Climate change
Climate change , including climate change , climate change or climate change , is a change in the climate that occurs worldwide on earth or earth-like planets / moons that have an atmosphere . The cooling or warming associated with climate change can take place over different periods of time.
The current global warming , mainly caused by humans ( anthropogenic ) (see there), is an example of a very rapid, but not yet completed, climate change. For this purpose, in the public debate also the term climate change synonymously used (but then as " The climate change"), lately more often the term " climate crisis ".
A climate change on a global scale is mainly due to a change in the radiative forcing , which the Erdklimasystem of a stable thermal-radiative equilibrium transferred to a new equilibrium. The radiative forcing results from the atmospheric concentrations of greenhouse gases such as carbon dioxide (CO 2 ), methane (CH 4 ) and water vapor (H 2 O), from the varying solar radiation due to the Milanković cycles as well as from the reflectivity ( albedo ) of the earth's surface including the Oceans. The climatic condition during the last millions of years was that of an ice age and was mainly controlled by the Milanković cycles, which significantly changed the solar radiation over tens of thousands of years and thus gave the impetus for the alternation of cold and warm periods. Taking into account the above-mentioned factors, eleven of these warm periods (including interglacial or interglacial periods ) could be identified and described in detail during the last 800,000 years.
A special form of climate change is abrupt climate change . They were in the Earth by eruptions of super volcanoes , large Magmaausflüsse , greatly increased greenhouse gas emissions, rapid changes in ocean currents or through short-term feedback processes triggered in the climate system, often in conjunction with biological crises or mass extinction . Abrupt climate changes can occur regionally (like the Dansgaard-Oeschger events in the North Atlantic area during the last glacial period) or have global effects, for example as a result of a major impact event .
The term climatic variability is occasionally used for climatic changes that last only a few decades or are of a cyclical nature with a variable period and only rarely have a global impact. Cyclical fluctuations are also calledCalled climatic fluctuations , relatively rapid cyclical changes also called climatic oscillation . An epoch of comparatively cool climates is sometimes used in this contextCalled the climatic peak , a relatively warm phase Climate optimum or heat optimum . Optimum and pessimum are a convention in climate systematics and not a valuation, but can lead to misinterpretations and are therefore replaced by the term climate anomaly in more recent specialist literature . During the early Holocene in parts of the northern hemisphere occurring Misox-fluctuation (international 8.2 kilo year event ) was a climate anomaly time sharply defined, but relatively distinct.
Paleoclimatic overview
Evolution of the earth's atmosphere
The earth was formed from several protoplanets of different sizes 4.57 billion years ago . According to the collision theory , it is said to have obtained its current mass from a side collision with a Mars-sized celestial body called Theia 4.52 billion years ago. As a result, parts of the earth's mantle and numerous pieces of debris were hurled by Theia into the then still very low orbit , from which the initially glowing moon was formed within 10,000 years . Due to a lack of valid data, no reliable statements can be made about this earliest and chaotic stage in the history of the earth. Only from 4.0 to 3.8 billion years ago, after the formation of the oceans and the first forms of life, did fossil traces and proxies (“climate indicators”) allow conclusions to be drawn about the environmental conditions. On the basis of this evidence, it is assumed that over large parts of the Archean, despite the significantly reduced radiation output of the sun at that time, a warm or at least mildly temperate climate prevailed.

When it was formed , the earth probably had a primordial atmosphere with the main components hydrogen and helium . This gas mixture only existed for a relatively short time, as light elements quickly evaporated due to the thermal effects of a possible series of impacts as well as the influence of the solar wind and the solar magnetic field . The earth's first atmosphere was formed more than four billion years ago and was essentially the result of extremely strong volcanism with intensive outgassing of carbon dioxide , nitrogen and sulfur dioxide . Since precipitation evaporated immediately on the heated surface of the earth, water vapor dominated the very dense and hot atmosphere with a share of around 80 percent. This was followed by carbon dioxide and hydrogen sulfide in proportions of around 10 and 6 percent, respectively.
Towards the end of the Hadaic era , around 4 billion years ago, the first oceanic basins formed. With the spread of life in the course of the Eoarchean , unicellular organisms such as the archaea first had a direct influence on the atmospheric composition by gradually increasing the methane content with their metabolic products. At the same time, carbon dioxide was withdrawn from the atmosphere and dissolved in seawater, which resulted in precipitation and extensive deposition of carbonates . The inert nitrogen was not involved in these processes, so its concentration increased steadily until it became its main component 3.4 billion years ago when the development of the second atmosphere came to an end.
The formation of the third atmosphere was closely linked to the appearance of free oxygen . It is very likely that cyanobacteria that used oxygen-phototrophic photosynthesis already existed more than three billion years ago . The oxygen released was consumed in the oxidation of various iron compounds and sulfides dissolved in the water . At the end of this long-lasting oxidation process, larger amounts of oxygen diffused into the atmosphere for the first time. There, 2.4 billion years ago, their oxidative effects caused the methane concentration to collapse. This turning point, known as the Great Oxygen Catastrophe, led to the mass extinction of almost all anaerobic life forms in the oceans and then to serious climate change. It is very likely that the 300 million year Paleoproterozoic glaciation (also known as the Huronian Ice Age ) was the direct consequence of methane shortages and an increase in oxygen.
