of land is moved and consumed in open- cast lignite mines alone .
Wind and solar energy
Compared to this, the intervention by wind power and solar systems , which will bear the main load in a regenerative energy system, is significantly less. However, in contrast to conventional energy use, it does not take place selectively or regionally, but is visible on site for much larger sections of the population due to the decentralized character of regenerative energies.
For example, the area used by wind power plants in Germany in 2011 was around 100 km², only a fraction of the area used by open-cast lignite mines, but the wind power plants, which are spread across Germany, are much more present in the visual perception. It must be taken into account here that the actual land consumption of wind turbines, i.e. H. the area sealed by the foundation should not be confused with the space between the individual wind turbines and with residential buildings. While the foundation measures a maximum of a few 100 m², depending on the size of the system, the spacing areas per system are a few hectares. As a result, almost 99% of the area used by a wind farm can usually still be used without restriction for arable farming etc.
That is why the generation of energy from wind energy has a comparatively low space requirement overall, while the surface sealing that results from it is very low compared to conventional forms of energy generation. For example, the energy yield of a modern 3 MW system with 300 m² of stand space and an electricity production of 6.4 GWh is around 21,000 kWh per m² of foundation area and year. This value is slightly above the corresponding value for hard coal power plants (including ancillary buildings), even if the area for coal mining is not included in the latter. On the other hand, the effects on the landscape are judged controversially .
Photovoltaic systems are usually installed on buildings and therefore do not require any additional space. However, additional space consumption occurs with photovoltaic open-space systems . Although hardly any surface is sealed by the usual elevation, since the foundations of the frames require little space, the surfaces in solar parks can either no longer be used at all or can only be used for extensive agriculture , for example as pasture for sheep . It is also possible to create secondary biotopes among the modules, which represent an ecological upgrade compared to intensive agriculture. Wesselak et al. indicate a space requirement of almost 38 m² per kW p for open-space photovoltaic systems . The space required for inverters , paths and spacing areas is already included. In the future, the use of floating photovoltaic systems , which would be built on water surfaces that are not otherwise used, could help avoid potential competition for land with agriculture. A number of prototypes of such systems have meanwhile been realized, but the technology is still at an early stage of development and market launch (status 2014).
In addition, there is the need to expand power grids and energy storage systems for these forms of energy generation, which must also be taken into account. So have z. For example, pumped storage power plants consume a large amount of space, while compressed air storage power plants require significantly less space than pumped storage and at the same time place fewer demands on the topography, but are also less efficient. Long-term storage facilities such as the production of hydrogen or synthetic methane can fall back on storage capacities in the existing natural gas infrastructure and therefore do not require any additional space, with the exception of the facilities for the production of hydrogen or methane. However, the lower efficiency of these systems and the associated higher energy losses during storage in turn increase the need for regenerative generation capacity, i.e. H. Wind power and solar systems.
According to Jacobson and Delucchi, a completely regenerative energy supply for the entire world economy , which is based entirely on wind power, solar energy and water power as well as a small amount of geothermal energy, would require between 0.41 and 0.74% of the earth's surface on a permanent basis. An additional 0.59 to 1.18% would be required as spacing areas for wind turbines, but could continue to be used for agriculture. The space requirement is therefore not considered an obstacle to a global energy transition.
Biomass cultivation
In contrast to the standing areas of wind turbines and possibly open-space solar systems, with the exception of the power plants and ferments themselves, no area is sealed in the case of biomass cultivation, but only used for other agricultural purposes. However, the competition for land and use between food production , cultivation of energy crops and the extraction of renewable raw materials for material use must be taken into account . That is why the cultivation of energy crops is controversial. A so-called cascade use is therefore considered to be more sensible than the direct use of biomass for energy production , in which the material use of plant raw materials is first in the foreground and only after this use has ended, energy recovery.
The specific area required for the production of bioenergy sources is comparatively high compared to other forms of energy generation. Using energy maize as a substrate for biogas plants results in usable biomethane yields of approx. 45 MWh per hectare or 4.5 kWh per m² per year. As of 2017, bioenergy sources were grown on around 14 million hectares of arable land worldwide, which corresponds to around 1% of the world's arable land. See also: Corning .
While the use of agricultural residues and waste materials such as For example, when straw is seen as unproblematic from an ecological and socio-political perspective, the production of biofuels in particular is controversial. In addition, excessive use of biofuels can not only impair food security, especially in less developed countries, but also have strong negative effects on ecology, biodiversity and near-natural habitats. Because of this, the potential of bioenergy is estimated to be significantly lower overall in more recent studies than in older studies. Went z. For example, the IEA has assumed in the past that bioenergy could optimally deliver 700 EJ / a of energy, which would correspond to 60% of the estimated global energy demand in 2050, but more recent studies put the biomass potential at around 180 EJ / a or 15% of the world energy demand in 2050. In order to guarantee the sustainable use of biomass, in some more recent studies that model a full regenerative energy supply exclusively using renewable energies, the use of bioenergy is significantly limited or even completely excluded.
Basically, biomass alone does not have the potential to completely replace fossil fuels. For example, according to Volker Quaschning , Germany would only be able to cover a third of German diesel consumption with biodiesel, even if all the domestic arable land was used for rapeseed cultivation for biodiesel production . The use of electromobility is therefore seen as much more promising than the use of biofuels. The potential of biofuels, on the other hand, is seen primarily in areas where the use of electric vehicles is not practicable even in the long term.
See also
- Club of the Energy Transition States
- Energy transition by country
- Green New Deal
- Renewable energy
- Climate change
Movies
- The 4th Revolution - EnergyAutonomy (2010), The film title describes the energy transition as the fourth revolution after the agricultural revolution, the industrial revolution and the digital revolution.
literature
Reference books
- Nicola Armaroli , Vincenzo Balzani : Powering Planet Earth - Energy Solutions for the Future . Wiley-VCH 2013, ISBN 978-3-527-33409-4 .
- Nicola Armaroli, Vincenzo Balzani: Energy for a Sustainable World - From the Oil Age to a Sun-Powered Future . Wiley-VCH, 2011, ISBN 978-3-527-32540-5 .
- Günther Brauner: Energy systems: regenerative and decentralized. Strategies for the energy transition . Wiesbaden 2016, ISBN 978-3-658-12754-1 .
- Franz-Josef Brüggemeier : Sun, water, wind: the development of the energy transition in Germany . (PDF) Friedrich-Ebert-Stiftung , Bonn 2015, ISBN 973-8-95861-238-9 .
- Achim Brunnengräber , Maria Rosaria du Nucci (Ed.): In the hurdles for the energy transition. About transformations, reforms and innovations. For the 70th birthday of Lutz Mez , Wiesbaden 2014, ISBN 978-3-658-06787-8 .
- Felix Ekardt : The task of the energy transition of the century: A manual . Berlin 2014, ISBN 978-3-86153-791-5 .
- Steven Engler, Julia Janik, Matthias Wolf (eds.): Energy transition and megatrends. Interactions between global social development and sustainability , transcript, Bielefeld 2020, ISBN 978-3-8376-5071-6 for PDF download .
- Sebastian Giacovelli (Ed.): The energy transition from an economic- sociological point of view: Theoretical concepts and empirical approaches . Springer VS, Wiesbaden 2016, ISBN 978-3-658-14344-2
- Josef Gochermann: Expedition Energiewende . Springer Spectrum 2016. ISBN 3-658-09851-1 .
- Matthias Günther: Energy efficiency through renewable energies. Possibilities, potentials, systems , Wiesbaden 2015, ISBN 978-3-658-06753-3 .
- Peter Hennicke , Susanne Bodach: Energy Revolution: Increasing Efficiency and Renewable Energies as a New Global Challenge , published by the Wuppertal Institute for Climate, Environment, Energy . Oekom, Munich 2010, ISBN 978-3-86581-205-6 .
- Martin Kaltschmitt , Wolfgang Streicher, Andreas Wiese (eds.): Renewable energies. System technology, economy, environmental aspects . Springer Vieweg, Berlin / Heidelberg 2013, ISBN 978-3-642-03248-6 .
- Gregor Kungl: The big electricity companies and the energy transition . Campus, Frankfurt am Main 2018, ISBN 978-3-593-50942-6 .
- Henrik Lund : Renewable Energy Systems: A Smart Energy Systems Approach to the Choice and Modeling of 100% Renewable Solutions , Academic Press 2014, ISBN 978-0-12-410423-5 .
- Volker Quaschning : Renewable energies and climate protection. 4th edition. Hanser, Munich 2018, ISBN 978-3-446-45703-4 .
- Volker Quaschning : Regenerative Energy Systems. 9th edition. Hanser, Munich 2015, ISBN 978-3-446-44267-2 .
- Holger Rogall : 100% supply with renewable energies. Conditions for global, national and local implementation . Marburg 2014, ISBN 978-3-7316-1090-8 .
- Hermann Scheer : The energy ethical imperative: 100 percent now. How to implement the complete switch to renewable energies . Kunstmann, Munich 2010, ISBN 978-3-88897-683-4 .
- Michael Sterner , Ingo Stadler (Hrsg.): Energy storage - demand, technologies, integration . 2nd edition Berlin / Heidelberg 2017, ISBN 978-3-662-48892-8 .
- Viktor Wesselak , Thomas Schabbach , Thomas Link, Joachim Fischer: Handbuch Regenerative Energietechnik , 3rd updated and expanded edition, Berlin / Heidelberg 2017, ISBN 978-3-662-53072-6 .
Technical articles
- Nicola Armaroli , Vincenzo Balzani : Towards an electricity-powered world . In: Energy and Environmental Science 4, (2011), 3193-3222, doi: 10.1039 / c1ee01249e .
- Antje Boetius , Ottmar Edenhofer , Bärbel Friedrich , Gerald Haug , Frauke Kraas , Wolfgang Marquardt , Jürgen Leohold , Martin J. Lohse , Jürgen Renn , Frank Rösler , Robert Schlögl , Ferdi Schüth , Christoph M. Schmidt , Thomas Stocker 2019: Climate targets 2030: Paths to a sustainable reduction in CO2 emissions. . Statement by the National Academy of Sciences Leopoldina .
- Hans-Martin Henning , Andreas Palzer: A comprehensive model for the German electricity and heat sector in a future energy system with a dominant contribution from renewable energy technologies — Part I: Methodology. In: Renewable and Sustainable Energy Reviews 30, (2014), 1003-1018, doi: 10.1016 / j.rser.2013.09.012 .
- Andreas Palzer, Hans-Martin Henning: A comprehensive model for the German electricity and heat sector in a future energy system with a dominant contribution from renewable energy technologies — Part II: Results. In: Renewable and Sustainable Energy Reviews 30, (2014), 1019-1034, doi: 10.1016 / j.rser.2013.11.032 .
- Olav Hohmeyer , Sönke Bohm: Trends toward 100% renewable electricity supply in Germany and Europe: a paradigm shift in energy policies . In: Wiley Interdisciplinary Reviews: Energy and Environment 4, (2015), 74–97, doi: 10.1002 / wene.128 .
