Nuclear energy

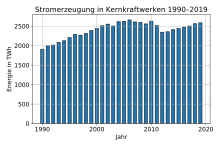
Nuclear energy , atomic energy , atomic power , nuclear power or nuclear energy is primarily the technology for the large-scale generation of secondary energy by means of nuclear fission . This technology has been used on a large scale for electricity production since the 1950s , while nuclear fusion energy , which is also covered by these terms, will only be able to play a role in electricity production in many years.
As of January 2020, 447 reactor units with a total output of 397 GW were in operation in 31 countries. Another 53 reactor blocks with a total output of 54.7 GW are under construction. To this end, more than 100 nuclear power plants are being planned for the coming decade. Since 181 nuclear power plant units have already been shut down in parallel to the new buildings, the number of reactors has remained largely constant since the 1990s. The share of nuclear power fell from 17.5% to 10.15% between 1996 and 2018 due to the increase in electricity generation worldwide . In 2018, the electrical energy generated in nuclear power plants was a good four percent lower than in 2006, when it reached an all-time high. In the EU , nuclear power plants cover around 25% of the electricity consumed (787 TWh generation with 117 GW installed capacity).
In addition to stationary nuclear reactors, there are around 180 reactors on around 140 watercraft, including nuclear submarines , aircraft carriers , some nuclear icebreakers , 4 cargo ships and a sea-based nuclear power plant . Satellites have also been operated with nuclear reactors and reactor concepts for aircraft have been researched.
There are many different views on the advantages and disadvantages of nuclear fission energy, so that its use in general and its safety in particular are controversial both in science and in the public.
history
Concept history
In 1899 , the physicist Hans Geitel was one of the first to coined the term atomic energy for the phenomena associated with radioactive decay processes. Later came synonyms atomic energy , nuclear power , nuclear power and nuclear energy added.
The use of these terms has experienced a politically and ideologically motivated shift. In the 1950s, Franz Josef Strauss was Federal Minister for Nuclear Affairs . A conference with high-ranking scientists held in Geneva in 1955 was entitled International Conference on the Peaceful Uses of Atomic Energy and became known in the German media as the Atomic Conference . As a result of this conference, the International Atomic Energy Agency (IAEA) was founded in 1957 . The lobby association of German companies interested in technology was founded in 1959 as the German Atomic Forum . In the following decades, the advocates of the technology distanced themselves from the prefix atom and only used kernel in Germany . At the same time, there was a shift from atomic to nuclear in the English-speaking world . The reason is the unwanted association with the increasingly negative concept of the atomic bomb ; the technical-physical justification emphasizes that the relevant processes take place in the nucleus and not in the entire atom, the chemical properties of which are determined by the atomic shell. Critics, on the other hand, kept the prefix atom both in the self-denomination of nuclear power opponents and in slogans such as “ Atomkraft? No thanks "at. You continued to speak of atomic energy and nuclear power plants with the abbreviation AKW.
The synonym atomic nuclear energy was used in the early days of technical use (change of name from the atomic ministry to the federal ministry for nuclear energy in 1961) and to this day as a term under nuclear law, for example at the state committee for atomic nuclear energy .
All of these terms related to nuclear fission energy. The term “atomic nuclear energy” also includes “nuclear fusion energy”. On October 31, 1952, the first fusion-based hydrogen bomb was detonated.
Technology history
The first experiments on radioactivity were carried out around 1890 . The aim of Antoine Henri Becquerels , Marie and Pierre Curies and others was the study of nuclear reactions.
In 1938 Otto Hahn and Fritz Straßmann discovered the induced nuclear fission of uranium, which was theoretically explained by Lise Meitner and Otto Frisch in 1939 . Together with the evidence provided in particular by Frédéric and Irène Joliot-Curie that a chain reaction is possible because several more neutrons are released for each nuclear fission triggered by a neutron, the practical application possibilities of nuclear fission became clear.
First, these findings were used for military research during the Second World War . As part of the Manhattan Project succeeded Enrico Fermi on December 2, 1942, the first controlled nuclear chain reaction in a nuclear reactor in Chicago ( Chicago Pile One). While the goal of the Manhattan project led by Robert Oppenheimer was achieved with the first successfully detonated atomic bomb on July 16, 1945 ( Trinity test ), the German research group under Werner Heisenberg and Carl Friedrich von Weizsäcker did not succeed in finding a functioning one until the end of the war To develop nuclear reactor ( uranium project ).
After the Second World War, military research continued. At the same time, the civil use of nuclear energy was developed. At the end of 1951, the test reactor EBR-I in the US state of Idaho generated electricity from nuclear energy for the first time and lit four light bulbs on December 20. The first power plant for the large-scale generation of electrical energy was commissioned in 1954 with the Obninsk nuclear power plant near Moscow. In 1955, the Calder Hall nuclear power plant in north-west England followed on the site of the Sellafield nuclear complex .
In Germany, the first research reactor was put into operation in 1957 with the Munich research reactor in Garching . In 1961, in the district of Karlstein am Main , the Kahl nuclear power plant, with an output of 15 MW, fed electricity from nuclear energy into the West German supply network for the first time. In 1966, the Rheinsberg nuclear power plant began operating in the GDR . The expansion of nuclear energy in West Germany was not driven by the market or as a reaction to an energy shortage. Instead, state authorities played the key role. For example, the energy supply companies "have long been the slowing factor in the implementation of nuclear energy". It is even believed that in the early years the decisive impetus for the German nuclear energy program was to create the option of nuclear armament. While German nuclear policy, as a continuation of the nuclear project during the Nazi dictatorship, initially relied on the heavy water reactor , the more affordable American concept of the light water reactor , a “victory of economists over technicians” , was adopted in the 1960s .
This imitation of the Americans gave rise to specific problems for Germany: For example, in view of the USA's status as a nuclear power , the American civilian reactors were chosen in such a way that they benefited from the military uranium and plutonium plants, which means that a fluid border with military technology is a basic requirement for those there Reactor development was. The suitability of American reactor technology for Germany was therefore questionable, at least in the event that Germany had perceived itself as a non-nuclear power for all time. In addition, the safety philosophy on both sides of the Atlantic was different: in the USA it was aware that light water reactors offered less inherent safety than other reactor types discussed at the time. Therefore, there was broad consensus that one must expect the worst and that nuclear power plants should therefore be built predominantly in sparsely populated and easily evacuated regions. In the much more densely populated Federal Republic, however, this was not possible, since otherwise it would hardly have been possible to identify reactor sites. Instead, in order to be able to avoid a corresponding safety distance, consideration was given to building nuclear power plants underground, but this was vehemently rejected by the nuclear industry. Other plans, however, specifically provided for nuclear power plants, which should be located near the large cities of Ludwigshafen am Rhein and Frankfurt am Main , in order to supply the chemical industry located there with process heat ; largely motivated by the considerations to prove the special reliability of German nuclear power plants.
In the 1960s, numerous other nuclear power plants with significantly higher output were built. The Gundremmingen nuclear power plant , which went into operation in 1966, had an output of 250 MW. In 1968 the ore freighter " Otto Hahn " was put into operation as a nuclear research cargo ship; after the end of nuclear operation in 1979, it was converted back to diesel drive.
In the 1970s, especially after the first oil crisis in 1973, the construction of nuclear power plants was pushed. These nuclear reactors , such as Unit B of the Biblis nuclear power plant , have an output of around 1.3 GW (= 1300 MW). In the course of the protests of the anti-nuclear movement against the construction of the Wyhl nuclear power plant in Germany in 1975, there was a greater opposition to the civil use of nuclear energy. In 1978, a referendum in Austria resolved not to put the already completed Zwentendorf nuclear power plant into operation. Criticism of nuclear power was intensified and intensified in particular by the serious reactor accident at the Three Mile Island nuclear power plant near Harrisburg ( USA ) on March 28, 1979, when a partial core meltdown occurred for the first time .
In 1983 the thorium high-temperature reactor ( THTR-300 nuclear power plant ) was put into operation in Schmehausen . It goes back to the developments by Rudolf Schulten . This prototype of a pebble bed reactor was shut down six years later after several technical malfunctions, long periods of standstill and only 14 months of full load operation. The shutdown had become necessary because the plant was on the verge of bankruptcy in 1989 and no agreement could be reached on assuming the high operating losses that would continue to be expected. The THTR was transferred to safe enclosure .
On April 26, 1986, the Chernobyl catastrophe occurred , in which, after a core meltdown , large amounts of radioactivity also fell in Western Europe . As a result, criticism of the use of nuclear energy increased significantly, especially in Europe. In 2000, under pressure from the federal government, it was decided in Germany to phase out the commercial use of nuclear energy by around 2020. In this context, two nuclear power plants were taken off the grid by 2005. In 2010 the black-yellow coalition Cabinet Merkel II decided to extend the service life of German nuclear power plants by 8 or 14 years. This decision was politically and socially controversial (especially since the Fukushima nuclear disaster in Japan in March 2011). The serious accident in Fukushima showed that the light water reactor with Mark-I containment from General Electric , which is widely used around the world, does not fully meet the safety requirements, with the reasons for the accident also being the incorrect decisions of the operator (economy over safety) and negligence on the part of the authorities . In response to this, the federal government first announced a three-month nuclear moratorium in March 2011. Finally, the nuclear consensus decided to phase out by 2022, and the eight oldest nuclear power plants were shut down immediately.
International perspective

(Output of all newly installed nuclear power plants [solid frame] or all destroyed or permanently shut down nuclear power plants [dotted frame] - broken down by year and country. The legend gives the ISO-3166-1 codes of the countries. Source :)
According to IAEA / PRIS, the number of nuclear power plants and the installed capacity have increased since 1995. The number of reactor blocks increased from 434 to 445, the available capacity increased from 341 GW to 387.4 GW. In 2011, according to the IAEA, 65 reactors were under construction worldwide and 114 in the planning stage. However, after the Fukushima nuclear disaster , numerous new construction plans were revised. For example, China temporarily stopped all new construction plans.
In the USA, President Barack Obama announced the construction of a "new generation of safe, clean nuclear power plants" and offered government loan guarantees of 38.6 billion euros. As of February 2020, there are two nuclear reactors under construction in the USA .
The former French government under François Fillon affirmed in 2011 that nuclear energy would remain the cornerstone of the 40-year energy independence policy. President Hollande, newly elected in May 2012, agreed in the election campaign with the (French) Greens to shut down 24 of the 58 French reactors.
As of February 2020, seven nuclear power plants are being built in India . It is envisaged that 25% of electricity needs will be covered by nuclear energy by 2050.
As of February 2020, there are 10 nuclear power plants under construction in China , and around 148 additional reactor blocks are in the planning stage. In March 2011, the government temporarily suspended the approval of new nuclear power plants. By 2020, the installed capacity was planned to increase eightfold to a total of 80 GW, but by February 2020 it was only 45.53 GW. In July 2011 it was reported that China would again rely on the rapid expansion of nuclear energy. The Fukushima nuclear disaster did not change that much.
As of February 2020, Russia operates 38 reactors and is building 4, 31 are in the planning stage.
South Korea previously promoted nuclear energy, currently 5 reactors are still under construction. In June 2017, the South Korean president announced Moon Jae-in on, completely out of nuclear power by 2057 to get out . The existing nuclear power plants are to go offline after 40 years, the oldest reactor block, Kori 1, was shut down on June 18, 2017.
After a referendum in June 2011 in which 95% of citizens voted against re-entry, Italy shelved the re-entry planned by the Berlusconi government .
The Czech Republic has rejected new construction plans for nuclear power plants. The tender for the two new nuclear power plants at the Temelín site was withdrawn by the semi-state energy company ČEZ.
Germany decided under the Schröder government to phase out nuclear power by around 2020/21. The Merkel government initially extended the terms, but initiated an accelerated nuclear phase-out by 2021 after the reactor accident in Fukushima.
In Japan , until May 5, 2011, all reactors were successively taken off the grid for maintenance reasons. The local parliaments, which have long rejected all applications, are responsible for the restart permit. In mid-September 2012, a year and a half after the Fukushima nuclear disaster, the Japanese government decided to phase out nuclear energy by 2040 at the latest. However, the Shinzo Abe government has reversed this phase-out. The Sendai nuclear power plant was restarted on August 10, 2015 as the first nuclear power plant after the Fukushima nuclear disaster. Applications for readmission have been pending for a further 25 reactors. After the nuclear power plants were shut down, electricity prices rose by 20 to 30%; Japan had to import additional fossil fuels for an estimated € 26 billion a year. By 2019, 8 reactors were back on the grid, 21 were finally shut down and 25 are officially in operation, although most of them will never be connected again. For example, Fukushima II has been out of operation since March 2011, but was not officially shut down for good until September 30, 2019.
Technologies
The nuclear power plants include the numerous existing power plants based on nuclear fission and those based on nuclear fusion, which have been propagated for decades as the future .
Nuclear fission
In the induced fission a decomposes nucleus of a uranium - or plutonium - isotope , having a neutron has absorbed into (usually) two lighter nuclei (the fission fragments ). The energy released comes from the difference in binding energy of the fission fragments compared to the original nucleus and is released in the form of kinetic energy of the fission fragments and as gamma radiation . Including the energy that is still released during the subsequent radioactive decay of the fission fragments, this results in around 200 MeV per fission , i.e. just under 1 MeV per nucleon. In addition to the fission products, 2-3 prompt neutrons are also released during the fission . These can cause further nuclear fission and thus lead to a chain reaction . The delayed neutrons that are still released from the fission fragments after the fission make it possible to control the chain reaction in a nuclear reactor technically (see criticality ).