In the late Proterozoic , about 717 and 635 million years ago with the Sturtic Ice Age and the Marino Ice Age , further extended glacial phases occurred. It is believed that a series of snowball-earth events occurred during these glacial periods , with almost complete glaciation of the land masses and oceans over a period of several million years each. This change from longer warm to shorter cold periods continued in the further course of the earth's and climatic history up to the geological present.
Depending on the influences of the earth system, the atmosphere was repeatedly subject to strong changes. The proportions of oxygen, carbon dioxide and methane fluctuated considerably in some cases and played a decisive role, either directly or indirectly, in a number of climate change events. Biological crises have correlated several times in the last 540 million years with a cooling phase (with a global temperature drop of 4 to 5 ° C), but more often with strong warming in the range of 5 to 10 ° C. In the latter case, a bundle of side effects (decline in vegetation, outgassing of toxins and pollutants, oxygen deficits, acidification of the oceans, etc.) contributed to further destabilizing the terrestrial biosphere.

Climate change events in the Phanerozoic
Paleozoic

541 million years ago, the Phanerozoic began the youngest aeon in earth's history. At the same time, this point in time marks the beginning of the Paleozoic Era with the geological system of the Cambrian . During the Cambrian Explosion , the representatives of all animal phyla that exist today were created within just 5 to 10 million years . From a climatic point of view, the Cambrian was a period with partly extremely increased volcanism, with global temperature values of around 20 ° C or partly above and an atmospheric CO 2 concentration of over 5,000 ppm (with a simultaneous reduction in the solar radiation output of around 5 percent). These environmental conditions influenced the chemical composition of the oceans, so that the marine biotopes often reached their limits due to sulfur dioxide and carbon dioxide input, oxygen shortage ( hypoxia ) and the bacterial production and release of hydrogen sulfide . This resulted in significant disruptions to the carbon cycle , combined with several biological crises and mass extinctions .
The warm climate prevailing in the Cambrian continued in the subsequent Ordovician (485.4 to 443.4 mya). However, a gradual cooling process began around 460 million years ago, leading to the Ordovician Ice Age . This development was primarily related to the spread of vegetation on the mainland, which probably took place in the form of moss-like plants and earlier mushroom forms as early as the Middle Cambrian and continued increasingly in the Ordovician. The thickening of the plant cover developed into an elementary climate factor, as it contributed considerably to the accelerated chemical weathering of the earth's surface and thus to a significant reduction in the CO 2 concentration. During the last Ordovician stage of the brain antium (445.2 to 443.4 mya) there was an intensification of the cold-age conditions with a rapid expansion of sea ice surfaces and continental ice sheets , with the surface temperature of equatorial oceans decreasing by 8 ° C and the global average temperatures to about 11 to 13 ° C. At the same time, one of the most momentous mass extinctions in the history of the earth happened , with an estimated loss of species of oceanic life forms of up to 85 percent, possibly partly caused by longer lasting oceanic anoxic events and heavy metal pollution of the seas.
The Silurian (443.4 to 419.2 mya) was characterized by profound plate tectonic processes and several waves of extinction, but the climatic condition remained largely stable after the Ordovician Ice Age had subsided . This changed fundamentally in the Upper Devonian 372 and 359 million years ago with the Kellwasser and Hangenberg events . Both times of crisis lasted a few 100,000 years, recorded the collapse of several ecosystems and showed a rapid alternation of cold and warm phases, with sea level fluctuations in the range of 100 meters. Various factors are considered as possible causes of the mass extinctions in the specialist literature, including the effects of megavolcanism , profound geochemical changes in the oceans with increased release of highly toxic hydrogen sulfide or a significantly increased influence of the Milanković cycles associated with the decreasing carbon dioxide concentration a sudden overturning of the entire climate system. Due to the massive deposition of organic carbon in black shale sediments, the CO 2 content was reduced by around 50 percent and was around 1,000 ppm at the end of the Devonian. The tendency of a progressive decrease in the CO 2 concentration persisted over the entire “hard coal age” of the Carboniferous (358.9 to 298.9 mya) and could lead to an atmospheric proportion of around 100 ppm at the beginning of the Permian (298.9–252 , 2 mya). The increasing spread of deep-rooted and soil-splitting plants in connection with increased soil erosion and extensive coalification processes , which contributed significantly to the formation of the 80 to 100 million year-old Permocarbon Ice Age , had a major influence on this development .