- Mark Z. Jacobson , Mark A. Delucchi: Providing all global energy with wind, water, and solar power, Part I: Technologies, energy resources, quantities and areas of infrastructure, and materials . In: Energy Policy 39, Vol. 3, (2011), 1154–1169, doi: 10.1016 / j.enpol.2010.11.040 .
- Mark A. Delucchi, Mark Z. Jacobson : Providing all global energy with wind, water, and solar power, Part II: Reliability, system and transmission costs, and policies . In: Energy Policy 39, Vol. 3, (2011), 1170–1190, doi: 10.1016 / j.enpol.2010.11.045 .
- Henrik Lund et al .: From electricity smart grids to smart energy systems - A market operation based approach and understanding . In: Energy 42, Issue 1, (2012) 96-102, doi: 10.1016 / j.energy.2012.04.003 .
- Brian Vad Mathiesen et al .: Smart Energy Systems for coherent 100% renewable energy and transport solutions . In: Applied Energy 145, (2015), 139–154, doi: 10.1016 / j.apenergy.2015.01.075 .
Web links
- Information portal for renewable energies (Federal Ministry for Economic Affairs and Energy)
- Energy Transition - The German Energiewende - Information about the energy transition in English
- Energiewende on the information portal for political education
- Scientific Advisory Council of the Federal Government Global Environmental Change : World in Transition. Energy transition towards sustainability . Springer, Berlin 2003, ISBN 3-540-40160-1 ( online (PDF) PDF, 3.84 MB).
- Paths to 100% renewable electricity supply Special report by the German Advisory Council on Environmental Issues
- Energy concept of the Research Association for Renewable Energies FVEE (PDF; 4.35 MB) A vision for a sustainable energy concept based on energy efficiency and 100% renewable energies
- Volker Quaschning : Sector coupling through the energy transition. Requirements for the expansion of renewable energies to achieve the Paris climate protection targets, taking into account sector coupling . Berlin University of Technology and Economics , 2016
- Energy system Germany 2050 . (PDF) Study by the Fraunhofer Institute, 2013
- Global Energy Assessment - Toward a Sustainable Future . Cambridge University Press, Cambridge UK / New York NY and the International Institute for Applied Systems Analysis, Laxenburg 2012, ISBN 978-1-107-00519-8 .
- Agora Energiewende , Berlin 2013: 12 theses on the energy transition. A contribution to the discussion on the most important challenges in the electricity market. (PDF; 2.97 MB)
- visualcapitalist.com , October 31, 2018, Iman Gosh: Visualizing the Global Transition to Green Energy (graphic "Global transition to green energy")
Individual evidence
- ^ Nicola Armaroli , Vincenzo Balzani : Towards an electricity-powered world . In: Energy and Environmental Science 4, (2011), 3193-3222, p. 3203 doi: 10.1039 / c1ee01249e .
- ^ MA Sayegh et al. Heat pump placement, connection and operational modes in European district heating . In: Energy and Buildings 166 (2018), 122–144, pp. 128f. doi: 10.1016 / j.enbuild.2018.02.006
- ↑ Aviel Verbruggen: Could it be that Stock-Stake Holders Rule Transition Arenas? in: Achim Brunnengräber , Maria Rosaria du Nucci (Ed.): In the hurdles for the energy transition. About transformations, reforms and innovations. On the occasion of Lutz Mez's 70th birthday , Wiesbaden 2014, 119–133, p. 120.
- ↑ Energy transition in Germany: definition, goals and history
- ^ Roland Roth: Local politics. ISBN 978-3-322-93826-8 , p. 664 ( limited preview in Google book search).
- ↑ Welcome To The Energiewende: The Movie
- ↑ Florian Lüdeke-Freund, Oliver Opel: Energie , in: Harald Heinrichs , Gerd Michelsen (Ed.): Sustainability Sciences, Berlin / Heidelberg 2014, p. 429.
- ↑ Philippe Poizot, Franck Dolhem: Clean energy new deal for a sustainable world: from non-CO2-generating energy sources to greener electrochemical storage devices . In: Energy and Environmental Science 4, (2011), 2003-2019, p. 2003, doi: 10.1039 / c0ee00731e .
- ^ Nicola Armaroli , Vincenzo Balzani : The Future of Energy Supply: Challenges and Opportunities . In: Angewandte Chemie International Edition 46, (2007), 52-66, p. 52, doi: 10.1002 / anie.200602373 .
- ↑ a b Christophe McGlade, Paul Ekins: The geographical distribution of fossil fuels unused When limiting global warming to 2 ° C . In: Nature 517, (2015), 187-190, doi: 10.1038 / nature14016 .
- ↑ International Organization for Renewable Energies : Definition according to Article III of the statutes of January 26, 2009 ( Federal Law Gazette II p. 634, 635 , bilingual).
- ↑ Geoffrey P. Hammond, Peter JG Pearson: Challenges of the transition to a low carbon, more electric future: From here to 2050 . In: Energy Policy 52, (2013), 1–9, p. 6, doi: 10.1016 / j.enpol.2012.10.052 .
- ↑ Volker Quaschning : Regenerative Energy Systems. Technology - calculation - simulation . 9th updated edition. Munich 2015, p. 56.
- ^ A b c Mark Z. Jacobson , Mark A. Delucchi: Providing all global energy with wind, water, and solar power, Part I: Technologies, energy resources, quantities and areas of infrastructure, and materials . In: Energy Policy 39, Vol. 3, (2011), 1154–1169, doi: 10.1016 / j.enpol.2010.11.040 .
- ^ Nicola Armaroli , Vincenzo Balzani : Towards an electricity-powered world . In: Energy and Environmental Science 4, (2011), 3193-3222, p. 3216, doi: 10.1039 / c1ee01249e .
- ↑ a b Mark A. Delucchi, Mark Z. Jacobson : Providing all global energy with wind, water, and solar power, Part II: Reliability, system and transmission costs, and policies . In: Energy Policy 39, Vol. 3, (2011), 1170–1190, doi: 10.1016 / j.enpol.2010.11.045 .
- ^ A b Andreas Palzer, Hans-Martin Henning: A comprehensive model for the German electricity and heat sector in a future energy system with a dominant contribution from renewable energy technologies — Part II: Results. In: Renewable and Sustainable Energy Reviews 30, (2014), 1019-1034, p. 1027, doi: 10.1016 / j.rser.2013.11.032 .
- ↑ a b Deng et al .: Transition to a fully sustainable global energy system . In: Energy Strategy Reviews 1, (2012), 109–121, p. 118, doi: 10.1016 / j.esr.2012.07.003 .
- ↑ a b Olav Hohmeyer , Sönke Bohm: Trends toward 100% renewable electricity supply in Germany and Europe: a paradigm shift in energy policies . In: Wiley Interdisciplinary Reviews: Energy and Environment 4, (2015), 74–97, p. 91f, doi: 10.1002 / wene.128 .
- ^ Benjamin Biegel, Lars Henrik Hansen, Jakob Stoustrup, Palle Andersen, Silas Harbo: Value of flexible consumption in the electricity markets . In: Energy 66, (2014), 354-362, p. 354 doi: 10.1016 / j.energy.2013.12.041 .
- ↑ Dirk Dubbers , Johanna Stachel , Ulrich Uwer: Is there an energy transition taking place? (PDF; 253 kB) Physics Institute of the University of Heidelberg, accessed on February 18, 2019 .
- ↑ Ulrike Fettke, Gerhard Fuchs, Incumbent Challenger Interactions and the Changes in the Market for Power Generation and Distribution in Germany , in: Sebastian Giacovelli (Ed.): The energy transition from an economic sociological point of view. Theoretical concepts and empirical approaches . Wiesbaden 2017, 15–44, p. 22.
- ^ Felix Ekardt : Theory of Sustainability Baden-Baden 2011, p. 379; Günther Brauner: Energy systems: regenerative and decentralized. Strategies for the energy transition . Wiesbaden 2016, p. 184; See also Viktor Wesselak , Thomas Schabbach , Thomas Link, Joachim Fischer: Handbuch Regenerative Energietechnik , Berlin / Heidelberg 2017, pp. 36–38.
- ^ Arnulf Grübler: Energy transitions research: Insights and cautionary tales . In: Energy Policy 50, (2012), 8–16, doi: 10.1016 / j.enpol.2012.02.070 .
- ^ Robert C. Allen : Backward into the future. The shift to coal and implications for the next energy transition . In: Energy Policy 50, (2012), 17-23, p. 17, doi: 10.1016 / j.enpol.2012.03.020 .
- ↑ Rolf Peter Sieferle : The underground forest. Energy crisis and industrial revolution. Munich 1982, chap. V, especially pp. 240-249.
- ^ Hans-Werner Hahn : The industrial revolution in Germany . Oldenbourg Wissenschaftsverlag, Munich 2005, ISBN 3-486-59831-7 , p. 117 .
- ↑ Rolf Peter Sieferle : The underground forest. Energy crisis and industrial revolution . CH Beck, Munich 1982, ISBN 3-406-08466-4 , p. 252-254 .
- ↑ Franz-Josef Brüggemeier , Michael Toyka-Seid (ed.): Industrial nature. Reader on the history of the environment in the 19th century . Frankfurt / New York 1995, pp. 255-257.
- ↑ a b Joachim Radkau , Lothar Hahn : Rise and Fall of the German Nuclear Industry , Munich 2013, p. 82f.
- ↑ Wilhelm Ostwald : Energetic Basics of Cultural Studies, Dr. Werner Klinkhardt, Verlag, Leipzig 1909, page 44. In: archive.org. Retrieved October 5, 2014 .
- ^ Giacomo Ciamician : The Photochemistry of the Future . In: Science 36, No. 926, (1912), 385-394, doi: 10.1126 / science.36.926.385 .
- ↑ Vincenzo Balzani et al .: Photochemical Conversion of Solar Energy . In: ChemSusChem , 1, (2008), pp. 26-58, here p. 27, doi: 10.1002 / cssc.200700087 .
- ↑ Naomi Oreskes , Erik M. Conway : Merchants of Doubt. How a handful of Scientists obsured the truth on issues from tobacco smoke to Global Warming . Bloomsbury Press, New York 2010, p. 170.
- ↑ Carbon Dioxide Information Analysis Center ( cdiac.ornl.gov ): According to the data series available there , the global emissions in 1896 amounted to 419 million tons of CO 2 , whereas they were 6765 million tons in 2000 and up to Increased to 9167 million tons in 2010.