The energy yield of around 200 MeV per fission corresponds to thermal energy of around 0.96 MWd (megawatt days) per gram of uranium-235 or plutonium-239. The same thermal energy can be obtained by burning 2.8 tons of hard coal, 10 tons of lignite or 1.9 tons of light heating oil.
Nuclear power plant
Nuclear power plants convert the energy from nuclear fission into thermal energy and this into electrical energy. Nuclear power plants carry out controlled chain reactions of nuclear fission in nuclear reactors . The heat released in this process is used to produce water vapor, which is directed to turbines that drive generators and produce electricity in the process. Furthermore, in the military sector, some aircraft carriers , nuclear submarines and a few nuclear cruisers are equipped with nuclear energy propulsion; In the civil sector, this drive has only established itself for nuclear icebreakers ( see also: List of civilian ships with nuclear drive ).
security
The safety of nuclear fission power plants plays an increasingly important role, especially as a result of the reactor accidents in Chernobyl and Fukushima , in which the chain reaction and the production of residual heat got out of control. The ever stricter safety regulations led to many additional safety measures, but also to increased operating costs.
Fuel chain
The generic term fuel cycle is common for the entirety of the work steps that are used to supply nuclear reactors with fuel elements , including the measures required to dispose of the radioactive waste . This term was originally known in the discussion about the construction of the Wackersdorf reprocessing plant . The term “cycle” does not mean a complete recycling of the material; In reprocessing plants, the used fuel is to be broken down into its constituent parts after it has been removed from the reactor, so that raw material for new fuel assemblies is obtained.
Range of fuels
Price category | secured | supposed | total | Range |
---|---|---|---|---|
$ / kgU | kt | kt | kt | Years |
<40 | 507 | 176 | 683 | 11 |
40-80 | 1,212 | 745 | 1.957 | 31 |
80-130 | 3,699 | 2,204 | 5,902 | 95 |
130-260 | 4,587 | 3,048 | 7,635 | 123 |
total: | 16,178 | 261 |
Similar to fossil fuels, the stocks of nuclear fuels on earth are limited. The table gives an overview of the known minable uranium reserves and uses the current consumption of almost 62,000 tU / year. At the moment (7/2016) the uranium price is around $ 60 / kg. Since uranium only makes up a fraction of the value chain of nuclear energy (according to the information letter from the Scientific Services of the German Bundestag around 5%), even a multiple of the uranium price would only have a small effect on the total costs and thus the price of electricity.
The use of thorium (Th 232 ) as a nuclear fuel is proposed by various sources. However, thorium is not fissile, it must first be converted into fissile U 233 in an incubation process, similar to the use of the U 238 . Thorium is around three times more common in the earth's crust with 9.6 ppm than uranium with 2.7 ppm, of which only U 235 with a weight fraction of 0.7% has been used so far . With the help of this breeding technology, which has not yet been used on a large scale, the range given in the table could be extended by a factor of 100 (U 238 ) or 300 (Thorium).
Since no more nuclear fuel is mined in Germany, German nuclear power plants are dependent on imports.
Extraction and enrichment
After mining, the ore is ground and the uranium is extracted chemically - usually as triuranium octoxide (U 3 O 8 ). The U 3 O 8 is then converted into gaseous uranium hexafluoride (UF 6 ). While uranium with the natural isotope distribution of 99.3% 238 U to 0.7% 235 U can be used in heavy water reactors and in breeder reactors , the widespread light water reactors require enriched uranium with a proportion of up to about 6% 235 U. The enrichment of 235 U is usually carried out by means of gas diffusion or ultracentrifuges of uranium hexafluoride. The uranium enriched in U-235 is then processed into fuel rods as uranium dioxide, possibly together with plutonium dioxide as mixed oxide . Several fuel rods are then combined into fuel assemblies .
Around half of uranium production currently takes place in sparsely populated areas of Kazakhstan, Canada and Australia. Uranium and thorium are mostly extracted from mining for other more common metals, such as the Olympic Dam mine in Australia. The uranium content of currently used deposits fluctuates considerably between 0.03 and 18 percent. The uranium mining in the former GDR was historically important and important for the production of weapons , especially in the case of Wismut .
When operating nuclear reactors, irradiating uranium with neutrons produces fissile plutonium. It is obtained from the spent fuel during reprocessing and can be used for new fuel assemblies. Another currently (2016) significant source is uranium and plutonium from former nuclear weapons that were decommissioned as a result of disarmament .
The World Nuclear Association believes that it is possible to increase uranium reserves by extracting them from coal-fired power station ash.
Interim storage
Many different radioactive isotopes are produced during nuclear fission . Therefore, spent fuel assemblies radiate strongly; their material must not get into the environment. Immediately after use, the radiation is so strong that further processing is not possible. For this reason, the fuel elements are stored for a few months or years in the interim storage facility of the nuclear power plant in a cooling pool . After this time, short-lived isotopes have largely decayed . However, long-lived isotopes remain, which means that the fuel elements are still highly radioactive and also continuously produce heat. Some of these isotopes are fissile and, after chemical separation, can in principle be used as nuclear fuel. The rest must be stored until radioactive decay has rendered it harmless.
Special transport containers , such as Castor containers, are used for the removal and storage of the fuel assemblies outside the decaying basin . Burned, non-reprocessed fuel assemblies and radioactive waste from reprocessing plants are stored in storage containers in interim storage facilities until the heat development has subsided to such an extent that final storage is possible. This takes a few decades.
Remanufacturing
In reprocessing plants - such as the La Hague reprocessing plant in France - the 95% uranium and 1% plutonium contained in spent fuel can be separated from the 4% fission products and higher actinides . After re-enrichment (e.g. in Seversk, Russia ) about 10% of the separated uranium can be reused. 90% is still radioactive waste . However, the proportion of highly active waste drops considerably (e.g. 5%). According to studies by Greenpeace, around 0.5 billion liters of radioactively contaminated wastewater is discharged into the English Channel in La Hague and radioactively contaminated exhaust air is released over Europe. The proportion of radioactive krypton ( 85 Kr) in the exhaust air (approx. 90,000 Bq per cubic meter of air) is particularly significant .
In Germany, a reprocessing plant was under construction in Wackersdorf , but was not completed for financial reasons and due to strong resistance from the population.
One way of converting long-lived radioactive waste is to transmutate this waste into isotopes that are either stable or whose radioactivity has declined to a harmless level within a few hundred years. These systems are currently in research and development and are funded at European level by the Eurotrans project. Technically, these systems consist of a proton accelerator system and a target in which fast neutrons are generated. Some of the problematic isotopes can be converted into less problematic isotopes by bombarding them with fast neutrons. However, the transmutation requires multiple, complex reprocessing. The first test facility is to be built in 2020 as part of the Myrrha project in Belgium.
Disposal
The radioactive waste from a nuclear power plant still radiates strongly even after decades. Highly radioactive waste ( High Active Waste ) only subsides sufficiently after a few thousand to a few hundred thousand years (depending on what is classified as harmless). In addition, some of the elements it contains are chemically very toxic. For this reason, radioactive waste must be stored in a repository in such a way that it is permanently kept away from the biosphere . Highly radioactive waste must have subsided to such an extent that the decay heat can no longer weaken the storage container (e.g. due to corrosion). This takes place in interim storage facilities that can be designed as dry or wet storage facilities. In Germany, dry storage is common, the steel storage containers - for example castors - are stored upright and have cooling fins.
In 2015, the Finnish government issued the building permit for the world's first repository for highly radioactive waste, the Onkalo repository in Olkiluoto. Storage is to begin in 2020.
Dismantling a nuclear power plant
At the end of the life of a nuclear power plant after around 40 years, the nuclear power plant is decommissioned and dismantled. According to the IEA, around 200 nuclear power plants are to be decommissioned due to old age by 2024. The IEA estimates the cost of dismantling these nuclear power plants at over USD 100 billion. The effort is so high because almost all components are radioactive, have to be separated and stored according to their half-life .
Nuclear fusion
The hydrogen isotopes deuterium and tritium can be used to generate energy from nuclear fusion on Earth . During their fusion, very fast neutrons are created, whose energy is to be used as heat to generate electricity, and helium ions, whose energy can maintain the plasma temperature required for fusion. The following paragraphs describe processes that are under development and successful on a small scale, but not yet implemented on a large scale.
Nuclear fusion power plant
Nuclear fusion power plants are supposed to convert the energy from nuclear fusion into thermal energy and convert this into electrical energy by using turbines with generators coupled to them to produce electrical power .
security
In terms of the safety of nuclear fusion power plants, only the outward (neutron and gamma) radiation plays a role. A chain reaction does not take place. Unintentional increases in performance are not possible: An increase in temperature (e.g. if the cooling fails) leads to the plasma inflating, which then hits the walls of the plasma chamber and is immediately extinguished. Other minor disturbances also cause the plasma to go out immediately. In the event of a sudden leak in the plasma chamber wall, nothing escapes, but rather, due to the low density of the plasma (high vacuum), around 500,000 times the mass of fuel (10 g) of cold outside air (5 t) flows in.
Radioactivity, waste
Nuclear fusion does not produce any radioactive waste, but rather non-radioactive helium. However, the material used for construction is activated . This radioactivity "inventory" of a fusion power plant will be comparable to that of a nuclear power plant of the same capacity, but the half-lives are significantly shorter with a suitable choice of material. The fuel tritium , of which a few kilograms would be in the power plant during operation, represents only a small part of the total activity in continuous operation; The inventory built up by neutron activation of the structural materials would be far larger .
However, the radioactivity is distributed over a much larger amount of material than in the nuclear fission power plant, because the reactor is much larger overall and the outer parts also contain radionuclides. In an unspecified example, it is stated that “a fusion reactor” will produce 16,000 tons of radioactive waste over a period of 30 years because the blanket modules have to be replaced from time to time. If it were demolished, more than twice the amount would be added. The radioactivity of 90 percent of this waste would have subsided enough after 50 years that it could be released into the environment. The remaining ten percent would have to be stored in underground repositories for 100 years.
Fuels
Equal volumes of the hydrogen isotopes deuterium and tritium are used. Tritium is bred from lithium in the reactor. The raw material costs for deuterium and tritium are insignificant. The stable gas helium is produced as a direct waste material from the fusion reaction.
Deuterium is naturally contained in water; the oceans contain so much deuterium that the Mediterranean could be filled with it several times. The deuterium stocks are therefore practically unlimited. The radioactive tritium is bred from lithium . This would take place in the fusion power plant itself, so that the tritium always remains within the plant. The worldwide conventionally minable lithium reserves are estimated at around 30 million tons, which leads to a range of over 100,000 years. The neutron released during the fusion, after it has given up its energy, meets lithium-6 in the blanket of the fusion reactor, whereby one tritium atom is formed in each case. A neutron multiplier such as beryllium or lead is used to control the breeding rate .
Dismantling
At the end of the life cycle of a nuclear fusion power plant, decommissioning and dismantling take place. The effort for this is as high as with nuclear fission power plants, because many components are radioactive, have to be separated and stored according to their half-life .
Economic prospects for the future
The German Renewable Energies Act aims for a share of renewable energies in electricity production of at least 80% by 2050. The Advisory Council for Environmental Issues and the German Federal Environment Agency even consider it possible to generate electricity entirely with renewable energies by 2050 if electricity were used and generated very efficiently. There is therefore the possibility that nuclear fusion will no longer be needed in Germany if it should be available in perhaps 50 years.
economics
The economic viability of nuclear fission energy is controversial in both professional and public discourse. While nuclear power plants that have been written off are considered cheap, the economic viability of newly built nuclear power plants is questionable, which is why the cost information in this regard is subject to great uncertainty. According to Constantine, for example, the production costs of a fully written-off nuclear power plant of the 1.3 GW class are 2.18 cents per kilowatt hour, while in a study by the Öko-Institut , which emerged from the anti-nuclear movement , operating costs amount to 1.7 cents per kilowatt hour is assumed.
However, depreciated nuclear power plants are also coming under economic pressure in markets in which electricity prices have fallen as a result of current economic developments such as the shale gas boom in the USA and the expansion of renewable energies in many countries around the world. In the USA, several nuclear power plants have therefore been decommissioned long before their approved end of life.
In the case of new buildings, in addition to any rising investment costs, the economic risks that must also be taken into account and external costs cause great uncertainty. These can only be estimated to a limited extent, but they have a considerable effect on profitability. In addition, the high investment costs result in very long refinancing periods of more than 30 years after commissioning (plus planning and construction time), in which the operators are subject to a high investment risk, especially in liberalized markets with the difficult-to-forecast revenues there. In 2009 Citibank published a study with the meaningful title: "New Nuclear - The Economics Say No". In some countries, planned power plant projects are therefore being postponed or canceled, while in other countries, such as the USA or Great Britain, subsidies are granted in order to make the construction of power plants economical.
Today, nothing binding can be said about the profitability and competitiveness of future nuclear fusion power plants, as neither the actual construction costs for approx or taxes) can influence it.
LCOE and competitiveness
The electricity production costs result from the use of nuclear energy, especially from the comparison with other power plants high cost of its construction and the financing conditions on the capital market . In the case of new reactors of the EPR type, the share of investment costs in electricity generation costs is estimated at around 65%, while fuel costs only make up around 12%. Nuclear power plants are therefore expensive to build, cheap to operate and expensive to dismantle.
In 2009, Konstantin estimated the specific investment costs for nuclear power plants to be more than twice as high as those for large lignite power plants. For the EPR with 1600 MW electrical output, he forecasted construction costs of 4.2 billion euros (corresponds to 2625 euros / kW), provisions for dismantling of 320 million euros, 48 months of construction and commissioning in 2004, electricity generation costs of 5, 02 cents per kilowatt hour.
Up until 2014, the investment costs of the EPR under construction had risen significantly compared to the original plans: Both the Olkiluoto 3 reactor in Finland and the French nuclear power plant Flamanville saw massive overruns of the originally planned construction costs and delays in the construction process of up to more than 9 years . The original target of building costs of 3 and 3.3 billion euros, as of 2012, was 8.5 billion each, resulting in investment costs of around 5300 euros / kW. For two further nuclear power plant blocks of this type in Great Britain, a total investment of almost 19 billion euros is calculated, which corresponds to an investment of almost 6000 euros per kW with a combined nominal output of 3200 MW. In order to still make the project economical, a feed-in tariff of 92.50 pounds / MWh (converted 11 cents / kWh) plus inflation compensation is charged for 35 years. This is below the feed-in tariff for large photovoltaic and offshore wind turbines in Great Britain, but above that for onshore wind turbines. This means that the feed-in tariff is twice as high as the current market price. At the same time, the state guarantees 65 percent of the construction costs.
Since different types of reactors from different manufacturers are being built around the world with inconsistent safety standards, the costs of the EPR need not necessarily be representative of all nuclear power plants currently under construction. At the Vogtle nuclear power plant under construction in the USA , where two Westinghouse AP 1000 reactors, each with an output of around 1100 MW, an investment sum of 14 billion dollars (10.5 billion euros) was initially assumed at the beginning of 2012. for two reactors, which corresponds to 4800 euros / kW. In February 2014, a few months after the start of construction, construction costs had risen to 15.5 billion dollars; the originally planned completion dates in 2016 and 2017 were each postponed by two years. The construction costs of the blocks 3 and 4 of the Slovak Mochovce nuclear power plant , in which the Russian type VVER is -440/213 used with a rated power of 405 MW, however, given as 3.8 billion Euros, an investment of about 4700 euro / kW corresponds. In countries with lower purchasing power , the values can be lower. So z. In China, for example, building eight AP1000s would cost a total of US $ 24 billion. H. around 2000 euros / kW at an exchange rate of 1.35 to 1.
As a result of these price increases in various power plant projects, the economic profitability of nuclear energy has been called into question for a number of years, especially in liberalized markets, and several power plant projects have been terminated. The construction of new nuclear power plants is therefore currently largely limited to countries in which state operators bear the risk of the projects. According to Neles and Pistner, new nuclear power plants are currently only being built where certain conditions are met. These are:
- the payment of government funds such as in the USA
- an electricity market that is not organized in a competitive manner, such as B. in France , Russia or China
- where there is interest in building a prototype whose financial risk lies not with the operator but with the manufacturer, such as B. in Finland
Also Uekötter points out that a further additional construction of nuclear power today, especially in authoritarian held out states where the laws of the market do not materialize and also the participation of the population is low.
The relative competitiveness of nuclear energy is difficult to determine because there are hardly any valid comparative data and historical data do not provide a clear picture. In addition, nuclear energy has received extensive government funding in all user countries around the world. These subsidies flowed into nuclear energy in various ways and were partly hidden in technical details, whereby, according to Uekötter, mixing with the military use of nuclear energy was particularly important. It is certain, however, that the use of nuclear energy would have "had no chance" without massive state aid.
While there was true atomic euphoria in certain circles in the 1950s and 1960s, this mood shifted in the 1970s when it became clear that the profitability of the nuclear power plants that had been implemented was significantly worse than had previously been believed. In Europe, therefore, after a boom in the 1960s and 1970s, the construction of nuclear power plants declined and then ebbed completely in the early 1980s. According to Uekötter, the reason for this practically Europe-wide construction freeze were largely economic considerations, while the Chernobyl nuclear disaster had hardly any influence on the states' nuclear programs; at this point in time, the further expansion plans had already been stopped.
According to Hans-Joachim Braun , for example, at the beginning of the 1980s, when the majority of the installed capacity from nuclear power plants worldwide (326 GW) was already connected to the grid, electricity production with nuclear power plants in Germany continued to be more expensive than electricity production with coal-fired power plants. According to the French authors Debeir / Deléage / Hémery, nuclear power plants in Western Europe and Canada offered a cost advantage over coal-fired power plants in the mid-1980s, while competitiveness was achieved in the USA, but coal-fired electricity production became cheaper than nuclear energy. Great influence was attributed to the development of fossil fuel prices on the one hand, while the authors emphasized on the other hand that the construction times of American nuclear power plants had already been extended from six to ten years in the 1970s and, in parallel, the construction costs of nuclear power plants continued to rise. Ultimately, "even after thirty years, nuclear power is still not really economically and financially viable".
With global warming becoming more and more obvious , there was a renewed debate about the use of nuclear energy in the 21st century against the background of its low CO 2 emissions, but this has so far been followed by very little construction activity. After the nuclear disaster in Fukushima, higher production costs are also to be expected due to increased safety measures.
Critics nowadays consider nuclear power to be uneconomical because the high capital costs cannot be offset by the low fuel costs. In addition, in the past, waste disposal costs were often not billed to the electricity companies that caused them, but paid for by the state. Costs for nuclear energy in Germany are borne by the state in the billions. B. for the decommissioning of research reactors or the rehabilitation of research repositories like the Asse . In a study by Citibank , the construction of new nuclear power plants without government subsidies was found to be too risky and not economically feasible: " New Nuclear - The Economics Say No ".
According to an analysis by the Hertie School of Governance on major projects in Germany, the construction of offshore wind farms incurred significantly less additional costs than the historically built nuclear power plants. Case studies on the construction of eight offshore wind farms and six nuclear power plants showed that wind farms showed significant learning effects despite considerable planning risks; As a result, the additional costs of the offshore wind farms were 20% higher than originally estimated, but three times higher for the nuclear power plants. Learning effects could not be found in the case of the nuclear power plants.
Another study in 2010 came to the conclusion that the total costs for solar power were below the electricity production costs from new nuclear power plants. Any costs incurred for storing the solar power and the required control reserve were not taken into account.
Economic damage risk and inadequate liability insurance
In the event of a nuclear accident in Germany, the follow-up costs are insured up to an amount of € 2.5 billion as part of liability insurance. The sum is specified in the Atomic Energy Act. In addition, the operators are liable with all their assets for further costs. Based on the experience of the Chernobyl and Fukushima disasters, however, it is certain that neither the financial security provided by the operators nor the support provided by the public sector would be sufficient to compensate for all the damage caused by such an event. The European Nuclear Law Handbook . Nuclear Energy Law. holds on:
“The advantage of the limitation of liability for the operator of a nuclear facility is to be able to plan economically. This is also to ensure that nuclear activities take place. It is clear to all experts that in the event of a nuclear accident the liability limits are insufficient and that the individual states must use public funds to compensate for the damage. "
A French government study carried out by the French Institute for Radiation Protection and Nuclear Safety (IRSN) estimates the economic damage from a catastrophic accident in a French nuclear power plant at € 430 billion, which corresponds to a quarter of the country's annual economic output. In the case of private insurance for citizens (household insurance, building insurance), risks from nuclear energy are generally excluded.
It is also open who bears the costs for the dismantling of decommissioned nuclear power plants in the event of the operator's insolvency . In the draft of the working group on the environment for the coalition agreement between the Union and the SPD it says: "To secure the financing of the nuclear waste disposal, a fund under public law could be considered". The coalition partners are considering pooling the provisions of the nuclear power plant operators for decommissioning and final storage in such a fund. It is feared that the state would have to pay for costs in the event of bankruptcies. So far, e.on, RWE, EnBW and Vattenfall have been able to set up 32.6 billion euros in provisions and invest the money immediately in power plants and networks. State-controlled funds have been required for a long time.
Electricity price in Germany
According to a study by the ISE , which was commissioned by the Bundestag parliamentary group from Bündnis 90 / Die Grünen , nuclear power plants were operated with up to 96% of the installed capacity in times of negative electricity prices on the exchange . The minimum utilization was 49%. The study therefore comes to the conclusion that in the case of nuclear power plants, a fine-grained, demand-adjusted curtailment cannot be determined on the basis of the investigated data.
According to the Federal Association of Consumer Organizations (vzbv), extending the service life of German nuclear power plants would not be a means of noticeably lowering the price of electricity for private consumers. For an average household, the monthly electricity bill would decrease by an average of “just 50 cents per month” if more electricity from nuclear energy were purchased.
Incomplete competition
Despite all efforts to liberalize and regulate, there is incomplete competition on the European electricity markets, which leads to excessive prices. This is especially true for the conditions in Germany. In the power plant sector, the four large companies RWE, E.ON, EnBW and Vattenfall dominate, accounting for around 85% of the total capacity.
Capital-intensive power plant types such as nuclear power plants that also produce large amounts of energy secure a strong position for their operators in the market. Several current studies are available for the German electricity market that empirically prove the influence of incomplete competition. The influence of the dominant position of the large energy companies on the market price of electricity is given as around 0.69 ct / kWh.
The additional income from nuclear energy due to incomplete competition is given in 2003 at 1.8 billion euros.
State regulation
Legal bases
The International Atomic Energy Agency (IAEA) is intended to promote international cooperation in the field of the peaceful uses of nuclear energy and the use of radioactive materials, while at the same time preventing the misuse of this technology (in particular the proliferation of nuclear weapons ) through surveillance measures. Various international treaties such as the Nuclear Non-Proliferation Treaty and the Nuclear Liability Convention provide corresponding guidelines.
In Germany , the legal basis for the civil use of nuclear energy is the German Atomic Energy Act (law on the peaceful use of nuclear energy and the protection against its dangers).
In Switzerland , the Swiss Atomic Energy Act (Federal Act on the Peaceful Use of Atomic Energy) was the legal basis until 2005 , and since then it has been the Nuclear Energy Act .
In Austria, on the other hand, the Federal Constitutional Law for a nuclear-free Austria has given the ban on the commercial use of nuclear reactors constitutional status after a national referendum since 1999.
Other ordinances , such as the Nuclear Insurance Cover Ordinance (AtDeckV) , implement international guidelines in Germany. The financial security for a nuclear power plant is 2.5 billion euros, which is partly covered by liability insurance and partly as a solidarity agreement between the nuclear power plant operators.
The maximum liability limit for damage that is directly attributable to acts of an armed conflict, hostility, civil war, uprising or a serious natural disaster of an exceptional nature is 2.5 billion euros. In theory, the operators have unlimited liability for damage from other causes; in fact, the liability is limited by the operator's equity. Other insurance, such as B. Comprehensive car insurance, as a rule, expressly excludes the coverage of damage that could be caused by nuclear energy accidents.
Subsidies and other grants
Nuclear energy was extensively subsidized by the state both in Germany and around the world .
European Union
Since the European Atomic Energy Community was founded in 1957, nuclear energy has been promoted politically and economically. According to the EU Commission, funds totaling 3 billion euros were available for the implementation of the seventh framework program in the period 2007–2011. In December 2011 it was decided to provide an additional 1.3 billion euros for fusion research in 2012 and 2013 as a result of the increase in the cost of the ITER fusion reactor . The money comes from budget funds for the years 2011–2013.
According to information from the information service dpa Insight EU, the subsidy for nuclear energy in the EU states is estimated at 35 billion euros in 2011, according to an internal report by the EU Commission, and this figure is picked up by various media. Accordingly, the subsidies for nuclear energy were higher than the subsidies for renewable energies (30 billion) and for fossil energies (26 billion); 15 billion was spent on efficiency measures. Due to the lack of transparency, the exact funding costs for nuclear energy in Europe are currently unclear. According to Radkau and Hahn , the largest subsidy for nuclear energy is the waiver of liability insurance with sufficient coverage, which is equivalent to the commitment of the state to largely assume the follow-up costs of serious reactor accidents.
Since currently (2012) hardly any companies are prepared to bear the very high investment costs in the construction of nuclear power plants, according to Süddeutscher Zeitung Great Britain, France, Poland and the Czech Republic are demanding subsidies for electricity generation using nuclear energy. According to this, among other things, feed-in tariffs should be introduced analogous to the promotion of renewable energies for nuclear power and nuclear power plants, as “low-emission technologies”, are to be equated with renewable energies. The construction of the power plants themselves is also to be subsidized. The EU Energy Commissioner, Günther Oettinger , has already announced his readiness to “discuss various options”. Previously, several planned projects for nuclear power plants had been canceled for financial reasons, the construction of the two nuclear power plants Flamanville 3 in France and Olkiluoto 3 had become much more expensive.
Germany
In Germany, nuclear energy was the first technology to be advanced with extensive government subsidies without any specific need. In the 1950s, at the time of atomic euphoria, their use was simply taken for granted. The initiative initially came from the chemical industry, while energy companies (and also the Federal Ministry of Economics) slowed down development and were therefore repeatedly criticized by those in favor of nuclear energy. When RWE planned its first nuclear reactor at the end of the 1950s, it was done “so as not to lose touch with technology”. However, the associated order was not placed until 1969 with the Biblis nuclear power plant.
On October 21, 1955, Franz Josef Strauss became Minister in the newly founded Federal Ministry for Atomic Affairs . The aim of this ministry was the introduction of nuclear energy, its promotion and the creation of the legal basis for it. In 1957, a year before the Atomic Energy Act was passed, the government pushed ahead with reactor planning. The reactors were to be developed and built by corporations, while the state granted loss guarantees and extensive investment aid. In the end, however, only two reactors were built, while the billions in funding that had been spent so far flowed into the establishment of development departments.
On November 13, 1960, the Kahl nuclear power plant went into operation as a test reactor with 15 MW, with American reactor technology from General Electric . In the early years it was believed: "No nuclear power plant in Germany could ever be competitive with a coal-fired power plant". For this reason, both the construction of the first nuclear reactors and the electricity fed in were subsidized by the state. Overall, several research programs were launched in West Germany, each with several billion marks. In Germany, the subsidy is given as around 2000 euros / kW installed capacity.