- ^ Svante Arrhenius : On the Influence of Carbonic Acid in The Air Upon The Temperature of The Earth . 1896, bibcode : 1897PASP .... 9 ... 14A , p. 19 below and 20 above: “… the comparison instituted is of very great interest, as it proves that the most important of all the processes by means of which carbonic acid has been removed from the atmosphere in all times - namely, the chemical weathering of siliceous minerals, - is of the same order of magnitude as a process of contrary effect which is caused by the development of our time, and which must be conceived of as being of a temporary nature. "
- ↑ Garcia et al .: Performance model for parabolic through solar thermal power plants with thermal storage: Comparison to operating plant data . In: Solar Energy , 85, (2011), pp. 2443-2460, here p. 2443, doi: 10.1016 / j.solener.2011.07.002 .
- ↑ Erdem Cuce, Pinar Mert Cuce: A comprehensive review on solar cookers . In: Applied Energy , 102, (2013), pp. 1399-1421, here p. 1400, doi: 10.1016 / j.apenergy.2012.09.002 .
- ↑ Michael Mende : Early industrial drive technology - wind and water power. In: Ulrich Wengenroth (ed.): Technology and economy. VDI-Verlag, Düsseldorf 1993, pp. 289-304, p. 291.
- ↑ a b Erich Hau: Wind power plants - basics, technology, use, economic efficiency. 5th edition. Springer, Berlin Heidelberg 2014, chap. 2 (Electricity from Wind - The First Attempts), in particular pp. 23–44.
- ↑ Jens Nørkær Sørensen: Aerodynamic Aspects of Wind Energy Conversion . In: Annual Review of Fluid Mechanics 43, (2011), 427-448, doi: 10.1146 / annurev-fluid-122109-160801 .
- ↑ Alois Schaffarczyk (Ed.): Introduction to wind energy technology. Munich 2012, p. 37.
- ^ Matthias Heymann : The history of the use of wind energy 1890-1990. Frankfurt am Main - New York 1995, p. 268.
- ↑ Erich Hau: Wind turbines - basics, technology, use, economy. 5th edition. Springer, Berlin Heidelberg 2014, pp. 34–36.
- ↑ Holger Schlör et al .: The system boundaries of sustainability . In: Journal of Cleaner Production 88, (2015), 52–60, p. 52, doi: 10.1016 / j.jclepro.2014.04.023 .
- ^ Rolf Wüstenhagen , Michael Bilharz: Green energy market development in Germany: Effective public policy and emerging customer demand . In: Energy Policy 34, (2006), 1681–1696, p. 1682, doi: 10.1016 / j.enpol.2004.07.013 .
- ↑ Hans Günter Brauch : Energy policy under the sign of climate policy in the transition to the 21st century . In the S. Energy policy. Technical development, political strategies, strategies for action on renewable energies and the rational use of energy , Berlin / Heidelberg 1997, 1–24, p. 12
- ↑ a b Frank Uekötter : Environmental history in the 19th and 20th centuries , Munich 2007, p. 28.
- ^ A b Lutz Mez : Energy Consensus in Germany? A political science analysis of the consensus talks - prerequisites, history, course and post-banter . in: Hans Günter Brauch (Ed.) Energy Policy. Technical development, political strategies, concepts for action on renewable energies and the rational use of energy , Berlin / Heidelberg 1997, 433–448, pp. 433f.
- ↑ Rüdiger Graf: From the forgetting of energy to the theoretical metonymy of energy as a medium for describing society in the 20th century . In: Hendrik Ehrhardt, Thomas Kroll (Hrsg.): Energy in modern society. Zeithistorische Perspektiven , Göttingen 2012, 73–92, p. 84.
- ↑ a b c Linguistics. The energy transition . In: Die Zeit , No. 47/2012.
- ↑ Amory Lovins : Soft Energy Paths: Towards a Durable Peace . (Penguin Books, 1977) ISBN 0-06-090653-7 .
- ↑ Olav Hohmeyer , Sönke Bohm: Trends toward 100% renewable electricity supply in Germany and Europe: a paradigm shift in energy policies . In: Wiley Interdisciplinary Reviews: Energy and Environment 4, (2015), 74–97, p. 75, doi: 10.1002 / wene.128 .
- ^ Bent Sørensen : A plan is outlined according to which solar and wind energy would supply Denmark's needs by the year 2050 . In: Science 189, Number 4199, (1975), 255-260, doi: 10.1126 / science.189.4199.255 .
- ↑ Olav Hohmeyer , Sönke Bohm: Trends toward 100% renewable electricity supply in Germany and Europe: a paradigm shift in energy policies . In: Wiley Interdisciplinary Reviews: Energy and Environment 4, (2015), 74–97, pp. 75f, doi: 10.1002 / wene.128 .
- ^ A b Mario Neukirch: The international pioneering phase of wind energy use. Dissertation. Göttingen 2010, p. 20.
- ^ Benjamin K. Sovacool: Energy policy making in Denmark: Implications for global energy security and sustainability . In: Energy Policy 61, (2013), 829-839, pp. 829f, doi: 10.1016 / j.enpol.2013.06.106 .
- ↑ See Volker Quaschning : Renewable Energies and Climate Protection , Munich 2013, p. 63f.
- ↑ Rizzi et al .: The production of scientific knowledge on renewable energies: Worldwide trends, dynamics and challenges and implications for management . In: Renewable Energy 62, (2014), 657-671, pp. 660f, doi: 10.1016 / j.renene.2013.08.030 .
- ↑ Gregor Czisch : Scenarios for future power supply - cost-optimized variations for supplying Europe and its neighbors with electricity from renewable energies , dissertation Kassel 2005, uni-kassel.de (PDF)
- ↑ Henrik Lund : Large-scale integration of optimal combinations of PV, wind and wave power into the electricity supply . In: Renewable Energy 31, Issue 4, (2006), 503-515, doi: 10.1016 / j.renene.2005.04.008 .
- ^ Henrik Lund : Renewable energy strategies for sustainable development . In: Energy 32, Issue 6, (2007), 912-919, doi: 10.1016 / j.energy.2006.10.017 .
- ^ Henrik Lund , Brian Vad Mathiesen : Energy system analysis of 100% renewable energy systems - The case of Denmark in years 2030 and 2050 . In: Energy 34, Issue 5, (2009), 524-531, doi: 10.1016 / j.energy.2008.04.003 .
- ↑ Olav Hohmeyer , Sönke Bohm: Trends toward 100% renewable electricity supply in Germany and Europe: a paradigm shift in energy policies . In: Wiley Interdisciplinary Reviews: Energy and Environment 4, (2015), 74–97, p. 76f, doi: 10.1002 / wene.128 .
- ^ Mark Z. Jacobson , Mark A. Delucchi: Providing all global energy with wind, water, and solar power, Part I: Technologies, energy resources, quantities and areas of infrastructure, and materials . In: Energy Policy 39, Vol. 3, (2011), 1154–1169, doi: 10.1016 / j.enpol.2010.11.040 .
- ^ Mark A. Delucchi, Mark Z. Jacobson : Providing all global energy with wind, water, and solar power, Part II: Reliability, system and transmission costs, and policies . In: Energy Policy 39, Vol. 3, (2011), 1170–1190, doi: 10.1016 / j.enpol.2010.11.045 .
- ↑ Wen Liu et al .: Potential of renewable energy systems in China . In: Applied Energy 88, Issue 2, (2011), 518-525, doi: 10.1016 / j.apenergy.2010.07.014 .
- ↑ Olav Hohmeyer , Sönke Bohm: Trends toward 100% renewable electricity supply in Germany and Europe: a paradigm shift in energy policies . In: Wiley Interdisciplinary Reviews: Energy and Environment 4, (2015), 74–97, p. 77f, doi: 10.1002 / wene.128 .
- ↑ Robert Gasch , Jochen Twele (Ed.): Wind power plants. Basics, design, planning and operation. Springer, Wiesbaden 2013, p. 6f.
- ↑ John A. Mathews, Hao Tan: Manufacture renewables to build energy security . In: Nature 513, Issue 7517, September 10, 2014, 166-168, doi: 10.1038 / 513166a .
- ↑ Peter Sheehan, Enjiang Cheng, Alex English, Fanghong Sun: China's response to the air pollution shock . In: Nature Climate Change 4, (2014), 306-309, doi: 10.1038 / nclimate2197 .
- ^ A b c Donald T. Swift-Hook: The case for renewables apart from global warming . In: Renewable Energy 49, (2013), 147–150 doi: 10.1016 / j.renene.2012.01.043 .
- ^ Achim Brunnengräber, Maria Rosaria di Nucci: Race of the systems. The starting shot for the race between fossil and renewable energies has been given - an introduction , in: Achim Brunnengräber, Maria Rosaria di Nucci (Ed.): In the hurdles for the energy transition. On transformations, reforms and innovations, Springer-Verlag 2014, ISBN 978-3-658-06788-5 . ( limited preview in Google Book search)
- ↑ Statutes (PDF) International Organization for Renewable Energies (IRENA). Retrieved May 13, 2009.
- ↑ About Us of the UN initiative “Sustainable Energy for All”.
- ↑ United Nations General Assembly Declares 2014–2024 Decade of Sustainable Energy for All. UN press release GA / 11333-EN / 274, December 21, 2012.
- ^ Pathways to Deep Decarbonization . (PDF) Sustainable Development Solutions Network . Retrieved October 15, 2014.
- ^ Tough targets (editorial). In: Nature 522, Issue 7555, (2015), 128, doi: 10.1038 / 522128a .
- ↑ Jeff Tollefson: Obama orders stronger limits on power-plant emissions . In: Nature 2015, doi: 10.1038 / nature.2015.18030 .
- ^ Jeff Tollefson, Kenneth R. Weiss: Nations approve historic global climate accord . In: Nature 2015, doi: 10.1038 / 528315a .
- ↑ Climate Pledges Will Fall Short of Needed 2 Degree C Limit . In: Scientific American , November 3, 2016. Retrieved November 6, 2016.
- ^ "More than a billion people without electricity" The time of April 3, 2017
- ↑ bundesregierung.de CO2 emissions
- ^ Krause, Bossel , Müller-Reissmann: Energiewende - growth and prosperity without crude oil and uranium , S. Fischer Verlag 1980.
- ↑ Lutz Mez : Energy Consensus in Germany? A political science analysis of the consensus talks - prerequisites, history, course and post-banter . In: Hans Günter Brauch (ed.): Energy policy. Technical development, political strategies, strategies for action on renewable energies and the rational use of energy. Berlin, Heidelberg 1997, 433-448, pp. 437f.
- ^ Rolf Wüstenhagen , Michael Bilharz: Green energy market development in Germany: Effective public policy and emerging customer demand . In: Energy Policy 34, (2006), 1681–1696, pp. 1686f, doi: 10.1016 / j.enpol.2004.07.013 .
- ^ Staffan Jacobsson, Volkmar Lauber : The politics and policy of energy system transformation — explaining the German diffusion of renewable energy technology . In: Energy Policy 34, (2006), 256-276, p. 267, doi: 10.1016 / j.enpol.2004.08.029 .