In a study from 2007, the German Institute for Economic Research (DIW) came to the conclusion that the German federal and state spending alone on nuclear energy research and technology from 1956 to 2006 amounted to at least 50 billion euros . This does not include, among other things, public expenditure for the refurbishment of uranium ore mining in Germany (€ 6.6 billion) and shares in the decommissioning / dismantling of nuclear facilities (€ 2.5 billion).
If you add these costs and relate them to the amount of electricity generated by nuclear energy of around 4100 TWh by the end of 2006, the result is an average support of 1.5 cents per kilowatt hour (ct / kWh). If one considers only the total of all quantified effects effective in 2006 (if information is available, including unification-related burdens and international projects) for the promotion of nuclear energy, the money supply amounts to 3.7 billion euros (currency value from 2006). This corresponds to support (167.4 TWh electricity from nuclear energy in 2006 in Germany) of 2.2 ct / kWh (currency value from 2006). The values are to be understood as the lower limit, since many of the costs of nuclear energy can hardly or not at all specifically be quantified and the figures “by no means include all public expenditures in favor of nuclear energy”. For example, damage caused by nuclear energy is not covered by any private household insurance, and the costs for the Gorleben salt dome and for the closure of the Asse II mine cannot be quantified.
In 2010, the Ecological-Social Market Economy Forum carried out an extensive study on behalf of Greenpeace : “State subsidies for nuclear energy” (2010). This determined a total of the subsidies of 203.7 billion euros for the period from 1950 to 2010, which would correspond to 4.3 ct / kWh. This includes tax breaks, the shutdown of reactors, research including nuclear fusion research, membership in international organizations such as Euratom and the rehabilitation of uranium mining facilities in the former GDR. In addition, according to Greenpeace, it would be up to 2.70 euros per kWh more expensive if the same liability rules apply to nuclear power plants as in all other economic sectors.
In this extensive exemption from liability insurance, the two economists Peter Hennicke and Paul JJ Welfens see a hidden subsidy for the nuclear power industry, which “creates absurd investment incentives, grotesquely distorted competition in the power and energy industry and promotes completely unnecessary risks for billions of people”. In percentage terms, the “shadow subsidy” for nuclear power surpasses all other sectors of the economy. Radkau and Hahn come to the same conclusion, who see in renouncing adequate liability insurance the crucial subsidization of nuclear energy, which made the use of nuclear energy possible in the first place.
According to calculations by financial mathematicians, a liability policy for a nuclear power plant would cost 72 billion euros annually. The price of electricity in a nuclear power plant could thus rise to more than forty times.
For the dismantling of nuclear power plants, the operators in Germany (and in Switzerland) have to set up a provision of around 500 million euros per power plant. These provisions remain tax-free in Germany for the entire period and may also be invested, for example, to acquire company shares or to use them in one's own power plant. Critics see the provisions, which now total 36 billion euros, as "the bank of the electricity companies".
In May 2014, plans by the three German nuclear power plant operators E.on, EnBW and RWE were made public to want to transfer their nuclear power plants to a state-owned foundation to be newly established. This should operate the nuclear power plants until the end of their term and then function as a so-called bad bank and pay for the dismantling, final storage and all other risks. For this purpose, the operators want to bring in provisions amounting to around 30 billion euros, and there may be billions in damages due to the nuclear phase-out.
An analysis by the Handelsblatt in 2015 came to the conclusion that nuclear power was "probably the largest and worst investment in the history of the Federal Republic".
France
In France granted Prime Minister Francois Fillon in May 2011, nine weeks after the start of the Fukushima nuclear disaster , the General Accounting Office in France to determine the order, the cost of nuclear energy and the electricity generated. The Court of Auditors presented the report on January 31, 2012. This was the first attempt to determine all French research expenditures in the field of electricity generation from nuclear energy since 1957. Accordingly, the research, development and construction of the 58 French nuclear power plants cost a total of around 188 billion euros (in purchasing power in 2010). These costs have so far been amortized by around 75% through the sale of the electricity (summary, p. 12 below).
However, far not the necessary sums have been set aside for dismantling and interim and final storage of nuclear waste.
In March 2014, the French industry association Uniden demanded a price limit for nuclear power from the French government, as electricity costs for large industrial consumers in Germany would soon be 35% lower than in France.
Great Britain
In Great Britain, a feed- in tariff of 92.5 pounds / MWh (approx. 11.2 cents / kWh) plus annual inflation compensation has been promised by the government for the new Hinkley Point C nuclear power plant . This is roughly double the current UK electricity exchange price and is below the feed-in tariff for large photovoltaic and offshore wind turbines and above the onshore wind turbines. In October 2014, the EU Commission approved the funding commitment as being compatible with EU competition law. The EU Commission is assuming construction costs of 31 billion euros, while the manufacturer and the British government are only talking about 19 billion euros.
United States
The Atomic Energy Commission (AEC) was founded in 1946 and was the central authority for research and development into the utilization of atomic energy until 1974. In 1977 her duties were transferred to the United States Department of Energy.
The Energy Policy Act , passed in 2005, included subsidies and government guarantees to expand nuclear power. On the basis of this law, 32 applications for the construction of new reactors were submitted to the Nuclear Regulatory Commission by January 2008.
Environmental aspects
Comparison with other types of power plants
Based on the 2001 database, the specific greenhouse gas emissions were 15 kilotons per terawatt hour , which is significantly cheaper than coal (around 1,000 kt / TWh) or oil (778 kt / TWh). Wind energy was 9 kt / TWh and photovoltaics was 13 kt / TWh. Run-of-river power has the lowest potential at 2 kt / TWh. According to a study by the Paul Scherrer Institute from 2005, nuclear power plants produced just as little CO 2 as wind energy and less than photovoltaics during their entire life cycle (production, operation, dismantling) .
The sulfur dioxide emissions are 3 tons per terawatt hour and are significantly cheaper than with oil (8013 t / TWh) and coal (5274 t / TWh). In 2001 wind power was 69 t / TWh, photovoltaics 24 t / TWh. River hydropower has the lowest emissions with 1 t / TWh.
The direct land requirements of nuclear energy are 0.5 square kilometers per terawatt hour. In 2001 wind power was 72 km² / TWh (here the (for the most part still usable) space between the systems was taken into account, not the pure stand area), photovoltaics at 45 km² / TWh (without taking into account that in reality mainly already built-up areas / Roofs are used). From this point of view, nuclear energy has the lowest space requirements of all forms of energy.
The harvest factor , i.e. the ratio of energy gained to energy used, is 3 to 7 for photovoltaics and 16 to 25 for wind power. Depending on the location, hydropower has a harvest factor of 10 to 270. The harvest factor for nuclear energy is in a range from 5 to 15, for optimized new plants up to 24. A recent publication sees a trend towards less favorable harvest factors for renewable energies, but gives harvest factors of 75 to 106 for conventional nuclear power plants and even considers values of 2000 to be possible for future nuclear power plants. This study optimistically assumes a nuclear power plant service life of 60 years with a work availability of 91.3% (2009: averaged 74.2% in Germany).
Carbon dioxide emissions
Nuclear fission and nuclear fusion power plants do not generate any CO 2 during operation . However, the use of energy in the manufacture of the power plants, in their operation (in the case of nuclear fission power plants including fuel procurement and waste disposal) and in their demolition is generally associated with CO 2 releases. In 2007, the Scientific Service of the German Bundestag evaluates various sources that quote between 6 and 126 grams of CO 2 per kWh of electricity generated. Electricity from coal-fired power plants is around 950 g / kWh (hard coal) and 1150 g / kWh (lignite). In view of the estimation uncertainties, the scientific service comes to the conclusion that "various forms of renewable energies, but also nuclear power" belong to the "top group" of low- CO 2 energy sources .
The total amount of CO 2 released over the entire life cycle is therefore significantly lower in nuclear power plants than when the same amount of electricity is generated using conventional (fossil-fuel-fired) power plants. Similar CO 2 reduction factors can be achieved with wind power and hydropower plants , while other renewable energies , in particular photovoltaics , only achieve slightly smaller CO 2 reduction factors.
The CO 2 emissions from nuclear fission energy depend on the uranium content of the ore - there are currently between 1,000 and 40,000 tons of overburden per ton of uranium oxide - and the selected uranium enrichment process . The limited nature of uranium means that ores with ever lower uranium content are increasingly being processed as fuels. This increases the use of non-CO 2 -neutral energy sources for uranium mining and enrichment, the CO 2 emissions per kWh of electricity. It should also be noted that none of the studies cited improve the burn-up parameters of newly designed nuclear power plants, such as B. the EPR , which should lead to a reduction in the use of uranium while maintaining the same output.
Comparison between Germany and France
Not all power plants generate in operating CO 2 , but is created in the production, basically in the operation and they were demolished and climate-damaging CO 2 . The total amount released (over the entire life cycle) is very different, as the following table shows. The fields highlighted in red show that coal-fired power plants in Germany only generate 47% of the total electrical energy, but cause 80% of the carbon dioxide generated. The CO 2 share that nuclear power plants indirectly contribute to 22.6% electricity generation is very low at 0.7%. The current distribution in neighboring France is compared in the two right-hand columns.
Nuclear fusion power plants will not generate CO 2 during operation, nor during fuel production and processing , but during their construction and dismantling (comparable to nuclear fission power plants).
Type of power plant | CO 2 emissions per kWh in grams | Share of total gross electricity generation (2015) in Germany | Share in the CO 2 generation of all power plants in Germany | Share of the total electr. Energy (2007) in France | Share in the CO 2 production of all power plants in France |
---|---|---|---|---|---|
Hydropower | 10-40 | 3.0% | 0.06% | 8.8% | 1.2% |
Wind energy | 10-40 | 13.5% | 0.12% | 0% | - |
Nuclear power plant (fission) | 10-30 | 14.1% | 0.7% | 86.6% | 27.8% |
Photovoltaic | 50-100 | 5.9% | 0.1% | 0% | - |
natural gas | 400-550 | 9.1% | 8.1% | - | - |
oil | 890 | - | 1.9% | - | - |
Hard coal | 790-1080 | 18.1% | 35.3% | 4.6% | 71% |
Brown coal | 980-1230 | 23.8% | 44.9% | - | - |
others (garbage, biomass, ...) | 12.5% | 8.9% | - | - | |
Electricity mix in Germany (2007) | 604 | - | - | - | - |
A comparison of the power plant parks in the neighboring countries of France and Germany shows which savings are possible through political stipulations : Although CO 2 is also released in France by the 15 coal-fired power plants, the total amount is considerably lower, as the following table shows. According to the EDF 95% of the electrical energy in France CO 2 - free generated. With almost the same total electrical energy, France does not even produce 10% of the greenhouse gas CO 2 released in Germany . The energy industry causes less than half of the anthropogenic CO 2 emissions. In terms of per capita emissions , France is around a third lower than Germany (2008).
Country | Total generation of all power plants in TWh |
Electricity mix g per kWh | Total CO 2 in billions of kg |
Number of large fossil- thermal power plant units |
Number of nuclear power plant blocks |
---|---|---|---|---|---|
Germany | 636.5 | 604 | 384 | ≈70 | 17th |
France | 610.6 | 61 | 37 | 15th | 58 |
Historical impact on CO 2 emissions and competition with renewable energies
A scientific study published in October 2020 (Sovacool et al.) With data on 123 countries over 25 years stated that a trend towards significantly reduced CO 2 emissions through nuclear power could not be statistically proven, while this was possible for renewable energy sources. However, an analysis of the same data by another group of scientists found that the CO 2 savings from nuclear power and renewable energy sources are comparably high, and that this is statistically significant from the data, in line with the theoretical savings potential. The discrepancy between the two results was due to serious methodological errors in the data analysis by Sovacool et al. in particular the misinterpretation of statistical hypothesis tests and the influence of countless unobserved disruptive factors . Sovacool et al. however, explained their unexpected result with tensions between various national development strategies, which could reduce their climate protection effectiveness - for example due to different infrastructure requirements and limited budgets for investments in the energy system. The social allocation of resources for nuclear energy production could compete with the allocation of resources for research, development, construction, expansion and improvement of technologies and structures in connection with renewable energies.
Risk of accident (risk of a core meltdown)
In the first "German Risk Study" from 1979, prepared by the Society for Reactor Safety , the possible consequences of accidents of up to 14,500 immediate deaths and 104,000 later deaths are given with a probability of occurrence of once every two billion years. According to the Society for Reactor Safety, an area of up to 5600 square kilometers could be so heavily contaminated that 2.9 million people would have to be evacuated and resettled. In the phase B risk study, the Biblis B pressurized water reactor has a probability of an accident with a core meltdown of 3.6 · 10⁻⁶ per year. This roughly corresponds to one accident every 280,000 years of operation. The expected value is therefore just under 0.5 deaths / year of operation.
During the Chernobyl disaster , the largest nuclear accident in history to date, large areas of land - also in Germany - were contaminated with radioactive nuclides in 1986. Almost 50 people died as a result of the high levels of radiation . The prognosis of deaths from radiation-induced cancer involves great uncertainties; the estimates of the World Health Organization and the International Atomic Energy Agency are in the order of magnitude of a total of 9,000 additional deaths. Other organizations estimate a far higher number of victims: Greenpeace names 200,000 additional deaths as a result of the accident, the IPPNW determined a number of 50,000 to 100,000 deaths and 540,000 to 900,000 disabled persons by 2006, and the TORCH report names 30,000 to 60,000. These extrapolated numbers of victims are based on the nuclear medicine controversial Linear No Threshold model , which states that a certain dose of ionizing radiation is essentially always equally harmful, regardless of the period of time over which it was absorbed. There are now some retrospective studies that cast doubts on the correctness of the LNT model.
A study by Prognos AG in 1992 put the damage caused by an accident with a significant release of radioactivity at 5 to 12 trillion DM (2.6 to 6.1 trillion €), corresponding to three to four times the annual German gross national product at the time . Since such an accident can only be expected once in around 1700 years in Germany, the “expected damage value” is 6.4 billion marks (3.3 billion €) per year, or 4.3 pfennigs (2 cents) per kilowatt hour of nuclear power .
In May 2012 a study by the Max Planck Society for Chemistry was published, according to which the risk of catastrophic core meltdowns in reactors such as in Chernobyl (outdated RBMK type without containment) and Fukushima (BWR / 3-5 type without adequate flood and earthquake protection, built in a tsunami and earthquake-prone area) is much higher than previously estimated, namely once in 10 to 20 years or 200 times more frequently than previously assumed. Whether nuclear power plants such as in Chernobyl or Fukushima are representative of all nuclear power plants worldwide remains open in the article, especially since a global trend towards passive safety is emerging in the construction of new nuclear power plants. Most of the nuclear power plants currently under construction (as of 2020) such as the AP1000 from Westinghouse, the Russian WWER 1200, the Korean APR1400 , the Indian IPHWR-700 or the European EPR are equipped with extended passive safety features. Such reactors can compensate for a loss of coolant over several days without an external power supply only through passive cooling, without a core meltdown occurring. An accident like the one in Fukushima would be ruled out.
In addition, in the USA, for example, the frequency of unplanned reactor shutdowns has fallen by a factor of around 25 since the reactor accident at the Three Mile Island nuclear power plant . Globally, the number of unplanned reactor shutdowns has fallen to around a third since 1990, and the number of industrial accidents in nuclear power plants to a fifth. The aforementioned trends were not taken into account in the above-mentioned study by the Max Planck Society for Chemistry.
Pressures from uranium mining
The mining of uranium is linked to negative environmental influences that occur both during the mining itself and also remain effective in the long term after the mining activity has been completed due to the mostly inadequately secured mining legacies. Uranium is used primarily in the day - and underground mining mined, with the bulk of the uranium comes from states that "apply their mining environmental standards as underdeveloped". In countries such as Russia, Canada, Niger or Kazakhstan, there are no additional requirements for handling residue landfills. This goes hand in hand with land use, water consumption and pollution as well as general environmental pollution and health hazards for mine workers and the affected population. The resulting release of and exposure to radioactivity , which has led to increased (lung) cancer cases in the history of uranium mining, is specific to the extraction of uranium. Anti- nuclear opponents criticize the fact that the CO 2 emissions in uranium mining are not taken into account when considering the ecological balance of nuclear energy.
In Australia, Aborigines are particularly affected by cancer near uranium mining sites. Also, the uranium mining in Germany (in the former GDR , the reunification in 1990 set, see bismuth ) resulted in diseases of miners . Through reports, medical dossiers and litigation files, this uranium mining is considered to be the best documented in the world.
Loads from normal operation
A study by the Federal Office for Radiation Protection from 2007 shows a statistically significant increase in the incidence of leukemia in children who grew up less than five kilometers from a nuclear power plant. According to this, from 1980 to 2003 37 children within a five-kilometer radius of the nuclear power plants in Germany fell ill with leukemia - the statistical average would have been 17 children. The reasons for this correlation have not yet been clarified; according to the current state of knowledge, the connection cannot be explained using radiation biology.
There is no consensus on the interpretation of this finding. While the authors of the study are of the opinion that the ionizing radiation emitted by German nuclear power plants during normal operation cannot be considered as the cause due to the many times higher natural radiation exposure, the external expert committee of the BfS for the KiKK study is convinced that due to the particularly high radiation risk for small children as well as insufficient data on emissions from power reactors, this connection can in no way be ruled out. Other studies, however, are controversial. They show no or even a clear connection between living near a nuclear power plant and the occurrence of cancer cases. It is also pointed out that 'many possibly combined factors ... are (are) conceivable causes of disease and ... may occur (or occur) in the vicinity of German nuclear power plants', so these are not yet unknown emissions from power reactors.
Plutonium and unused uranium as well as other radioactive materials (for example americium) contained in spent fuel are extracted in the French reprocessing plant in La Hague and in Sellafield in the UK ; the remaining residues are conditioned for final disposal. This process produces 400 cubic meters of radioactive waste water every day in La Hague; this is directed into the English Channel . This ("direct introduction") is legal; Sinking barrels with nuclear waste in the sea has been banned since 1993.
Unsolved disposal
The disposal of the highly radioactive fuel elements or the residues from reprocessing is still unsecured. As of 2012, there was no repository for highly radioactive substances anywhere in the world. The reasons given for this are:
- The underestimation of the task
- Unrelated considerations when setting the task
- Lack of public and political acceptance of the projects
- a controversy about the fundamental suitability of final disposal and its risks for coping with the problem
For decades, Germany has been making do with a large number of so-called interim storage facilities and "test storage facilities" such as the well-known Asse mine to store the radioactive waste that has accumulated up to now . On July 27, 2013, a new Site Selection Act (StandAG) came into force, after which new proposals for safety requirements and geological exclusion and selection criteria are to be drawn up by the end of 2015. Only then does a new location search take place. Because some of the accumulating nuclides have very long half-lives ( 239 Pu for example 24,000 years), the requirements in particular for long-term geological stability are correspondingly high. It is true that the half-life can be reduced to a few hundred years through reprocessing and transmutation ; however, this also increases the current radiation exposure associated with such procedures.
According to various organizations and experts, even the transport of nuclear waste is not safe because of possible accidents. Plutonium extracted during reprocessing can be used to make nuclear weapons . In addition, there were media reports, in particular about the Sellafield reprocessing plant , that radioactivity had escaped uncontrolled there and that the families living in the vicinity were confronted with some cases of leukemia- related diseases in their children and senior citizens. Such a connection has not yet been scientifically confirmed.
By the 1970s, around 100,000 barrels of radioactive waste were dumped on the high seas, some in fishing areas. The consequences for ecology and human nutrition are classified as negligible due to the high dilution. Some scientists have suggested in the past that the dumping of nuclear waste in the world's oceans should be taken up again, since the contribution of natural radioactive isotopes dissolved in seawater is arithmetically about five million times greater than that of the dumping of all of America's nuclear waste.
Comparison with other sources of danger
The different health effects of different forms of electricity generation are difficult to attribute. According to an estimate based on data from the European Union , the number of public deaths caused by nuclear power in Europe is 0.003 and among those employed in the nuclear energy sector it is 0.019 (each per terawatt hour generated ). Deaths from air pollution due to the use of nuclear energy are 0.052 and serious illnesses are 0.22. In comparison, the number of deaths from electricity generation with lignite is 0.02 (accidents in public), 0.1 (accidents among employees), 32.6 (air pollution) and 298 (serious illnesses). The authors therefore do not see air pollution and normal operation, which caused comparatively few deaths, as more significant problems of nuclear energy, but rather long-term dangers associated with the storage of nuclear waste, military use and damage in the event of an accident. This assessment was also made after the nuclear disaster in Fukushima et al. Confirmed by James E. Hansen . In a study published in 2013, he compared the risks of various energy sources and advocated nuclear energy as a technology with significantly lower risk and lower emissions.
Threats to Peace and Security
Nuclear Weapons Proliferation - Abuse
Opponents of nuclear power argue that it is not possible to effectively separate civil and military uses. Nuclear energy contributes to the diffusion of technology and materials for the manufacture of nuclear weapons, especially the enrichment and reprocessing plants that produce weapons-grade plutonium. India , North Korea and South Africa started civil nuclear programs with special research reactors . It is controversial whether plutonium suitable for weapons was produced in these or in special plants. South Africa has since given up its nuclear weapons. Iran and Israel have recently had no nuclear power plants for commercial energy generation. South Africa started operating its only commercial nuclear power plant to date long after acquiring nuclear weapons. John Large, a leading nuclear energy expert in Great Britain, says: Every civil nuclear program is per se suitable for hiding a weapons program [...] In many areas, military and civil use can hardly be distinguished.
Plants for 235 uranium enrichment , such as the German uranium enrichment plant in Gronau , could also be used to produce material suitable for nuclear weapons , with a share of 80% 235 U.
During the production of nuclear fuel rods, the proportion of the fissile uranium isotope 235 for use in most reactor types (but not in heavy water reactors and some graphite-moderated reactor designs) must be increased from the natural proportion of 0.7% to around 4% (" uranium enrichment ") ) so that it is able to start a chain reaction. Nuclear opponents fear that uranium enrichment plants could be converted at any time so that weapons-grade uranium with around 80% uranium-235 content could be produced there. The techniques used in reprocessing plants are in principle also suitable for extracting plutonium from spent fuel rods, which can also be used to generate energy in MOX fuel assemblies . The suitability of the extracted plutonium for weapons decreases as the fuel elements burn up . But a nuclear explosive device of lower efficiency can still be produced from plutonium from highly spent fuel elements.
Many technologies related to civilian nuclear energy are also relevant to the development and manufacture of nuclear weapons . Therefore, if a state so wishes, civilian nuclear energy programs can be used as a cover for a secret military nuclear weapons program. The Iranian nuclear program is one of the prominent examples of this.
A fundamental goal of national and global security efforts is to minimize the proliferation risk associated with global use and the expansion of civilian use of nuclear energy. If the development “is poorly implemented or the measures to contain the risk of poliferation fail, it will be dangerous in the future”. The Global Nuclear Energy Partnership is an approach to make countries with a need for nuclear fuel available at low cost. In return, the states undertake to forego their own uranium enrichment programs.
Benjamin K. Sovacool said some "senior officials, even within the United Nations, have argued that there is little they can do to discourage states from using nuclear reactors for the manufacture of nuclear weapons." A 2009 report from the United Nations said:
“The renewed interest in the use of nuclear energy could lead to the worldwide spread of technologies for uranium enrichment and recycling. This poses a clear risk of proliferation as these technologies can generate fissile material that can be used directly in nuclear weapons. "
On the other hand, power reactors can be used to reduce nuclear arsenals. As part of the megaton-to-megawatt program , 425 tonnes of highly enriched uranium from former nuclear weapons have been processed into nuclear fuel for reactors. This corresponds to around 17,000 nuclear warheads. This makes it the most successful anti-proliferation program to date.
Professor Matthew Bunn says:
“Russia is no longer interested in continuing the program after 2013. We set it up so that it costs them more and they get less from it than if they just made new reactor fuel. But there are other options that would make the whole thing more profitable and would also serve your strategic interests of expanding your nuclear exports. "
In April 2012 there were nuclear power plants in 31 countries . In 2013, Mark Diesendorf said the governments of France, India, North Korea, Pakistan, England and South Africa have used power and research reactors to develop nuclear weapons or to expand nuclear weapon stocks from military reactors.
The development of new reactor systems and associated fuel cycles by the Generation IV International Forum has the explicit aim of making the removal of nuclear weapons-grade or terrorist-usable material as unattractive as possible.
Risk of terrorist attacks
Nuclear power plants are considered targets for terrorist attacks, although these findings have not only been discussed since the 9/11 attacks . Safety committees pointed out this problem as early as the construction of the first nuclear power plants. Specific threats to attack nuclear power plants by terrorists or criminals have also been documented from several countries. While older nuclear power plants were built in Germany without any special protection against air accidents, the later built nuclear power plants are partially secured against air accidents with a massive concrete building. They are designed to withstand the impact of combat aircraft at a speed of around 800 km / h. The impact of a Phantom II aircraft with a mass of 20 tons and a speed of 215 m / s was assumed as the basis for assessment .
The dangers that arise from a terrorist attack by means of a large aircraft on a nuclear power plant are now also being discussed. Such a terrorist attack could have catastrophic consequences. For example, the federal government has confirmed that the Biblis A nuclear power plant is not secured against the crash of a military aircraft. After the terrorist attacks in Brussels in 2016, several nuclear power plants were partially evacuated. At the same time it became known that the terrorists had also spied on the nuclear power plants. Access authorizations were withdrawn from several employees.
In addition, "nuclear terrorism", z. For example, the use of so-called "dirty bombs" by terrorists represents a considerable risk potential. Any radioactive waste or uranium enriched for nuclear power plants could be used for their production, but by far the greatest threat potential is the existence of radioactive substances in nuclear medicine . because the supervision and monitoring of nuclear medicine stocks is nowhere near as strictly organized as in the electricity-generating nuclear industry. The theft of nuclear medicine equipment and containers alone has in the past led to incidents with considerable contamination and a considerable number of radiation victims (some of them resulting in death), for example the Goiânia accident or the nuclear accident in Samut Prakan
Nuclear Energy Controversies

The discussion about nuclear energy is a societal dispute about the nuclear fission used for civil purposes to generate electricity from nuclear fuels. The discussion reached a high point in the 1970s and 1980s; in some countries at that time nuclear energy was discussed more intensely than a technology had ever been before. In Germany in particular, the anti-nuclear movement was firmly anchored in society for decades: it was given a political platform initially by the Greens , and later by the SPD . Their work led to Germany's pioneering role in phasing out nuclear power .
Proponents see nuclear energy as a sustainable technology that increases security of supply as it reduces dependence on imports of fossil fuels. Proponents have emphasized that using nuclear energy produces a much lower amount of greenhouse gases or smog than fossil-fuel power plants. In contrast to certain renewable energies , in particular wind energy and solar energy , which can only achieve high proportions of the electricity mix in combination with storage power plants, nuclear energy is base load capable . Proponents have claimed that the risk associated with disposal is small and can be further reduced by using advanced technology. The (historical) safety record of nuclear energy in the western world is excellent compared to other large energy sources.