- ^ Volkmar Lauber , Staffan Jacobsson: The politics and economics of constructing, contesting and restricting socio-political space for renewables - The German Renewable Energy Act . In: Environmental Innovation and Societal Transitions 18, (2016), 147–163, doi: 10.1016 / j.eist.2015.06.005 .
- ^ Staffan Jacobsson, Volkmar Lauber : The politics and policy of energy system transformation — explaining the German diffusion of renewable energy technology . In: Energy Policy 34, (2006), 256-276, p. 269, doi: 10.1016 / j.enpol.2004.08.029 .
- ↑ Joachim Radkau , Lothar Hahn : Rise and Fall of the German Nuclear Industry , Munich 2013, p. 389.
- ↑ Joachim Radkau , Lothar Hahn : Rise and Fall of the German Nuclear Industry , Munich 2013, p. 364, ibid. Tab. P. 409.
- ↑ Alfred Voss : Guiding principles and ways of an environmentally and climate-friendly energy supply. In: Hans Günter Brauch : Energy Policy. Technical development, political strategies, concepts for action on renewable energies and the rational use of energy , Berlin / Heidelberg 1997, 59–74, pp. 64f.
- ↑ a b c Valentin Crastan : Electrical energy supply 2 , Berlin / Heidelberg 2012, p. 20.
- ↑ Matthias Eichelbrönner, Hermann Henssen: Criteria for the evaluation of future energy systems . In: Hans Günter Brauch : Energy Policy. Technical development, political strategies, concepts for action on renewable energies and the rational use of energy , Berlin / Heidelberg 1997, 461–470, p. 462.
- ↑ Jan Jonker, Wolfgang Stark, Stefan Tewes: Corporate Social Responsibility and Sustainable Development , Berlin Heidelberg 2011, pp. 145–147.
- ↑ Matthias Eichelbrönner, Hermann Henssen: Criteria for the evaluation of future energy systems . In: Hans Günter Brauch : Energy Policy. Technical development, political strategies, concepts for action on renewable energies and the rational use of energy , Berlin / Heidelberg 1997, 461–470, pp. 463–466.
- ^ Matthias Günther: Energy efficiency through renewable energies. Possibilities, potentials, systems , Wiesbaden 2015, p. 31.
- ↑ Olav Hohmeyer , Sönke Bohm: Trends toward 100% renewable electricity supply in Germany and Europe: a paradigm shift in energy policies . In: Wiley Interdisciplinary Reviews: Energy and Environment 4, (2015), 74–97, p. 75, doi: 10.1002 / wene.128 .
- ↑ Henrik Lund : Renewable Energy Systems: A Smart Energy Systems Approach to the Choice and Modeling of 100% Renewable Solutions , Academic Press 2014, p. 13.
- ↑ Ottmar Edenhofer , Susanne Kadner, Jan Minx: Is the two-degree target desirable and can it still be achieved? The contribution of science to a political debate. In: Jochem Marotzke , Martin Stratmann (Hrsg.): The future of the climate. New insights, new challenges. A report from the Max Planck Society. Beck, Munich 2015, pp. 69–92, here p. 85.
- ↑ Michael Jakob, Jerome Hilaire: Unburnable fossil-fuel reserves . In: Nature 517, (2015), 150f, doi: 10.1038 / nature14016 .
- ↑ Ottmar Edenhofer , Susanne Kadner, Jan Minx: Is the two-degree target desirable and can it still be achieved? The contribution of science to a political debate. In: Jochem Marotzke , Martin Stratmann (Hrsg.): The future of the climate. New insights, new challenges. A report from the Max Planck Society. Beck, Munich 2015, pp. 69–92, here p. 78.
- ^ Johan Rockström et al .: A roadmap for rapid decarbonization . In: Science 355, 6331, (2017), pp. 1269–1271, doi: 10.1126 / science.aah3443
- ↑ a b c Peter U. Clark et al .: Consequences of twenty-first-century policy for multi-millennial climate and sea-level change . In: Nature Climate Change 6, (2016), 360-269, doi: 10.1038 / NCLIMATE2923 .
- ↑ Carl-Jochen Winter: Energy, Entropy and Environment - How do fossil / nuclear and renewable energy systems differ. In: Hans Günter Brauch : Energy Policy. Technical development, political strategies, concepts for action on renewable energies and the rational use of energy , Berlin / Heidelberg 1997, p. 57f.
- ↑ a b Julia Schmale et al .: Clean up our skies . In: Nature 515, (2014), 335-337, doi: 10.1038 / 515335a .
- ^ Mark Z. Jacobson : Review of solutions to global warming, air pollution, and energy security . In: Energy and Environmental Science 2, (2009), 148-173, p. 149, doi: 10.1039 / b809990c .
- ↑ Nick Watts et al .: Health and climate change: policy responses to protect public health . In: The Lancet 386, No. 10006, (2015), 1861-1914, doi: 10.1016 / S0140-6736 (15) 60854-6 .
- ↑ Kailasnath B. Sutar et al .: Biomass cookstoves: A review of technical aspects . In: Renewable and Sustainable Energy Reviews 41, (2015), 1128–1166, p. 1157, doi: 10.1016 / j.rser.2014.09.003 .
- ^ Mark Z. Jacobson , Mark A. Delucchi: Providing all global energy with wind, water, and solar power, Part I: Technologies, energy resources, quantities and areas of infrastructure, and materials . In: Energy Policy 39, Vol. 3, (2011), 1154–1169, pp. 1155f, doi: 10.1016 / j.enpol.2010.11.040 .
- ↑ Jonathan Buonocore: Climate policy not so costly . In: Nature Climate Change 4, (2014), 861–862, doi: 10.1038 / nclimate2391 .
- ↑ Viktor Wesselak , Thomas Schabbach , Thomas Link, Joachim Fischer: Handbuch Regenerative Energietechnik , Berlin / Heidelberg 2017, p. 8f.
- ↑ Cf. Hermann-Josef Wagner : What are the energies of the 21st century? , Frankfurt am Main 2011, pp. 247–249.
- ^ Nicola Armaroli , Vincenzo Balzani : Energy for a Sustainable World: From the Oil Age to a Sun-Powered Future , Weinheim 2011, p. 315.
- ^ Nicola Armaroli , Vincenzo Balzani : Towards an electricity-powered world . In: Energy and Environmental Science 4, (2011), 3193-3222, p. 3218, doi: 10.1039 / c1ee01249e .
- ^ Brijesh Mainali et al .: Assessing rural energy sustainability in developing countries . In: Energy for Sustainable Development 19, (2014), 15–28, p. 15, doi: 10.1016 / j.esd.2014.01.008 .
- ↑ a b Holger Schlör et al .: The system boundaries of sustainability . In: Journal of Cleaner Production 88, (2015), 52–60, pp. 58f, doi: 10.1016 / j.jclepro.2014.04.023 .
- ^ Holger Rogall : Economics for social scientists. Introduction to sustainable economics . 2nd edition, Wiesbaden 2013, p. 128.
- ↑ Joas et al .: Which goals are driving the Energiewende? Making sense of the German Energy Transformation . In: Energy Policy 95, (2016), 42–51, doi: 10.1016 / j.enpol.2016.04.003 .
- ^ Conrad Kunze: Sociology of the energy transition . Stuttgart 2012, Ibidem, ISBN 978-3-8382-0347-8 .
- ↑ Istemi Berk, Hakan Yetkiner: Energy prices and economic growth in the long run: Theory and evidence . In: Renewable and Sustainable Energy Reviews 36, (2014) 228–235. doi: 10.1016 / j.rser.2014.04.051
- ↑ Martin Kaltschmitt , Wolfgang Streicher (Hrsgs.): Regenerative Energies in Österreich. Basics, system technology, environmental aspects, cost analyzes, potentials, use , Wiesbaden 2009, p. 616.
- ↑ Robert Gasch , Jochen Twele (Ed.): Wind power plants. Basics, design, planning and operation. Springer, Wiesbaden 2013, p. 8.
- ↑ a b Martin Kaltschmitt / Wolfgang Streicher (HRSG.): Renewable energy in Austria. Basics, system technology, environmental aspects, cost analyzes, potentials, use , Wiesbaden 2009, SV
- ↑ a b M. Johst, B. Rothstein: Reduction of cooling water consumption due to photovoltaic and wind electricity feed-in . In: Renewable and Sustainable Energy Reviews 35, (2014), 311-317. doi: 10.1016 / j.rser.2014.04.029
- ↑ Jérôme Dangerman, Hans Joachim Schellnhuber : Energy system transformation . In: Proceedings of the National Academy of Sciences 110, Issue 7 (2013), 549-558, 549, doi: 10.1073 / pnas.1219791110 .
- ↑ a b c Brian Vad Mathiesen et al .: Smart Energy Systems for coherent 100% renewable energy and transport solutions . In: Applied Energy 145, (2015), 139–154, p. 140, doi: 10.1016 / j.apenergy.2015.01.075 .
- ^ Matthias Heymann : The history of the use of wind energy 1890-1990. Frankfurt am Main - New York 1995, p. 343.
- ↑ Alois Schaffarczyk (Ed.): Introduction to wind energy technology. Munich 2012, p. 45.
- ↑ a b Volker Quaschning : System technology for a climate-friendly energy supply in Germany for the 21st century , Düsseldorf 2000, p. 14.
- ↑ Hans Günter Brauch : Energy policy under the sign of climate policy in the transition to the 21st century. In: Ders .: Energy Policy. Technical development, political strategies, strategies for action on renewable energies and the rational use of energy. Berlin / Heidelberg 1997, 1-24, p. 2.
- ^ Robert C. Allen : The British Industrial Revolution in Global Perspective , Cambridge University Press, 2009, p. 88.
- ^ Edgar G. Hertwich et al .: Integrated life-cycle assessment of electricity-supply scenarios confirms global environmental benefit of low-carbon technologies . In: Proceedings of the National Academy of Sciences 112, No. 20, (2015), 6277-6282, doi: 10.1073 / pnas.1312753111
- ↑ Cf. Volker Quaschning : Regenerative Energy Systems. Technology - calculation - simulation . Munich 2011, p. 31.
- ↑ Environmental changes in mining regions (PDF; 247 kB). Retrieved March 1, 2012.
- ↑ a b S. M. Moosavian, NA Rahim, J. Selvaraj, KH Solangi: Energy policy to promote photovoltaic generation . In: Renewable and Sustainable Energy Reviews 25, (2013), 44–58, p. 45, doi: 10.1016 / j.rser.2013.03.030 .
- ↑ Wouter Poortinga, Alexa Spence, Lorraine Whitmarsh, Stuart Capstick, Nick F. Pidgeon Uncertain climate: An investigation into public skepticism about anthropogenic climate change . In: Global Environmental Change 21, (2011), 1015-1024, 1015, doi: 10.1016 / j.gloenvcha.2011.03.001 .
- ↑ a b IPCC 2014: Climate Change 2014: Synthesis Report. Contribution of Working Groups I, II and III to the Fifth Assessment Report of the Intergovernmental Panel on Climate Change [Core Writing Team, RK Pachauri and LA Meyer (eds.)]. IPCC, Geneva, Switzerland, 151 S., ipcc.ch (PDF) Retrieved on May 20, 2016.