Opponents of nuclear energy argue that it implies many dangers for humans and the environment. There were and are problems with the processing, transport and storage of radioactive waste , the risk of proliferation and terrorism, as well as health risks and risks from uranium mining .
They point out that malfunctions and operating errors are possible and unavoidable in the long run in nuclear power plants (see also list of accidents ). The risks of nuclear energy cannot be completely eliminated through further technical developments. Taking into account the entire chain from uranium mining to final storage and dismantling of the individual nuclear power plants, nuclear energy is neither a CO 2 -neutral nor an economic source of energy. Further points of criticism are the limited use of nuclear fuels and the dependence on uranium supplier countries. The fact that the nuclear power plant operators in Germany from 1979 to 2017 earned money from the disposal of their own waste through their participation in the German Society for the Construction and Operation of Final Storage for Waste Materials (DBE) has been criticized.
Arguments about the economic viability of nuclear energy have been put forward by both sides.
In the years 2006 to 2008, around half of the German population was against nuclear energy.
See also
literature
- Ian Hore-Lacy: Nuclear Energy in the 21st Century: World Nuclear University Press . Academic Press, 2006, ISBN 0-12-373622-6
- Paul Laufs: Reactor Safety for Power Nuclear Power Plants , Springer Vieweg, Berlin / Heidelberg 2013, ISBN 978-3-642-30654-9
- Raymond L. Murray: Nuclear Energy, Sixth Edition: An Introduction to the Concepts, Systems, and Applications of Nuclear Processes . Butterworth-Heinemann, 2008, ISBN 978-0-12-370547-1
- Julia Mareike Neles, Christoph Pistner (ed.): Nuclear energy. A technology for the future? Springer, Berlin / Heidelberg 2012, ISBN 978-3-642-24329-5
- Joachim Radkau, Lothar Hahn : The rise and fall of the German nuclear industry . Oekom-Verlag, Berlin 2013, ISBN 978-3-86581-315-2
- Christoph Wehner: The nuclear hazard insurance. Risk policy, security production and expertise in the Federal Republic of Germany and the USA 1945–1986 . Wallstein, 2017, ISBN 978-3-8353-3085-6
Web links
-
deutschlandfunk.de , Environment and Consumers , July 14, 2017, Mycle Schneider in conversation with Susanne Kuhlmann : Development of atomic energy worldwide: "It's China and the rest of the world"
- Science in focus , July 16, 2017, Dagmar Röhrlich : Brave new reactor world
- International Atomic Energy Agency (IAEA): iaea.org (English)
- International Nuclear Risk Assessment Group ( International Association for the Evaluation of nuclear risks ): inrag.org (English)
- German Atomic Forum : kernenergie.de (lobby group of operators of nuclear power plants and the nuclear industry)
- Lexicon on nuclear energy 2017 by Winfried Koelzer
Individual evidence
- ↑ Trend in Electricity Supplied. PRIS (IAEA database), accessed on July 3, 2017 (English).
- ↑ a b c PRIS - Power Reactor Information System, Operational & Long-Term Shutdown Reactors. iaea.org, accessed January 9, 2020 .
- ^ PRIS - Power Reactor Information System, Under Construction Reactors. iaea.org, accessed January 9, 2020 .
- ↑ Information from Statista.com [1]
- ↑ PRIS - Power Reactor Information System, Permanent Shutdown Reactors. iaea.org, accessed January 9, 2020 .
- ↑ Fig 7: World Nuclear Reactor Fleet, 1954-2019. In: The World Nuclear Industry Status Report 2019. Mycle Schneider Consulting, September 2019, accessed January 9, 2020 .
- ↑ Spiegel Online: Atomic energy is losing importance worldwide from July 6, 2012; Accessed July 9, 2012.
- ↑ a b Fig 3: Nuclear Electricity Production 1985-2018 in the World ... In: The World Nuclear Industry Status Report 2019. Mycle Schneider Consulting, September 2019, accessed on January 9, 2020 .
- ^ Nuclear Power in the European Union. World Nuclear Association, December 2019, accessed January 10, 2029 .
- ^ Nuclear Power Plants & Nuclear Reactors - Nuclear Power in the World Today. engineersgarage.com, archived from the original on October 4, 2013 ; Retrieved April 22, 2013 .
- ↑ Christopher Schrader: Upswing of the atoms. In: Süddeutsche Zeitung. June 4, 2008, p. 18, with a paragraph on the history of the concept; cf. for the history of the term in general: Matthias Jung: Public and Language Change. On the history of the discourse on atomic energy. Westdeutscher Verlag, Opladen 1994, ISBN 3-531-12392-0 . (i.e. thesis at Heinrich-Heine-Universität Düsseldorf 1992: The nuclear controversy as language history of the present )
- ↑ Werner Heisenberg : About the work on the technical utilization of nuclear energy in Germany. In: The natural sciences. Issue 11, 1946, p. 326.
- ↑ Joachim Radkau : Rise and Crisis of the German Nuclear Industry 1945-1975. Replaced Alternatives in Nuclear Technology and the Origin of the Nuclear Controversy. Hamburg 1983, p. 462f.
- ↑ see Radkau / Hahn in the literature list
- ^ Joachim Radkau: Technology in Germany. From the 18th century until today. Frankfurt / New York 2008, p. 359.
- ^ Joachim Radkau: Technology in Germany. From the 18th century until today. Frankfurt / New York 2008, p. 360.
- ^ Result of the referendum on the Zwentendorf nuclear power plant. federal Ministry of Internal Affairs
- ↑ Law on the orderly termination of the use of nuclear energy for the commercial generation of electricity. ( Memento from October 20, 2016 in the Internet Archive ) (PDF; 707 kB) at BMWi
- ↑ PRIS - Nuclear Power Capacity Trend
- ↑ Nuclear Power Reactors in the World - 2012 Edition. (PDF file; 794 kB) IAEA , June 1, 2012, p. 20 , accessed on May 18, 2013 (English).
- ↑ The World Nuclear Industry Status Report 2011. (PDF; 4.1 MB); see also interview with energy policy researcher Lutz Mez and the International Atomic Energy Agency (IAEA): Power Reactor Information System (PRIS) ( Memento from January 27, 2012 in the Internet Archive ); International Atomic Energy Agency (IAEA): International Status and Prospects of Nuclear Power. GOV / INF / 2008/10-GC (52) / INF / 6, August 12, 2008; atw Schnellstatistik Kernkraftwerke 2008. atw, 54th year, issue 1, January 2009.
- ↑ USA: Obama relies on nuclear power. In: Süddeutsche Zeitung . May 17, 2010, accessed April 22, 2013 .
- ^ Nuclear Power in the USA. World Nuclear Association, November 22, 2013, accessed December 24, 2013 .
- ↑ a b c Nuclear Power's Global Fallout. In: Science. Volume 331, March 25, 2011, pp. 1502-1503.
- ^ Nuclear Power in India. World Nuclear Association, December 1, 2013, accessed December 24, 2013 .
- ^ Nuclear Power in China. World Nuclear Association, December 1, 2013, accessed December 24, 2013 .
- ↑ China is now putting reactor construction on hold . In: FAZ. March 16, 2011. Retrieved September 10, 2011.
- ↑ China continues to rely on nuclear power. In: www.heise.de. July 5, 2011. Retrieved February 7, 2011.
- ^ Nuclear Power in Russia. World Nuclear Association, November 29, 2013, accessed December 24, 2013 .
- ↑ Italians say no to nuclear power - and to Berlusconi. In: Spiegel-online. June 13, 2011. Retrieved September 10, 2011.
- ↑ Import instead of export: the Czech Republic wants to change energy policy. Radio Prague, June 26, 2014.
- ↑ Bundestag : Lifetime extension of nuclear power plants approved. There links to the two amendments to the Atomic Energy Act (17/3051, 17/3052), the establishment of an energy and climate fund (17/3053) and the Nuclear Fuel Tax Act (17/3054)
- ↑ Because of the reactor accident in Fukushima: Japan announces nuclear phase-out by 2040 at focus.de, September 14, 2012 (accessed on September 14, 2012).
- ↑ Japan reactivates its nuclear power plants. n-tv , August 10, 2015, accessed on April 11, 2014 .
- ↑ Japan is starting up the reactor for the first time since the Fukushima Gau. In: Frankfurter Allgemeine Zeitung . August 10, 2015, accessed August 15, 2015 .
- ↑ nuclear energy. In: Brockhaus Encyclopedia. 21st edition. 2006.
- ^ A b OECD : Uranium: Resources, Production and Demand (The Red Book). (PDF) 2014, accessed on July 11, 2016 .
- ↑ UxC. July 11, 2016. Retrieved July 11, 2016 .
- ↑ Uranium as a nuclear fuel: supplies and range. ( Memento from May 13, 2013 in the Internet Archive ) on: bundestag.de (PDF; 782 kB)
- ^ Naturally-Occurring Radioactive Materials . World Nuclear Association website . Retrieved July 8, 2014.
- ↑ 239 Pu , the most commonly produced isotope of plutonium, has a half-life of 24,110 years
- ↑ DAtF Deutsches Atomforum eV: Take- back of waste from reprocessing (page 3). November 1, 2018, accessed January 9, 2019 .
- ^ Reprocessing in La Hague. on: greenpeace.de
- ↑ arte TV: Nightmare nuclear waste . Documentary by Eric Guéret & Laure Noualhat (German broadcast October 15, 2009); Watch it on YouTube
- ↑ Schwandorf district: 105.48.1 WAA Wackersdorf 1980–1989 , www.landkreis-schwandorf.de (October 26, 2006)
- ↑ Nuclear waste under fire. on: faz.net April 25, 2006, last accessed on March 17, 2011.
- ↑ Myrrha Project
- ↑ IEA forecast: decommissioning of nuclear power plants could cost 100 billion dollars. In: Spiegel online. November 12, 2014.
- ↑ Nuclear fusion - a “clean” energy? on: scinexx.de, the knowledge magazine. March 26, 2000.
- ↑ EEG 2014 § 1 sentence (2).
- ↑ Federal Environment Agency, expansion targets for electricity from renewable energies , February 7, 2015.
- ↑ Umweltinstitut München, Nuclear Fusion - Expensive and Superfluous ( Memento from April 6, 2016 in the Internet Archive ), July 2013.
- ↑ Anatol Hug: Nuclear Fusion: You Must Know That . Swiss Radio and Television - Knowledge, March 23, 2015.
- ↑ Julia Mareike Neles, Christoph Pistner (ed.): Nuclear energy. A technology for the future? Berlin / Heidelberg 2012, p. 210.
- ↑ Panos Konstantin: Praxishandbuch Energiewirtschaft. Energy conversion, transport and procurement in the liberalized market . Berlin / Heidelberg 2009, p. 302.
- ↑ Nuclear dispute: Minimal savings through longer nuclear power plant runtimes. In: time online. July 18, 2008, accessed on March 2, 2014 (the number is given at the beginning of the last paragraph.).
- ^ Vermont Yankee Plant to Close Next Year as the Nuclear Industry Retrenches . In: New York Times , August 27, 2013. Retrieved June 8, 2014.
- ↑ Terium warns of the economic end for nuclear power . In: Handelsblatt , January 20, 2014. Retrieved June 8, 2014.
- ↑ Julia Mareike Neles, Christoph Pistner (ed.): Nuclear energy. A technology for the future? Berlin / Heidelberg 2012, p. 213 f.
- ↑ a b Because of the renaissance of nuclear power . In: Frankfurter Allgemeine Zeitung , February 6, 2010. Accessed June 8, 2014.
- ^ New Nuclear - The Economics Say No. Citibank, November 2009, PDF, accessed March 31, 2015.
- ^ Off for new reactors in Temelín . In: Süddeutsche Zeitung , April 10, 2014. Accessed June 8, 2014.
- ↑ Poland postpones its nuclear plans . In: Märkische Oderzeitung , June 30, 2013. Accessed June 8, 2014.
- ^ Reviving nuclear power debates is a distraction. We need to use less energy ( Memento from November 8, 2013 in the Internet Archive ). In: The Guardian , November 8, 2013. Retrieved June 8, 2014.
- ↑ Julia Mareike Neles, Christoph Pistner (ed.): Nuclear energy. A technology for the future? Berlin / Heidelberg 2012, p. 211.
- ^ Frank Uekötter : Fukushima, Europe, and the Authoritarian Nature of Nuclear Technology. In: Environmental History. 17, April 2012, p. 279.
- ↑ Panos Konstantin: Praxishandbuch Energiewirtschaft. Energy conversion, transport and procurement in the liberalized market . Berlin / Heidelberg 2009, p. 300.
- ↑ Panos Konstantin: Praxishandbuch Energiewirtschaft. Energy conversion, transport and procurement in the liberalized market . Berlin / Heidelberg 2009, pp. 300-302.
- ↑ Olkiluoto 3 to be ready in 2018 . In: Helsinki Times , October 9, 2014. Retrieved October 9, 2014.
- ↑ Nuclear reactor becomes grave of billions. EDF lays a shiny cuckoo egg in Hollande's nest . In: Handelsblatt , December 5, 2012. Retrieved December 5, 2012.
- ↑ The grave of billions. taz, December 19, 2012, accessed December 19, 2012 .
- ↑ France and China build nuclear power plants in Great Britain . In: Frankfurter Allgemeine Zeitung , October 21, 2013. Accessed June 8, 2014.
- ↑ Conversion using the exchange rate from April 23, 2021.
- ^ Electricity Market Reform - Delivery Plan. (PDF file, 1.5 MB) Department of Energy and Climate Change, December 2013, accessed on May 4, 2014 .
- ↑ a b Carsten Volkery: cooperation with China: Britain is building the first nuclear power plant in decades . In: Spiegel online. 21st October 2013.