- ↑ Valentin Crastan : Electrical energy supply 2 . Berlin / Heidelberg 2012, p. 11.
- ↑ Volker Quaschning , Regenerative Energy Systems. Technology - calculation - simulation. 9th updated edition. Munich 2015, p. 44.
- ↑ DR Feldman et al .: Observational determination of surface radiative forcing by CO2 from 2000 to 2010 . In: Nature 519, (2015), 339-343, doi: 10.1038 / nature14240 .
- ↑ Alain Goeppert et al .: Air as the renewable carbon source of the future: an overview of CO2 capture from the atmosphere . In: Energy and Environmental Science 5, (2012), 7833-7853, doi: 10.1039 / c2ee21586a .
- ^ Mark C. Urban: Accelerating extinction risk from climate change . In: Science 348, Issue 6234, (2015), 571-573, doi: 10.1126 / science.aaa4984 .
- ↑ Valentin Crastan : Electrical energy supply 2 , Berlin / Heidelberg 2012, p. 19f.
- ↑ Hermann-Josef Wagner : What are the energies of the 21st century? , Frankfurt am Main 2011, p. 180.
- ^ Klaus Heuck / Klaus-Dieter Dettmann / Detlef Schulz: Electrical energy supply. Generation, transmission and electrical energy for study and practice , 9th revised and updated edition. Wiesbaden 2013, p. 60.
- ^ Nicola Armaroli , Vincenzo Balzani : Towards an electricity-powered world . In: Energy and Environmental Science 4, (2011), 3193-3222 doi: 10.1039 / c1ee01249e .
- ↑ Robert Schlögl : Learning from nature. Chemical reduction of CO 2 . In: Jochem Marotzke , Martin Stratmann (Hrsg.): The future of the climate. New insights, new challenges. A report from the Max Planck Society. Beck, Munich 2015, pp. 167-182, p. 170; Felix Ekardt : Theory of Sustainability Baden-Baden 2011, p. 383 .; Matthias Eichelbrönner, Hermann Henssen: Criteria for the evaluation of future energy systems . In: Hans Günter Brauch : Energy Policy. Technical development, political strategies, concepts for action on renewable energies and the rational use of energy , Berlin / Heidelberg 1997, 461–470, p. 461.
- ↑ Cf. Felix Ekardt : Theory of Sustainability Baden-Baden 2011, p. 379f.
- ^ Joachim Radkau : Technology and Environment . In: Gerold Ambrosius , Dietmar Petzina , Werner Plumpe (eds.): Modern economic history. An introduction for historians and economists , Munich 2006, 135–154, p. 143.
- ^ Rolf Peter Sieferle : The pre-industrial solar energy system. In: Hans Günter Brauch : Energy Policy. Technical development, political strategies, concepts for action on renewable energies and the rational use of energy , Berlin / Heidelberg 1997, 27–46, pp. 27f.
- ^ Edward Anthony Wrigley : Energy and the English Industrial Revolution , Cambridge University Press 2010, pp. 247f.
- ^ Mark Z. Jacobson : Review of solutions to global warming, air pollution, and energy security . In: Energy and Environmental Science 2, (2009), 148-173, pp. 149f, doi: 10.1039 / b809990c .
- ↑ Cf. Stefan Lars, Thoren Reun, Friederike Anna Dratwa: In varietate concordia - strategies and goals of the “new” EU energy and climate policy . In: Friederike Anna Dratwa et al. (Ed.): Energy industry in Europe. In the field of tension between climate policy, competition and security of supply , Berlin Heidelberg 2010, pp. 101–130, esp. 105–108.
- ↑ Germany - Raw Materials Situation 2013 (PDF) Federal Institute for Geosciences and Raw Materials . Retrieved February 6, 2015.
- ↑ Energy consumption in Germany in 2015 . AG energy balances. Retrieved August 6, 2016.
- ^ Matthias Günther: Energy efficiency through renewable energies. Possibilities, potentials, systems , Wiesbaden 2015, p. 182.
- ↑ Holger Rogall : 100% supply with renewable energies. Conditions for global, national and local implementation . Marburg 2014, pp. 200–202.
- ↑ Robert Stieglitz, Volker Heinzel: Thermal solar energy. Basics, technology, applications. Berlin Heidelberg 2012, p. 6.
- ↑ Viktor Wesselak , Thomas Schabbach , Thomas Link, Joachim Fischer: Handbuch Regenerative Energietechnik , Berlin / Heidelberg 2017, pp. 32–34.
- ^ A b Klaus Heuck / Klaus-Dieter Dettmann / Detlef Schulz: Electrical energy supply. Generation, transmission and electrical energy for study and practice , 9th revised and updated edition. Wiesbaden 2013, pp. 60f.
- ↑ Volker Quaschning : Regenerative Energy Systems. Technology - calculation - simulation . 9th updated edition, Munich 2015, pp. 30–33.
- ^ A b Nicola Armaroli , Vincenzo Balzani : Towards an electricity-powered world . In: Energy and Environmental Science 4, (2011), 3193-3222, 3216, doi: 10.1039 / c1ee01249e .
- ^ A b c Nicola Armaroli , Vincenzo Balzani : Towards an electricity-powered world . In: Energy and Environmental Science 4, (2011), 3193-3222, 3200f, doi: 10.1039 / c1ee01249e .
- ↑ The World Nuclear Industry Status Report 2014 (PDF) Retrieved March 4, 2015.
- ↑ Michael Dittmar: Nuclear energy: Status and future limitations . In: Energy , 37, (2012), pp. 35-40, here p. 35, doi: 10.1016 / j.energy.2011.05.040 .
- ^ Mark Z. Jacobson : Review of solutions to global warming, air pollution, and energy security . In: Energy and Environmental Science 2, (2009), 148-173, p. 155, doi: 10.1039 / b809990c .
- ^ Mark Z. Jacobson ; Mark A. Delucchi: Providing all global energy with wind, water, and solar power, Part I: Technologies, energy resources, quantities and areas of infrastructure, and materials . In: Energy Policy 39, Vol. 3, (2011), 1154–1169, p. 1156, doi: 10.1016 / j.enpol.2010.11.040 .
- ^ Nicola Armaroli , Vincenzo Balzani : Towards an electricity-powered world . In: Energy and Environmental Science 4, (2011), 3193-3222, p. 3216, doi: 10.1039 / c1ee01249e .
- ↑ Ottmar Edenhofer , Susanne Kadner, Jan Minx: Is the two-degree target desirable and can it still be achieved? The contribution of science to a political debate. In: Jochem Marotzke , Martin Stratmann (Hrsg.): The future of the climate. New insights, new challenges. A report from the Max Planck Society. Beck, Munich 2015, pp. 69–92, here p. 90f.
- ↑ See Hermann Scheer : Der energethische Imperativ , Munich 2010, p. 14 f.
- ↑ Florian Lüdeke-Freund, Oliver Opel: Energy . In: Harald Heinrichs , Gerd Michelsen (ed.): Sustainability Sciences, Berlin / Heidelberg 2014, p. 434.
- ^ David Connolly et al .: Smart Energy Europe: The technical and economic impact of one potential 100% renewable energy scenario for the European Union . In: Renewable and Sustainable Energy Reviews 60, (2016), 1634–1653, doi: 10.1016 / j.rser.2016.02.025 .
- ↑ Volker Quaschning : Regenerative Energy Systems. Technology - calculation - simulation . 9th updated edition. Munich 2015, p. 34.
- ↑ Cf. Martin Kaltschmitt , Wolfgang Streicher, Andreas Wiese (eds.): Renewable energies. System technology, economic efficiency, environmental aspects , Berlin Heidelberg 2013, p. 5.
- ^ A b Stefan Weitemeyer, David Kleinhans, Thomas Vogt, Carsten Agert: Integration of Renewable Energy Sources in future power systems: The role of storage . In: Renewable Energy 75, (2015), 14–20, p. 14, doi: 10.1016 / j.renene.2014.09.028 .
- ↑ Sarah Becker et al .: Features of a fully renewable US electricity system: Optimized mixes of wind and solar PV and transmission grid extensions . In: Energy 72, (2014), 443–458, p. 443, doi: 10.1016 / j.energy.2014.05.067 .
- ^ Mark Z. Jacobson , Mark A. Delucchi: Providing all global energy with wind, water, and solar power, Part I: Technologies, energy resources, quantities and areas of infrastructure, and materials . In: Energy Policy 39, (2011), 1154–1169, doi: 10.1016 / j.enpol.2010.11.040 .
- ↑ DF Dominkovic et al., Zero carbon energy system of South East Europe in 2050 . In: Applied Energy 184, (2016), 1517-1528, doi: 10.1016 / j.apenergy.2016.03.046
- ↑ Holger Rogall : 100% supply with renewable energies. Conditions for global, national and local implementation . Marburg 2014, p. 217.
- ↑ Omar Ellabban, Haitham Abu-Rub, Frede Blaabjerg: Renewable energy resources: Current status, future prospects and Their enabling technology . In: Renewable and Sustainable Energy Reviews 39, (2014), 748–764, p. 763, doi: 10.1016 / j.rser.2014.07.113 .
- ↑ Francesco Asdrubali, Giorgio Baldinelli, Francesco D'Alessandro, Flavio Scrucca: Life cycle assessment of electricity production from renewable energies: Review and results harmonization. In: Renewable and Sustainable Energy Reviews 42, (2015), 1113–1122, doi: 10.1016 / j.rser.2014.10.082 .
- ↑ Christian Breyer et al .: Profitable climate change mitigation: The case of greenhouse gas emission reduction benefits enabled by solar photovoltaic systems . In: Renewable and Sustainable Energy Reviews 49, (2015), 610–628, 611, doi: 10.1016 / j.rser.2015.04.061 .
- ↑ Global Status Report 2017 . REN21 website . Retrieved July 26, 2017.
- ↑ Viktor Wesselak , Thomas Schabbach , Thomas Link, Joachim Fischer: Handbuch Regenerative Energietechnik , Berlin / Heidelberg 2017, p. 37.
- ↑ a b Hermann-Josef Wagner : What are the energies of the 21st century? , Frankfurt am Main 2011, pp. 195f.
- ^ Nicola Armaroli , Vincenzo Balzani : Towards an electricity-powered world . In: Energy and Environmental Science 4, (2011), 3193-3222, p. 3213 doi: 10.1039 / c1ee01249e .
- ↑ a b Ursula Eicker : Solar technologies for buildings. Basics and practical examples , Wiesbaden 2012, pp. 1–4.
- ↑ Volker Quaschning : Regenerative Energy Systems. Technology - calculation - simulation . 9th updated edition. Munich 2015, p. 55.
- ↑ Martin Pehnt (Ed.): Energy efficiency. A teaching and manual . Berlin Heidelberg 2010, p. 6.
- ↑ Cf. Viktor Wesselak , Thomas Schabbach , Thomas Link, Joachim Fischer: Handbuch Regenerative Energietechnik , Berlin / Heidelberg 2017, p. 38f .; Felix Ekardt : The task of the energy transition of the century: A manual. Berlin 2014, chap. II.
- ↑ a b Hermann-Josef Wagner : What are the energies of the 21st century? , Frankfurt am Main 2011, pp. 199f.
- ↑ Ursula Eicker : Solar technologies for buildings. Basics and practical examples , Wiesbaden 2012, p. 4.
- ^ Henrik Lund et al .: Smart energy and smart energy systems . In: Energy . tape 137 , 2017, p. 556-565 , doi : 10.1016 / j.energy.2017.05.123 .
- ^ Nicola Armaroli , Vincenzo Balzani : Towards an electricity-powered world . In: Energy and Environmental Science 4, (2011), 3193-3222, doi: 10.1039 / c1ee01249e .
- ↑ James H. Williams et al .: The Technology Path to Deep Greenhouse Gas Emissions Cuts by 2050: The Pivotal Role of Electricity . Science 335, 2012, doi: 10.1126 / science.1208365 .
- ↑ Holger Rogall : 100% supply with renewable energies. Conditions for global, national and local implementation . Marburg 2014, p. 179.
- ^ Matthias Günther: Energy efficiency through renewable energies. Opportunities, Potentials, Systems , Wiesbaden 2015, pp. 103–113, esp. 103.
- ^ Benjamin K. Sovacool et al .: Tempering the Promise of Electric Mobility? A Sociotechnical Review and Research Agenda for Vehicle-Grid Integration (VGI) and Vehicle-to-Grid (V2G) . In: Annual Review of Environment and Resources . tape 42 , 2017, p. 377-406 , doi : 10.1146 / annurev-environ-030117-020220 .
- ^ Hans-Martin Henning, Andreas Palzer: A comprehensive model for the German electricity and heat sector in a future energy system with a dominant contribution from renewable energy technologies — Part I: Methodology. In: Renewable and Sustainable Energy Reviews 30, (2014), 1003-1018, p. 1004, doi: 10.1016 / j.rser.2013.09.012
- ↑ Cf. Volker Quaschning : Regenerative Energy Systems. Technology - calculation - simulation . 9th updated edition. Munich 2015, p. 52f.
- ^ Mark Z. Jacobson , Mark A. Delucchi: Providing all global energy with wind, water, and solar power, Part I: Technologies, energy resources, quantities and areas of infrastructure, and materials . In: Energy Policy 39, Vol. 3, (2011), 1154–1169, p. 1159, doi: 10.1016 / j.enpol.2010.11.040 .
- ^ Brian Vad Mathiesen et al .: Smart Energy Systems for coherent 100% renewable energy and transport solutions . In: Applied Energy 145, (2015), 139–154, 149f, doi: 10.1016 / j.apenergy.2015.01.075 .
- ^ Iain Staffell, Dan Brett, Nigel Brandon, Adam Hawkes: A review of domestic heat pumps . In: Energy and Environmental Science 5, (2012), 9291-9306, doi: 10.1039 / c2ee22653g .
- ↑ Valentin Crastan : Electrical energy supply 2 , Berlin / Heidelberg 2012, p. 359.
- ^ Mathias Günther: Energy efficiency through renewable energies. Possibilities, potentials, systems , Wiesbaden 2014, p. 111.
- ↑ Carvalho et al .: Ground source heat pump carbon emissions and primary energy reduction potential for heating in buildings in Europe - results of a case study in Portugal . In: Renewable and Sustainable Energy Reviews , 45, (2015), pp. 755–768, here p. 767, doi: 10.1016 / j.rser.2015.02.034 .
- ^ A b André Sternberg, André Bardow: Power-to-What? - Environmental assessment of energy storage systems . In: Energy and Environmental Science , 8, (2015), pp. 389-400, here pp. 398 f., Doi: 10.1039 / c4ee03051f .
- ↑ Andreas Bloess, Wolf-Peter Schill, Alexander Zerrahn: Power-to-heat for renewable energy integration: A review of technologies, modeling approaches, and flexibility potentials . In: Applied Energy . tape 212 , 2018, p. 1611-1626 , doi : 10.1016 / j.apenergy.2017.12.073 .
- ↑ Carvalho et al .: Ground source heat pump carbon emissions and primary energy reduction potential for heating in buildings in Europe - results of a case study in Portugal . In: Renewable and Sustainable Energy Reviews 45, (2015), 755–768, p. 767, doi: 10.1016 / j.rser.2015.02.034 .
- ^ Aymeric Girard et al .: Higher ground source heat pump COP in a residential building through the use of solar thermal collectors . In: Renewable Energy 80, (2015), 26–39, doi: 10.1016 / j.renene.2015.01.063 .
- ^ Matthias Günther: Energy efficiency through renewable energies. Possibilities, potentials, systems . Wiesbaden 2015, p. 103; Ibid. P. 110.
- ^ Abdul Rehman Mazhar et al .: A state of art review on district heating systems . In: Renewable and Sustainable Energy Reviews . tape 96 , 2018, p. 420-439 , doi : 10.1016 / j.rser.2018.08.005 .
- ↑ Henrik Lund et al .: 4th Generation District Heating (4GDH) Integrating smart thermal grids into future sustainable energy systems . In: Energy . tape 68 , 2014, p. 1–11 , doi : 10.1016 / j.energy.2014.02.089 .
- ^ Brian Vad Mathiesen et al .: Smart Energy Systems for coherent 100% renewable energy and transport solutions . In: Applied Energy , 145, (2015), pp. 139–154, here p. 144, doi: 10.1016 / j.apenergy.2015.01.075 .
- ↑ Henrik Lund : Renewable Energy Systems: A Smart Energy Systems Approach to the Choice and Modeling of 100% Renewable Solutions , Academic Press 2014, chapter 5, in particular p. 96.
- ^ MA Sayegh et al .: Heat pump placement, connection and operational modes in European district heating . In: Energy and Buildings . tape 166 , 2018, p. 122-144 , doi : 10.1016 / j.enbuild.2018.02.006 .
- ^ Andrei David et al .: Heat Roadmap Europe: Large-Scale Electric Heat Pumps in District Heating Systems . In: Energies . tape 10 , no. 4 , 2017, p. 578 ff ., doi : 10.3390 / en10040578 .
- ↑ Weert Canzler , Dirk Wittowsky: The impact of Germany's energy policy on the transport sector - Unsolved problems and conflicts . In: Utilities Policy 41, (2016), pp. 246-251, doi: 10.1016 / j.jup.2016.02.011
- ↑ a b Brian Vad Mathiesen et al .: Smart Energy Systems for coherent 100% renewable energy and transport solutions . In: Applied Energy 145, (2015), 139–154, p. 147, doi: 10.1016 / j.apenergy.2015.01.075 .
- ^ Nicola Armaroli , Vincenzo Balzani : Towards an electricity-powered world . In: Energy and Environmental Science 4, (2011), 3193-3222, 3214, doi: 10.1039 / c1ee01249e .
- ↑ a b Brian Vad Mathiesen et al .: Smart Energy Systems for coherent 100% renewable energy and transport solutions . In: Applied Energy 145, (2015), 139–154, p. 145, doi: 10.1016 / j.apenergy.2015.01.075 .
- ^ Dunn et al .: The significance of Li-ion batteries in electric vehicle life-cycle energy and emissions and recycling's role in its reduction . In: Energy and Environmental Science 8, (2015), 158–168, 166f, doi: 10.1039 / c4ee03029j .
- ^ A b Drew T. Shindell : The social cost of atmospheric release . In: Climatic Change 130, (2015), 313–326, doi: 10.1007 / s10584-015-1343-0 .
- ^ Alberto Moro, Eckard Helmers: A new hybrid method for reducing the gap between WTW and LCA in the carbon footprint assessment of electric vehicles . In: The International Journal of Life Cycle Assessment 22, Issue 1, (2017), 4–14, doi: 10.1007 / s11367-015-0954-z .
- ^ A b Alberto Varone, Michele Ferrari: Power to liquid and power to gas: An option for the German Energiewende . In: Renewable and Sustainable Energy Reviews 45, (2015), 207–218, p. 208, doi: 10.1016 / j.rser.2015.01.049 .
- ^ Mark Z. Jacobson et al .: 100% clean and renewable wind, water, and sunlight (WWS) all-sector energy roadmaps for the 50 United States . In: Energy and Environmental Science 8, (2015), 2093-2117, p. 2095, doi: 10.1039 / c5ee01283j .
- ↑ Siang Fui Tie, Chee Wei Tan: A review of energy sources and energy management system in electric vehicles . In: Renewable and Sustainable Energy Reviews 20, (2013), 82-102, pp. 89f, doi: 10.1016 / j.rser.2012.11.077 .
- ^ Nicola Armaroli , Vincenzo Balzani : Towards an electricity-powered world . In: Energy and Environmental Science 4, (2011), 3193-3222, p. 3218, doi: 10.1039 / c1ee01249e .
- ^ Nicola Armaroli , Vincenzo Balzani : Towards an electricity-powered world . In: Energy and Environmental Science 4, (2011), 3193-3222, p. 3215, doi: 10.1039 / c1ee01249e .
- ^ David B. Richardson: Electric vehicles and the electric grid: A review of modeling approaches, Impacts, and renewable energy integration . In: Renewable and Sustainable Energy Reviews 19, (2013), 247-254, 252f, doi: 10.1016 / j.rser.2012.11.042 .
- ↑ a b Henrik Lund et al .: From electricity smart grids to smart energy systems - A market operation based approach and understanding . In: Energy 42, Issue 1, (2012) 96-102, p. 96, doi: 10.1016 / j.energy.2012.04.003 .
- ↑ a b c d e f g Henning et al .: Phases of the transformation of the energy system . In: Energiewirtschaftliche Tagesfragen 65, Heft 1/2, (2015), pp. 10–13.
- ↑ a b c d Manfred Fischedick , What must the transformation phases of the energy system look like? . In: Renewable Energies. Das Magazin , October 28, 2014. Retrieved March 1, 2015.
- ↑ Volker Quaschning : Regenerative Energy Systems. Technology - calculation - simulation . 9th updated edition. Munich 2015, p. 52f.
- ↑ Panos Konstantin: Praxishandbuch Energiewirtschaft. Energy conversion, transport and procurement in the liberalized market. Berlin / Heidelberg 2013, p. 384.
- ↑ Henrik Lund et al .: From electricity smart grids to smart energy systems - A market operation based approach and understanding . In: Energy 42, Issue 1, (2012) 96-102, p. 97, doi: 10.1016 / j.energy.2012.04.003 .
- ^ Nicola Armaroli , Vincenzo Balzani : Towards an electricity-powered world . In: Energy and Environmental Science 4, (2011), 3193-3222, p. 3216, doi: 10.1039 / c1ee01249e .