- ↑ USA approve new nuclear reactors for the first time . In: Focus , February 10, 2012. Retrieved June 10, 2014.
- ↑ DOE Loan Guarantee Program: Vogtle Reactors 3 & 4 . www.taxpayer.net. Retrieved June 10, 2014.
- ↑ Expansion of the Mochovce nuclear power plant stopped by the Supreme Court . In: Der Standard , August 21, 2013. Retrieved June 10, 2014.
- ↑ China seen buying Westinghouse reactors for $ 24 billion nuclear energy projects . Reuters, April 21, 2014. Retrieved June 10, 2014.
- ↑ a b c d Julia Mareike Neles, Christoph Pistner (ed.): Nuclear energy. A technology for the future? Berlin / Heidelberg 2012, p. 216.
- ^ Frank Uekötter: Fukushima, Europe, and the Authoritarian Nature of Nuclear Technology. In: Environmental History. 17, April 2012, p. 282.
- ↑ a b Researchers give green electricity a good certificate . In: Frankfurter Allgemeine Zeitung , July 17, 2013. Accessed June 8, 2014.
- ^ A b Frank Uekötter: Fukushima, Europe, and the Authoritarian Nature of Nuclear Technology. In: Environmental History. 17, April 2012, p. 279.
- ^ Joachim Radkau: Technology in Germany. From the 18th century until today . Frankfurt / New York 2008, p. 372.
- ^ Frank Uekötter: Fukushima, Europe, and the Authoritarian Nature of Nuclear Technology. In: Environmental History. 17, April 2012, p. 278.
- ↑ Hans-Joachim Braun : Energy generation. In: Ulrich Troitzsch , Wolfhard Weber (ed.): The technology. From the beginning to the present. Braunschweig 1982, p. 396.
- ↑ Jean-Claude Debeir, Jean-Paul Déléage, Daniel Hémery: Prometheus on the Titanic. History of Energy Systems . Frankfurt am Main 1989 (original: Paris 1986), p. 294.
- ↑ Jean-Claude Debeir, Jean-Paul Déléage, Daniel Hémery: Prometheus on the Titanic. History of Energy Systems . Frankfurt am Main 1989 (original: Paris 1986), p. 301.
- ^ Frank Uekötter: Fukushima, Europe, and the Authoritarian Nature of Nuclear Technology. In: Environmental History. 17, April 2012, p. 280 f.
- ^ New Nuclear - The Economics Say No. Citibank, November 9, 2009, inserted March 16, 2012, accessed January 25, 2015.
- ↑ Hertie School: Major Projects in Germany - Between Ambition and Reality
- ^ Nuclear Energy Loses Cost Advantage : "Solar photovoltaics have joined the ranks of lower-cost alternatives to new nuclear plants," John O. Blackburn, a professor of economics at Duke University, in North Carolina, and Sam Cunningham, a graduate student, wrote in the paper, "Solar and Nuclear Costs - The Historic Crossover." In: New York Times . July 26, 2010.
- ↑ Law on the Peaceful Use of Nuclear Energy and Protection against its Dangers.
- ↑ Gerd Winter : The Rise and Fall of Nuclear Energy Use in Germany: Processes, Explanations and the Role of Law. In: Journal of Environmental Law. 25, No. 1, March 2013, p. 115 f.
- ↑ Government study: Nuclear accident would cost France € 430 billion. on: Spiegel online. February 7, 2013.
- ↑ Tricky mission in the nuclear ruin. In: Hannoversche Allgemeine Zeitung . November 18, 2013, accessed June 19, 2014.
- ↑ Generating electricity from coal at times of low electricity prices. ( Memento of October 16, 2013 in the Internet Archive ) (PDF; 1.9 MB). Fraunhofer ISE . Retrieved May 20, 2014.
- ↑ Consumer protection: Electricity customers save only 50 cents a month with nuclear power. on: Spiegel online. July 7, 2008.
- ↑ a b c d e f g h German Institute for Economic Research: Final report on the project “Expert discussion to take stock and methodical evaluation of existing approaches to quantify the promotion of renewable energies in comparison to the promotion of nuclear energy in Germany”. ( Memento of March 27, 2014 in the Internet Archive ) May 2007, accessed on September 22, 2010.
- ↑ Text of the Atomic Energy Act (Germany)
- ↑ Gerd Winter: The Rise and Fall of Nuclear Energy Use in Germany: Processes, Explanations and the Role of Law. In: Journal of Environmental Law. 25, No. 1, March 2013, p. 123.
- ↑ nuclear energy. European Parliament, accessed June 14, 2014 .
- ↑ Official Journal of the EU (PDF). (COM (2011) 0226 - C7-0108 / 2011 - 2011/2080 (ACI)). Retrieved June 19, 2014.
- ↑ 26 billion for coal, oil and gas . In: ORF , July 24, 2013. Retrieved July 24, 2013.
- ↑ EU: More money for atom than for eco ( Memento from October 10, 2014 in the Internet Archive ). In: Kleine Zeitung , July 24, 2013.
- ↑ a b Joachim Radkau, Lothar Hahn: Rise and fall of the German nuclear industry. Oekom-Verlag, Berlin (2013).
- ↑ EU should promote nuclear power . In: Frankfurter Rundschau . April 13, 2012, Retrieved April 13, 2012.
- ↑ Competition with renewable energies. EU states are demanding subsidies for nuclear power . In: Süddeutsche Zeitung . April 13, 2012, Retrieved April 13, 2012.
- ^ Joachim Radkau: Technology in Germany. From the 18th century until today . Frankfurt / New York 2008, p. 355.
- ^ Joachim Radkau: Technology in Germany. From the 18th century until today . Frankfurt / New York 2008, p. 356.
- ↑ Jochim Varchim, Joachim Radkau: Kraft, Energie und Arbeit. Energy and society. Reinbek near Hamburg 1981, p. 190.
- ^ The rise and crisis of the German nuclear industry. 1945-1975. Replaced Alternatives in Nuclear Technology and the Origin of the Nuclear Controversy. Rowohlt, Reinbek 1983, ISBN 3-499-17756-0 .
- ↑ a b Julia Mareike Neles, Christoph Pistner (ed.): Nuclear energy. A technology for the future? Berlin / Heidelberg 2012, p. 5.
- ↑ Julia Mareike Neles, Christoph Pistner (ed.): Nuclear energy. A technology for the future? Berlin / Heidelberg 2012, p. 5 f.
- ↑ Sheep as sensors. heise.de, November 15, 2010, inserted March 16, 2012
- ↑ a b The first German nuclear power flowed 50 years ago. on: proplanta.de , June 17, 2011, inserted March 16, 2012.
- ↑ Julia Mareike Neles, Christoph Pistner (ed.): Nuclear energy. A technology for the future? Berlin / Heidelberg 2012, p. 6.
- ↑ Subsidies for nuclear energy and hard coal and lignite. ( Memento from October 12, 2013 in the Internet Archive ) (PDF; 23 kB) Bundesverband Erneuerbare Energie e. V., accessed on January 13, 2011.
- ^ AG Energiebilanzen: Energy consumption in Germany in 2008. ( Memento from January 4, 2012 in the Internet Archive ) Retrieved on January 14, 2011.
- ↑ EuroSolar, April 2006: The costs of atomic energy ( Memento of May 22, 2014 in the Internet Archive ), inserted March 16, 2012. Accessed May 21, 2014.
- ↑ BMU, June 2013: Responsibilities for repository installation and operation as well as financing regulations. ( Memento from March 2, 2014 in the archive.today web archive ) accessed July 1, 2013.
- ↑ Greenpeace study State subsidies for nuclear energy (PDF; 4.2 MB), 2nd edition. October 12, 2010, accessed June 29, 2014.
- ↑ Greenpeace: Nuclear power - subsidized with 304 billion euros
- ↑ Peter Hennicke , Paul JJ Welfens : Energy transition after Fukushima: German special path or global role model? Munich 2012, 26 f.
- ↑ Manager-Magazin quote: “Financial mathematicians have for the first time calculated how expensive a liability policy for a nuclear power plant would be - 72 billion euros annually. (...) According to a study, a complete insurance of the risks of nuclear power would cause electricity prices to explode. According to calculations by actuaries, the premiums to be paid could increase the price of electricity by more than forty times. "
- ↑ TIME: Radiant parasites
- ↑ Magazine for renewable energies: nuclear provisions
- ↑ Plan of the energy companies: the federal government should finance the demolition of nuclear reactors . In: Spiegel-Online , May 11, 2014. Retrieved May 11, 2014.
- ↑ Energy companies should plan bad bank for nuclear power plants . In: Süddeutsche Zeitung , May 11, 2014. Retrieved May 11, 2014.
- ^ Gabor Steingart , Handelsblatt Morning Briefing, October 9, 2015; see also Germany's Non-Stop Nuclear Disaster, Handelsblatt Global Edition, October 9, 2015 ( Memento of March 5, 2016 in the Internet Archive )
- ↑ www.ccomptes.fr The cost of nuclear energy (January 2012). Summary (PDF, 24 pages); Long version (PDF, 441 pages); Glossary ; Cost of nuclear power in France. Incorrectly calculated . In: taz . February 1, 2012. Retrieved February 4, 2012.
- ↑ Large German industrial power users will pay 35 percent less for their electricity next year than those in France Bloomberg News of March 17, 2014.
- ↑ Agora / Prognos: Climate-friendly power generation: Which option is the cheapest? Electricity generation costs of new wind and solar plants as well as new CCS and nuclear power plants based on the subsidy conditions in Great Britain and Germany . Berlin 2014 ( Memento from October 17, 2014 in the Internet Archive )
- ↑ Hinkley Point C: EU approves billions in aid for British nuclear power plant SPIEGEL ONLINE from October 8, 2014.
- ↑ a b c d Luc Gagnon, Camille Bélanger, Yohji Uchiyama: Life-cycle assessment of electricity generation options: The status of research in 2001. In: Energy Policy. Volume 30, No. 14, 2002, pp. 1267-1278, doi: 10.1016 / S0301-4215 (02) 00088-5
- ↑ Energie-Spiegel No. 15 / November 2005
- ^ Ajay K. Gupta, Charles AS Hall: A Review of the Past and Current State of EROI Data. In: Sustainability. 3, 2011, pp. 1796-1809. doi: 10.3390 / su3101796
- ^ Clara Smith, James Blink, Max Fratoni, Harris Greenberg, William Halsey, AJ Simon, Mark Sutton: Nuclear Energy Return on Energy Investment . Lawrence Livermore National Laboratory January 2013. FCR & D-FCO-2012-000275 LLNL-TR-577013
- ^ MAJ Dale: Global energy modeling - A biophysical approach (GEMBA) . PhD thesis. University of Canterbury, 2010.
- ↑ D. Weißbach et al .: Energy intensities, EROIs (energy returned on invested), and energy payback times of electricity generating power plants. In: Energy. Volume 52, 2013, pp. 210 ff, doi: 10.1016 / j.energy.2013.01.029
- ↑ Nuclear Power Plants in Germany: Operating Results 2009 (PDF) Archived from the original on October 5, 2013 ; Retrieved October 27, 2014 .
- ↑ a b c Summary of the different balances of SZ, WNA and Ökoinstitut according to Daniel Lübbert: CO 2 balances of different energy sources in comparison . Ed .: Scientific Services of the German Bundestag. WD08, no. 56 , 2007 ( PDF - Info letter WD 8 - 56/2007).
- ↑ Life cycle analysis: external costs and greenhouse gases
- ↑ CO2 emissions from power generation - a holistic comparison of different technologies. (PDF; 1.7 MB) Trade journal BWK Volume 59, No. 10, 2007, accessed on Jan. 13, 2011.
- ↑ Structure of electricity generation in Germany 2015 Statistics of the Working Group on Energy Balances on the BMWi website
- ↑ EdF website ( Memento of February 14, 2009 in the Internet Archive )
- ↑ a b EdF website ( Memento from February 23, 2009 in the Internet Archive )
- ↑ EdF website ( Memento of February 13, 2009 in the Internet Archive )
- ↑ a b EdF website ( Memento from February 17, 2009 in the Internet Archive )
- ^ Benjamin K. Sovacool, Patrick Schmid, Andy Stirling, Goetz Walter, Gordon MacKerron: Differences in carbon emissions reduction between countries pursuing renewable electricity versus nuclear power . In: Nature Energy . 5, No. 11, October 5, 2020, ISSN 2058-7546 , pp. 928-935. doi : 10.1038 / s41560-020-00696-3 .
- ↑ Nuclear power or renewables? - A large study wants the answer (de) . In: HLK.co.at , November 11, 2020.
- ↑ Renewable energies save more CO2 than atomic energy (de) . In: Solarserver.de , October 7, 2020.
- ^ Harrison Fell, Alex Gilbert, Jesse D. Jenkins, Matto Mildenberger: Reply to “Differences in carbon emissions reduction between countries pursuing renewable electricity versus nuclear power,” by Sovacool et al. (2020) . In: SSRN . December 19, 2020.
- ^ MV Ramana: Second Life or Half-Life? The Contested Future of Nuclear Power and Its Potential Role in a Sustainable Energy Transition . In: Palgrave Macmillan UK (Ed.): The Palgrave Handbook of the International Political Economy of Energy . April, pp. 363-396. doi : 10.1057 / 978-1-137-55631-8_15 . “At the same time, a number of factors, including mounting costs and intense competition from other sources of electricity generation such as natural gas and renewable technologies, have propelled a decline in the share of nuclear energy in the world's power production. [...] To the extent that nuclear power grows in these countries, it will likely be at the expense of renewable energy. In both kinds of countries, however, local communities contest the expansion of nuclear power, fiercely in some cases, and this factor, in addition to the high economic costs associated with nuclear reactors, acts as a brake on accelerated nuclear construction. "
- ↑ Jochen Markard, Nuno Bento, Noah Kittner, Alejandro Nuñez-Jimenez: Destined for decline? Examining nuclear energy from a technological innovation systems perspective . In: Energy Research & Social Science . 67, April, ISSN 2214-6296 , p. 101512. doi : 10.1016 / j.erss.2020.101512 . "Also, increasingly fierce competition from natural gas, solar PV, wind, and energy-storage technologies speaks against nuclear in the electricity sector."
- ↑ Hisham Khatib, Carmine Difiglio: Economics of nuclear and renewables . In: Energy Policy . 96, April, ISSN 0301-4215 , pp. 740-750. doi : 10.1016 / j.enpol.2016.04.013 . "Wider introduction of smart grids and the likely demise of nuclear in some OECD countries are bound to enhance the future prospects for new renewables."
- ↑ German Risk Study Nuclear Power Plants: An investigation into the risk caused by accidents in nuclear power plants, TÜV Rheinland, p. 208 [2]
- ↑ Fred A. Mettler: Chernobyl's Living Legacy. (PDF) iaea, accessed on October 26, 2016 (English).
- ↑ Chernobyl Victims: The Big Numbers Lie. In: Spiegel Online. April 6, 2006, accessed on January 8, 2019 (A new study by German doctors and radiation protection experts calls the Chernobyl casualty figures published by the International Atomic Energy Agency into question as "absurdly" low. There were "serious inconsistencies" in the assessment of the accident, so the critics.).
- ↑ M. Taubiana et. al, "The Linear No-Threshold Relationship Is Inconsistent with Radiation Biologic and Experimental Data", published in the Journal Radiology , 251, 1 (2009)
- ↑ E. Cardis et al, "Estimates of the cancer burden in Europe from radioactive fallout from the Chernobyl accident", International Journal of Cancer (2006), quote 'Indeed, results of analyzes of time trends in cancer incidence and mortality in Europe do not, at present, indicate any increase in cancer rates - other than of thyroid cancer in the most contaminated regions - that can be clearly attributed to radiation from the Chernobyl accident. '
- ↑ a b Estimation of the damage caused by a so-called “super-GAU”. ( Memento of April 24, 2009 in the Internet Archive ) Study by Prognos AG Basel from 1992. 1 trillion = 1,000 billion
- ↑ J. Lelieveld, D. Kunkel, MG Lawrence: Global risk of radioactive fallout after major nuclear reactor accidients. (PDF; 10.7 MB) In: MPI Institute for Chemistry. May 12, 2012. Retrieved September 19, 2012 .
- ↑ The nuclear disaster is more likely than expected. In: MPI for Chemistry. May 22, 2012. Retrieved May 23, 2012 .
- ↑ World Nuclear Association , List of Types of Nuclear Power Plants Under Construction [3]
- ↑ Presentation of the Westinghouse AP 1000 reactor on the Westinghouse manufacturer's website [4]
- ↑ "Passive safety in VVERs", NEI magazine (2011) [5]
- ^ "Toward Improving Patient Safety Through Voluntary Peer-to-Peer Assessment", American Journal of Medical Quality 27, pp. 201-209 (2011) []
- ↑ World Association of Nuclear Operators, Performance Indicators 2015, page 2 [6]
- ↑ Julia Mareike Neles, Christoph Pistner (ed.): Nuclear energy. A technology for the future? Berlin / Heidelberg 2012, p. 152.
- ↑ Julia Mareike Neles, Christoph Pistner (ed.): Nuclear energy. A technology for the future? Berlin / Heidelberg 2012, p. 141.
- ↑ Left in the dust. Greenpeace report. April 2010 (PDF; 4.4 MB). Retrieved February 17, 2011.
- ↑ 3sat nano: Uranium mining poses cancer risk (February 8, 2008)
- ^ Cancer kills fourteen aboriginal uranium workers
- ↑ Uranium mine blamed for high Aboriginal cancer rate
- ↑ uranium trade. An unsafe business with no regard for people or the environment. (PDF; 2.8 MB) greenpeace.org, archived from the original on April 28, 2011 ; Retrieved April 22, 2013 .
- ↑ Background information on the KiKK study. ( Memento from January 11, 2012 in the Internet Archive ) on: bfs.de
- ↑ a b J. Michaelis: Childhood cancer in the vicinity of West German nuclear facilities. In: Deutsches Ärzteblatt. 89/1992, pp. C-1386-90.
- ↑ German Childhood Cancer Register ( Memento from 23 August 1999 in the Internet Archive )
- ↑ a b Epidemiological study on childhood cancer in the vicinity of nuclear power plants. on behalf of the Federal Office for Radiation Protection 2007. (PDF, 7.4 MB)
- ↑ Federal Office for Radiation Protection in Twilight. In: Welt Online. December 12, 2007.
- ↑ bfs.de: Statement by the external expert committee of the BfS on the KiKK study. ( Memento of May 7, 2013 in the Internet Archive ) December 10, 2007.
- ↑ Ben D. Spycher et al .: Childhood cancer and nuclear power plants in Switzerland: a census-based cohort study . In: International Journal of Epidemiology . July 12, 2011, doi : 10.1093 / ije / dyr115 ( oxfordjournals.org [accessed April 22, 2013]).
- ↑ LJ Kinlen et al.: Childhood leukaemia and non-Hodgkin's lymphoma near large rural construction sites, with a comparison with Sellafield nuclear site. In: BMJ. 310/1995, pp. 763-767.
- ↑ Eberhard Greiser: Leukemia diseases in children and adolescents in the vicinity of nuclear power plants in five countries meta-analysis and analysis. (Greiser study 2009) (PDF; 258 kB) This study builds on the KiKK study and extends the database to five countries and 80 nuclear power plants
- ↑ P. Kaatsch, C. Spix, I. Jung, M. Blattner: Leukemia in children under 5 years of age in the vicinity of German nuclear power plants. In: Dt. Ärzteblatt. 105, No. 42, 2009, pp. 725-732. data.aerzteblatt.org (PDF; 133 kB).
- ↑ And the mountain of waste is constantly growing. on: taz.de , October 13, 2009 - "The journalist Éric Guéret traced the radiant residues of nuclear power and found a story of lies, deceptions and trivializations" (reference to a TV documentary on arte )
- ↑ Julia Mareike Neles, Christoph Pistner (ed.): Nuclear energy. A technology for the future? Berlin / Heidelberg 2012, p. 181.
- ↑ Julia Mareike Neles, Christoph Pistner (ed.): Nuclear energy. A technology for the future? Berlin / Heidelberg 2012, pp. 181-183.
- ↑ Search for a repository in Germany. ( Memento from June 3, 2013 in the Internet Archive )
- ↑ IEER: Leukemia Clusters Near La Hague and Sellafield. (PDF; 5.6 MB; English)
- ^ R. Wakefield: Childhood leukaemia and nuclear installations: the long and winding road , in: British Journal of Cancer, volume 111, pp. 1681–1683 (2014)
- ↑ Kelly Martin, "Is Nuclear Waste Disposal A Threat To Our Oceans?" Forbes Magazine, (2018) [7]
- ↑ Frank Wicks, "The Nuclear Waste Problem And Reconsideration Of The Ocean Disposal Option", IECEC 2002 Conference Paper No.20116 [8]
- ↑ Anil Markandya, Paul Wilkinson: Electricity generation and health. ( Memento of January 23, 2014 in the Internet Archive ) In: Lancet. Volume 370, 2007, pp. 979, 981-982. (PDF; 492 kB)
- ↑ Pushker A. Kharecha, James E. Hansen: Prevented Mortality and Greenhouse Gas Emissions from Historical and Projected Nuclear Power. In: Environmental Science & Technology. 47, 2013, pp. 4889-4895, doi: 10.1021 / es3051197 .
- ↑ a b Experts warn of new terrorist threats from nuclear comeback. on: Spiegel online. July 11, 2008.
- ↑ Egbert Kankeleit, Christian Kuppers, Ulrich Imkeller: weapons fitness of reactor plutonium. ( Memento from October 4, 2013 in the Internet Archive )
- ^ A b Steven E. Miller & Scott D. Sagan: Nuclear power without nuclear proliferation? In: Dædalus. 2009, accessed September 24, 2013 .
- ^ Benjamin K. Sovacool: Contesting the Future of Nuclear Power: A Critical Global Assessment of Atomic Energy. In: World Scientific . 2011, p. 190.
- ^ The Bulletin of atomic scientists support the megatons to megawatts program. Archived from the original on July 8, 2011 ; Retrieved September 15, 2012 .
- ↑ All Things Considered: Future Unclear For 'Megatons To Megawatts' Program. Npr.org, December 5, 2009, accessed June 22, 2013 .
- ↑ Nuclear powere in the World Today. World-nuclear.org, accessed June 22, 2013 .
- ↑ Mark Diesendorf : Book review: Contesting the future of nuclear power. (PDF; 81 kB) In: Energy Policy. 2013, accessed September 24, 2013 .
- ^ Proliferation Resistance & Physical Protection. ( Memento of August 2, 2011 in the Internet Archive ) at: gen-4.org
- ↑ Julia Mareike Neles, Christoph Pistner (ed.): Nuclear energy. A technology for the future? Berlin / Heidelberg 2012, p. 114 f.
- ↑ a b Julia Mareike Neles, Christoph Pistner (ed.): Nuclear energy. A technology for the future? Berlin / Heidelberg 2012, p. 115.
- ↑ Manfred Grathwohl: Energy supply. Berlin / New York 1983, p. 429.
- ↑ Terrorist attack on Biblis nuclear power plant would threaten Berlin. In: Spiegel online. November 26, 2007.
- ↑ Biblis not protected against plane crashes. In: Spiegel online. January 11, 2007.
- ↑ Tihange employees blocked, terrorists spy on scientists. In: Aachener Zeitung. March 24, 2016.
- ↑ Wolf-Georg Schärf: European atomic law. Nuclear Energy Law. Berlin / Boston 2012, p. 1.
- ↑ Ferguson, CD, Kazi, T. and Perera J. Commercial Radioactive Sources: Surveying the Security Risks , Monterey Institute of International Studies, Center for Nonproliferation Studies, Occasional Paper # 11, (2003) [9]
- ^ Union-Tribune Editorial Board: The nuclear controversy. In: Union-Tribune. March 27, 2011, accessed July 13, 2014 .
- ↑ James J. MacKenzie: Review of The Nuclear Power Controversy by Arthur W. Murphy. In: The Quarterly Review of Biology. Vol. 52, No. 4, December 1977, pp. 467-468 JSTOR 2823429 .
- ↑ In February 2010 the nuclear debate took place on the pages of The New York Times , ( A Reasonable Bet on Nuclear Power und Revisiting Nuclear Power: A Debate and A Comeback for Nuclear Power? )
- ^ Herbert P. Kitschelt: Political Opportunity and Political Protest: Anti-Nuclear Movements in Four Democracies. In: British Journal of Political Science. Volume 16, No. 1, 1986, p. 57.
- ↑ Jim Falk : Global Fission: The Battle Over Nuclear Power. Oxford University Press, 1982.
- ↑ US Energy Legislation May Be 'Renaissance' for Nuclear Power.
- ↑ Thom Patterson: Climate change warriors: It's time to go nuclear. In: CNN. November 3, 2013, accessed June 13, 2016 .
- ^ Bernard Cohen: The Nuclear Energy Option. Retrieved December 9, 2009 .
- ^ Nuclear Power. Nc Warn, accessed June 22, 2013 .
- ^ Sue Sturgis: Investigation: Revelations about Three Mile Island disaster raise doubts over nuclear plant safety. Southernstudies.org, archived from the original on April 18, 2010 ; Retrieved August 24, 2010 .
- ↑ Greenpeace International and European Renewable Energy Council : Energy Revolution: A Sustainable World Energy Outlook. ( Memento of August 6, 2009 in the Internet Archive ) January 2007, p. 7.
- ^ Marco Giugni: Social Protest and Policy Change: Ecology, Antinuclear, and Peace Movements. 2004.
- ^ Benjamin K. Sovacool: The costs of failure: A preliminary assessment of major energy accidents, 1907-2007. In: Energy Policy . 36, 2008, pp. 1802-1820.
- ↑ Stephanie Cooke: In Mortal Hands: A Cautionary History of the Nuclear Age. Black Inc., 2009, ISBN 978-1-59691-617-3 , p. 280.
- ↑ Kurt Kleiner: Nuclear energy: assessing the emissions ( Memento from June 4, 2011 in the Internet Archive ) Nature Reports. Volume 2, October 2008, pp. 130-131.
- ^ Mark Diesendorf: Greenhouse Solutions with Sustainable Energy . University of New South Wales Press, 2007, p. 252.
- ↑ Mark Diesendorf: Is nuclear energy a possible solution to global warming? July 10, 2007.
- ↑ Guaranteed profit. In: Der Spiegel. November 10, 2008, accessed October 11, 2013.
- ↑ Matthias Brake: Operators earn a lot. on: Telepolis . May 12, 2010, accessed October 11, 2013.
- ↑ Large majority of the population in favor of continuing the nuclear phase-out. In: Forsa survey. Federal Ministry for the Environment, Nature Conservation and Nuclear Safety (BMU), August 18, 2006, archived from the original on June 28, 2011 ; Retrieved April 3, 2014 .
- ↑ Forsa survey for Bild am Sonntag , January 2007
- ↑ TNS Emnid survey for N24, July 2008
- ↑ deutschlandfunk.de , Andruck - Das Magazin für Politische Literatur , July 24, 2017, Dagmar Röhrlich : The Insurers' Dilemma