- ^ Mark Z. Jacobson : Review of solutions to global warming, air pollution, and energy security . In: Energy and Environmental Science 2, (2009), 148-173, p. 170, doi: 10.1039 / b809990c .
- ↑ Volker Quaschning : System technology for a climate-friendly energy supply in Germany for the 21st century , Düsseldorf 2000, p. 150.
- ↑ Cf. Hermann-Josef Wagner : What are the energies of the 21st century? , Frankfurt am Main 2011, pp. 114f.
- ↑ Robert Gasch , Jochen Twele (Ed.): Wind power plants. Basics, design, planning and operation. Springer, Wiesbaden 2013, p. 484f.
- ↑ Hermann-Josef Wagner : What are the energies of the 21st century? , Frankfurt am Main 2011, p. 117.
- ^ Matthias Günther: Energy efficiency through renewable energies. Possibilities, potentials, systems , Wiesbaden 2015, p. 143f.
- ↑ Sarah Becker et al .: Transmission grid extensions during the build-up of a fully renewable pan-European electricity supply . In: Energy 64, (2014), 404-418, doi: 10.1016 / j.energy.2013.10.010 .
- ↑ a b Michael Sterner , Ingo Stadler (ed.): Energy storage - requirements, technologies, integration . Berlin - Heidelberg 2017, p. 101.
- ↑ Holger Rogall : 100% supply with renewable energies. Conditions for global, national and local implementation . Marburg 2014, p. 186.
- ↑ a b Spyros Chatzivasileiadis, Damien Ernst, Göran Andersson: The Global Grid . In: Renewable Energy 57, (2013), 372-383, doi: 10.1016 / j.renene.2013.01.032 .
- ↑ Volker Quaschning : Regenerative Energy Systems. Technology - calculation - simulation . 9th updated edition. Munich 2015, p. 176.
- ↑ Spyros Chatzivasileiadis, Damien Ernst, Göran Andersson: The Global Grid . In: Renewable Energy 57, (2013), 372-383, p. 376, doi: 10.1016 / j.renene.2013.01.032 .
- ↑ Henrik Lund et al .: From electricity smart grids to smart energy systems - A market operation based approach and understanding . In: Energy 42, Issue 1, (2012) 96-102, pp. 97f, doi: 10.1016 / j.energy.2012.04.003 .
- ↑ Martin Stötzer et al .: Potential of demand side integration to maximize use of renewable energy sources in Germany . In: Applied Energy 146, (2015), 344-352, p. 350, doi: 10.1016 / j.apenergy.2015.02.015 .
- ^ Peter D. Lund: Clean energy systems as mainstream energy options . In: International Journal of Energy Research 40, (2016), 4–12, doi: 10.1002 / er.3283 .
- ↑ a b Mark Z. Jacobson et al. Low-cost solution to the grid reliability problem with 100% penetration of intermittent wind, water, and solar for all purposes . In: Proceedings of the National Academy of Sciences 112, No. 49, (2015), 15060-15065, doi: 10.1073 / pnas.1510028112 .
- ↑ See Michael Sterner , Ingo Stadler (Hrsg.): Energy storage - requirements, technologies, integration . Berlin - Heidelberg 2017, p. 579f.
- ^ Brian Vad Mathiesen et al .: Smart Energy Systems for coherent 100% renewable energy and transport solutions . In: Applied Energy 145, (2015), 139–154, doi: 10.1016 / j.apenergy.2015.01.075 .
- ↑ Michael Sterner , Ingo Stadler (ed.): Energy storage - demand, technologies, integration . Berlin - Heidelberg 2017, p. 677.
- ↑ Holger Rogall : 100% supply with renewable energies. Conditions for global, national and local implementation . Marburg 2014, p. 193.
- ^ Weert Canzler , Andreas Knie : Smart networks. How the energy and transport turnaround succeeds . Munich 2013, p. 47.
- ↑ A. Moser, N. Red Ring, W. Wellßow, H. Pluntke: Additional needs for storing 2020 at the earliest . In: Elektrotechnik & Informationstechnik 130, (2013) 75–80, pp. 77–79. doi: 10.1007 / s00502-013-0136-2
- ↑ Michael Sterner , Ingo Stadler (ed.): Energy storage - demand, technologies, integration . Berlin - Heidelberg 2017, p. 59.
- ↑ Stefan Weitemeyer, David Kleinhans, Thomas Vogt, Carsten Agert: Integration of Renewable Energy Sources in future power systems: The role of storage . In: Renewable Energy 75, (2015), 14-20, doi: 10.1016 / j.renene.2014.09.028 .
- ↑ Michael Sterner , Ingo Stadler (ed.): Energy storage - demand, technologies, integration . Berlin - Heidelberg 2017, p. 465.
- ^ André Sternberg, André Bardow: Power-to-What? - Environmental assessment of energy storage systems . In: Energy and Environmental Science 8, (2015), 389-400, pp. 389f, doi: 10.1039 / c4ee03051f .
- ↑ Veronica Palomares et al .: Na-ion batteries, recent advances and present challenges to become low cost energy storage systems . In: Energy and Environmental Science 5, (2012), 5884-5901, doi: 10.1039 / c2ee02781j .
- ↑ Huilin Pan et al .: Room-temperature stationary sodium-ion batteries for large-scale electric energy storage . In: Energy and Environmental Science 6, (2013), 2338-2360, doi: 10.1039 / c3ee40847g .
- ↑ Brian Huskinson et al .: AMETAL-free organic - inorganic wässrige flow battery . In: Nature 505, (2014), 195-198, doi: 10.1038 / nature12909 .
- ↑ Meng-Chang Lin et al .: An ultrafast rechargeable aluminum-ion battery . In: Nature 520, (2015), 324-328, doi: 10.1038 / nature14340
- ^ Matthias Heymann : The history of the use of wind energy 1890-1990. Frankfurt am Main / New York 1995, pp. 64-66.
- ↑ Volker Quaschning : Regenerative Energy Systems. Technology - calculation - simulation . 9th updated edition. Munich 2015, p. 393.
- ↑ Günther Brauner: Energy systems: regenerative and decentralized. Strategies for the energy transition . Wiesbaden 2016, p. 89.
- ↑ Jensen et al .: Large-scale electricity storage utilizing reversible solid oxide cells combined with underground storage of CO2 and CH4 . In: Energy and Environmental Science 8, (2015), 2471-2479, doi: 10.1039 / c5ee01485a .
- ^ Zhan Gao et al .: A perspective on low-temperature solid oxide fuel cells . In: Energy and Environmental Science 9, (2016), 1602–1644, doi: 10.1039 / c5ee03858h .
- ^ Andreas Palzer, Hans-Martin Henning: A comprehensive model for the German electricity and heat sector in a future energy system with a dominant contribution from renewable energy technologies — Part II: Results. In: Renewable and Sustainable Energy Reviews 30, (2014), 1019-1034, doi: 10.1016 / j.rser.2013.11.032 .
- ^ A b c Hans-Andreas Palzer, Martin Henning: A comprehensive model for the German electricity and heat sector in a future energy system with a dominant contribution from renewable energy technologies — Part II: Results. In: Renewable and Sustainable Energy Reviews 30, (2014), 1019-1034, doi: 10.1016 / j.rser.2013.11.032 .
- ↑ a b Sebastian Strunz: The German energy transition as a regime shift . In: Ecological Economics 100, (2014), 150–158, p. 154, doi: 10.1016 / j.ecolecon.2014.01.019
- ^ Staffan Jacobsson, Volkmar Lauber : The politics and policy of energy system transformation — explaining the German diffusion of renewable energy technology . In: Energy Policy 34, (2006), 256-276, pp. 261f, doi: 10.1016 / j.enpol.2004.08.029 .
- ^ Sebastian Strunz: The German energy transition as a regime shift . In: Ecological Economics 100, (2014), 150–158, p. 152, doi: 10.1016 / j.ecolecon.2014.01.019
- ↑ a b Volker Quaschning : Regenerative Energy Systems. Technology - calculation - simulation . 9th updated edition. Munich 2015, p. 57.
- ↑ Dörte Ohlhorst, Kerstin Tews, Miranda Schreurs: The energy transition as a challenge to coordination in a multi-level system . In: Achim Brunnengräber , Maria Rosaria du Nucci (Ed.): In the hurdles for the energy transition. About transformations, reforms and innovations. On the occasion of Lutz Mez's 70th birthday , Wiesbaden 2014, 93-104, p. 94.
- ↑ a b Sebastian Strunz: The German energy transition as a regime shift . In: Ecological Economics 100, (2014), 150–158, pp. 154f, doi: 10.1016 / j.ecolecon.2014.01.019
- ↑ Reader's note: The basic idea of the energy transition is absolutely nonsensical ; at solarify.eu
- ↑ "The basic idea of the energy transition is absolutely nonsensical" ; at welt.de
- ↑ Volker Quaschning : System technology for a climate-friendly electricity supply in Germany for the 21st century . Düsseldorf 2000, p. 39.
- ↑ Viktor Wesselak , Thomas Schabbach : Regenerative Energietechnik , Berlin Heidelberg 2009, p. 419f.
- ↑ Alois Schaffarczyk (Ed.): Introduction to wind energy technology. Munich 2012, p. 135.
- ↑ See Peter Hennicke , Manfred Fischedick : Renewable energies. With energy efficiency for the energy transition , Munich 2007, p. 17.
- ↑ Omid Palizban, Kimmo Kauhaniemi, Josep M. Guerrero: Micro grids in active network management - Part I: Hierarchical control, energy storage, virtual power plants, and market participation . In: Renewable and Sustainable Energy Reviews 36, (2014), 428–439, doi: 10.1016 / j.rser.2014.01.016 .
- ^ Jürgen Karl: Decentralized energy systems. New technologies in the liberalized energy market , Munich 2012, p. 14f.
- ^ Peter Hennicke , Manfred Fischedick : Renewable energies. With energy efficiency for the energy transition , Munich 2007, p. 16.
- ↑ Bene Müller: Renewable Energies and Regional Value Creation . In: Friederike Anna Dratwa et al. (Ed.): Energy industry in Europe. In the field of tension between climate policy, competition and security of supply , Berlin Heidelberg 2010, pp. 47–51.
- ↑ Cf. Alois Schaffarczyk (ed.): Introduction to wind energy technology. Munich 2012, pp. 69–72.
- ↑ Xue Zhang et al .: Emerging smart grid technology for mitigating global warming . In: International Journal of Energy Research 39, Issue 13, (2015), 1742–1756, doi: 10.1002 / er.3296 .
- ^ Mark A. Delucchi, Mark Z. Jacobson : Providing all global energy with wind, water, and solar power, Part II: Reliability, system and transmission costs, and policies . In: Energy Policy 39, Vol. 3, (2011), 1170–1190, doi: 10.1016 / j.enpol.2010.11.045 .
- ^ Nicola Armaroli , Vincenzo Balzani : Towards an electricity-powered world . In: Energy and Environmental Science 4, (2011), 3193-3222, p. 3203 doi: 10.1039 / c1ee01249e .
- ↑ Panos Konstantin: Praxishandbuch Energiewirtschaft. Energy conversion, transport and procurement in the liberalized market . Berlin / Heidelberg 2013, p. 332.
- ↑ Federal Environment Agency 2007, cited. based on: Viktor Wesselak , Thomas Schabbach , Thomas Link, Joachim Fischer: Handbuch Regenerative Energietechnik , Berlin / Heidelberg 2017, p. 31.
- ↑ Viktor Wesselak , Thomas Schabbach , Thomas Link, Joachim Fischer: Handbuch Regenerative Energietechnik , Berlin / Heidelberg 2017, pp. 29–31.
- ^ Nicola Armaroli , Vincenzo Balzani : Towards an electricity-powered world . In: Energy and Environmental Science 4, (2011), 3193-3222, p. 3195, doi: 10.1039 / c1ee01249e .
- ↑ Ben Machol, Sarah Rizk: Economic value of US fossil fuel electricity health impacts . In: Environment International 52, (2013), 75–80, p. 78, doi: 10.1016 / j.envint.2012.03.003 .
- ^ Mark Z. Jacobson et al .: 100% clean and renewable wind, water, and sunlight (WWS) all-sector energy roadmaps for the 50 United States . In: Energy and Environmental Science 8, (2015), 2093-2117, p. 2115, doi: 10.1039 / c5ee01283j .
- ↑ Kirsten Selder: Renewable Energy Sources Act and Trading of Emission Certificates: A national and a supranational tool direct energy turnover to renewable electricity-supply in Germany . In: Energy Policy 64, 2014, 302-312, p. 305, doi: 10.1016 / j.enpol.2013.08.030 .
- ↑ Viktor Wesselak , Thomas Schabbach , Thomas Link, Joachim Fischer: Handbuch Regenerative Energietechnik , Berlin / Heidelberg 2017, p. 31.
- ↑ Florian Lüdeke-Freund, Oliver Opel: Energy . In: Harald Heinrichs , Gerd Michelsen (ed.): Sustainability Sciences, Berlin / Heidelberg 2014, p. 442.
- ↑ Valentin Crastan : Electrical energy supply 2. Berlin / Heidelberg 2012, p. 87.
- ↑ Valentin Crastan : Electrical energy supply 2 , Berlin / Heidelberg 2012, p. 88.
- ^ AG Energiebilanzen : Energy consumption in Germany in 2013. p. 41, accessed on April 9, 2014.
- ↑ Jonathan M. Harris, Brian Roach: Environmental and Natural Resource Economics. A Contemporary Approach , Routledge 2014, p. 303.
- ↑ Zhujun Jiang, Boqiang Lin: The perverse fossil fuel subsidies in China. The scale and effects . In: Energy 70, (2014), 411-419, pp. 411f, doi: 10.1016 / j.energy.2014.04.010 .
- ↑ How Large Are Global Energy Subsidies? (PDF) Internet site of the International Monetary Fund . Retrieved May 19, 2015.
- ^ David Coady et al .: How Large Are Global Fossil Fuel Subsidies? In: World Development . tape 91 , 2017, p. 11-27 , doi : 10.1016 / j.worlddev.2016.10.004 .
- ^ Ottmar Edenhofer : King Coal and the queen of subsidies . In: Science 349, Issue 6254, (2015), 1286f, doi: 10.1126 / science.aad0674 .
- ↑ a b Fraunhofer ISE: Study of electricity generation costs for renewable energies March 2018 . Retrieved April 9, 2018.
- ↑ Omar Ellabban, Haitham Abu-Rub, Frede Blaabjerg: Renewable energy resources: Current status, future prospects and Their enabling technology . In: Renewable and Sustainable Energy Reviews 39, (2014), 748–764, p. 749, doi: 10.1016 / j.rser.2014.07.113 .
- ^ Mark A. Delucchi, Mark Z. Jacobson : Providing all global energy with wind, water, and solar power, Part II: Reliability, system and transmission costs, and policies . In: Energy Policy 39, Vol. 3, (2011), 1170–1190, p. 1176, doi: 10.1016 / j.enpol.2010.11.045 .
- ^ Brian Vad Mathiesen et al .: Smart Energy Systems for coherent 100% renewable energy and transport solutions . In: Applied Energy 145, (2015), 139–154, p. 151, doi: 10.1016 / j.apenergy.2015.01.075 .
- ^ Aviel Verbruggen, Volkmar Lauber : Basic concepts for designing renewable electricity support aiming at a full-scale transition by 2050 . In: Energy Policy 37, (2009), 5732-5743, p. 5742, doi: 10.1016 / j.enpol.2009.08.044 .
- ↑ Jörg Böttcher (Ed.): Handbook Wind Energy. Onshore projects: implementation, financing, law and technology. Munich 2012, p. 29.
- ↑ Klaus Heuck, Klaus-Dieter Dettmann, Detlef Schulz: Electrical energy supply: generation, transmission and distribution of electrical energy for study and practice. 9th edition. Vieweg + Teubner, Wiesbaden 2013, p. 43.
- ^ Nicola Armaroli , Vincenzo Balzani : Towards an electricity-powered world . In: Energy and Environmental Science 4, (2011), 3193-3222, p. 3217, doi: 10.1039 / c1ee01249e .
- ^ A b David Richard Walwyn, Alan Coli Brent: Renewable energy gathers steam in South Africa . In: Renewable and Sustainable Energy Reviews 41, (2015), 390–401, p. 391, doi: 10.1016 / j.rser.2014.08.049 .
- ↑ Mario Pagliaro, Francesco Meneguzzo, Federica Zabini, Rosaria Ciriminna: Assessment of the minimum value of photovoltaic electricity in Italy . In: Energy Science and Engineering 2 (2014), 94-105, p. 95, doi: 10.1002 / ese3.36 .
- ↑ Volker Quaschning : Regenerative Energy Systems. Technology - calculation - simulation . 9th updated edition. Munich 2015, p. 408.
- ^ Abdul Ghafoor, Anjum Munir: Design and economics analysis of an off-grid PV system for household electrification . In: Renewable and Sustainable Energy Reviews 42, (2015), 496–502, p. 497 doi: 10.1016 / j.rser.2014.10.012 .
- ^ Epstein et al .: Full cost accounting for the life cycle of coal . In: Annals of the New York Academy of Sciences 1219, (2011), 73-98, p. 77, doi: 10.1111 / j.1749-6632.2010.05890.x .
- ↑ Martin Kaltschmitt / Wolfgang Streicher (eds.): Regenerative energies in Austria. Basics, system technology, environmental aspects, cost analyzes, potentials, use. Wiesbaden 2009, p. 41.
- ↑ Lignite in the sights of environmentalists . Goethe Institute . Retrieved April 15, 2014.
- ^ Effects of renewable energies on the soil. (PDF; 11.8 MB) Bavarian State Office for the Environment , archived from the original on November 2, 2014 ; accessed on January 26, 2020 . .
- ^ Ziyad Salameh: Renewable Energy System Design , Academic Press 2014, p. 164.
- ↑ Martin Kaltschmitt , Wolfgang Streicher, Andreas Wiese (ed.): Renewable energies. System technology, economic efficiency, environmental aspects , 5th edition, Berlin Heidelberg 2013, p. 539f.
- ↑ Erich Hau: Wind turbines - basics, technology, use, economy. 5th edition. Springer, Berlin Heidelberg 2014, p. 671f.
- ↑ Martin Kaltschmitt , Wolfgang Streicher, Andreas Wiese (ed.): Renewable energies. System technology, economic efficiency, environmental aspects , 5th edition, Berlin Heidelberg 2013, p. 440f.
- ↑ Viktor Wesselak , Thomas Schabbach , Thomas Link, Joachim Fischer: Handbuch Regenerative Energietechnik , Berlin / Heidelberg 2017, p. 251.
- ↑ Kim Trapani, Miguel Redón Santafé: A review of floating photovoltaic installations: 2007–2013 . In: Progress in Photovoltaics 23, (2015), 524-532, doi: 10.1002 / pip.2466 .
- ^ Mark Z. Jacobson , Mark A. Delucchi: Providing all global energy with wind, water, and solar power, Part I: Technologies, energy resources, quantities and areas of infrastructure, and materials . In: Energy Policy 39, Vol. 3, (2011), 1154–1169, p. 1161, doi: 10.1016 / j.enpol.2010.11.040 .
- ^ Nicola Armaroli , Vincenzo Balzani : Towards an electricity-powered world . In: Energy and Environmental Science 4, (2011), 3193-3222, p. 3216, doi: 10.1039 / c1ee01249e .
- ↑ a b Oliver Türk: Material use of renewable raw materials: Basics - Materials - Applications , Wiesbaden 2014, p. 15.
- ↑ Volker Quaschning : Regenerative Energy Systems. Technology - calculation - simulation . 9th updated edition. Munich 2015, p. 367.
- ↑ Maria Ukhanova, Nicolas Schoof, Lucas Neher, Rainer Luick: Balancing energy transition in Germany: how will it influence permanent grassland? A delphi study . Grassland Science in Europe, No. 23 . Wageningen Academic Publishers, Wagening 2018, ISBN 978-1-84170-643-6 , p. 679-681 .
- ↑ Martin Kaltschmitt , Wolfgang Streicher (Ed.): Regenerative Energies in Österreich. Basics, system technology, environmental aspects, cost analyzes, potentials, use , Wiesbaden 2009, p. 487.
- ↑ Viktor Wesselak , Thomas Schabbach , Thomas Link, Joachim Fischer: Handbuch Regenerative Energietechnik , Berlin / Heidelberg 2017, p. 170.
- ↑ Viktor Wesselak , Thomas Schabbach , Thomas Link, Joachim Fischer: Handbuch Regenerative Energietechnik , Berlin / Heidelberg 2017, pp. 169–172.
- ^ Brian Vad Mathiesen et al .: Smart Energy Systems for coherent 100% renewable energy and transport solutions . In: Applied Energy 145, (2015), 139–154, doi: 10.1016 / j.apenergy.2015.01.075 .
- ^ Brian Vad Mathiesen , Henrik Lund , David Connolly: Limiting biomass consumption for heating in 100% renewable energy systems . In: Energy 48, (2012), 160-168, doi: 10.1016 / j.energy.2012.07.063 .
- ^ Mark Z. Jacobson et al .: 100% clean and renewable wind, water, and sunlight (WWS) all-sector energy roadmaps for the 50 United States . In: Energy and Environmental Science 8, (2015), 2093-2117, doi: 10.1039 / c5ee01283j .
- ↑ Volker Quaschning : Regenerative Energy Systems. Technology - calculation - simulation . 9th updated edition. Munich 2015, p. 376f.