At the Permian-Triassic border , together with the greatest mass extinction of the Phanerozoic, rapid and extremely strong warming occurred, in the course of which, coupled with numerous side effects, the temperatures of the mainland areas and the upper sea layers increased by 8 to 10 ° C. The trigger and main cause of the worldwide collapse of ecosystems are considered to be the outgassing of the Siberian trap , which covered an area of 7 million km² with flood basalts in its phases of activity . At the height of the global crisis, the duration of which is estimated in the more recent specialist literature at a maximum of 30,000 years, the greenhouse gas concentration with significant methane shares reached a very high CO 2 equivalent value , while the oxygen content in the opposite way of 30 percent at the beginning of the Perms dropped to 10 to 15 percent. As a result, it sometimes took more than 10 million years for the biotopes, which had been damaged by extreme warming, major fires, acid rain and oxygen reduction, to gradually regenerate.
Mesozoic
With the Triassic period (252.2 to 201.3 mya) began the Mesozoic Era ( Mesozoic Era), which was mainly characterized by a warm climate , whereby the global average temperatures were initially 2 to 3 ° C above the values of the previous 21st century after initial violent fluctuations. At the Triassic-Jura border, the creation of the 11 million km² Central Atlantic Magmatic Province due to extensive CO 2 emissions caused a warming peak in the range of +4 to +6 ° C and, together with global pollution, another mass extinction. Several cooling phases have been documented for the changeable climatic history of the Jura (201.3 to 145 mya), which according to some studies could have led to the formation of continental ice sheets . Other publications postulate a rather moderate drop in temperature and assess the existence of larger ice caps as unlikely. A comprehensive analysis of the concise and very rapid sea level fluctuations in the Jura comes to the conclusion that the changes in the sea water volume remain puzzling without the assumption of glacial eustasia .
In contrast to this, several icing processes could definitely be proven for the chalk (145 to 66 mya). A broad geological investigation of South Australian regions revealed clear indications, among other things in the form of tillites , dropstones and diamictites , that more or less pronounced glaciers took place on the continent in the course of the Lower Cretaceous. After changing climatic conditions at the beginning of the epoch, what was probably the most intense greenhouse phase of the Phanerozoic came into being in the optimum climate of the Middle and Upper Cretaceous , with a strongly fluctuating CO 2 level at an approximate average of 1,000 ppm and possibly partly caused by long-lasting superplume activities or respectively a greatly increased plate convergence .
A special feature of the Cretaceous was the accumulation of oceanic anoxic events , with that at the Cenomanium - Turonium boundary (93.9 mya) reaching global dimensions and which probably caused the most striking disruption of the carbon cycle of the last 100 million years, with significant climatic and biological factors Effects. Towards the end of the Cretaceous a gradual cooling set in over millions of years, in the Maastrichtian (72.0 to 66.0 mya) with several brief climatic changes and a decrease in the carbon dioxide concentration to approx. 420 to 650 ppm.
Cenozoic

system | series | step | ≈ age ( mya ) |
---|---|---|---|
quaternary | Holocene | Meghalayum | 0 ⬍ 0.012 |
Northgrippium | |||
Greenlandium | |||
Pleistocene |
Young Pleistocene (Tarantium) |
0.012 ⬍ 0.126 |
|
Middle Pleistocene (Ionian) |
0.126 ⬍ 0.781 |
||
Calabrium | 0.781 ⬍ 1.806 |
||
Gelasium | 1,806 ⬍ 2,588 |
||
deeper | deeper | deeper | older |
The asteroid impact on the Cretaceous-Paleogene border 66 million years ago, which extinguished about 75 percent of the species at that time, possibly combined with a global permafrost climate over several years, marks the transition from the Mesozoic to the Modern Age ( Cenozoic ). After the stabilization of the earth's climate system and the relatively rapid regeneration of the biosphere , a warm-temperate climate prevailed at the beginning of the Paleocene (the first series of the Cenozoic), which, however, became increasingly subtropical in the further course. Some studies cite lower CO 2 values of 300 to 450 ppm for the early and middle Paleocene than in the late Cretaceous, while other studies calculated a mean value of 600 ppm with a correspondingly higher global temperature on the basis of multiproxy evaluations.
On the border with the Eocene (56 mya), the Paleocene / Eocene Temperature Maximum (PETM) was the first and most pronounced of several thermal anomalies. Massive emissions from volcanic or oceanic sources quickly released several thousand gigatons of carbon dioxide and methane into the atmosphere, and the global temperature rose from around 18 ° C in the late Paleocene at the height of the 200,000 year anomaly to at least 24 ° C. Several studies favor the CO 2 emissions of the North Atlantic Magmatic Greater Province , which arose during the formation of the North Atlantic, as the primary cause of the abrupt warming at the beginning of the PETM . However, this assumption is not undisputed and competes with other explanatory approaches. What is certain is that the expansion of the tropical climatic zone to higher latitudes led to extensive migration of flora and fauna and had diverse biological effects.
The Eocene climatic optimum came to an end with the Azolla event around 49 million years ago, which resulted in a significant reduction in CO 2 . At about the same time, the main phase of the collision of the Indian continental plate with the Eurasian plate, which was initially accompanied by violent flood basalt volcanism, ended . In the course of the unfolding of the Himalayas into high mountains, erosion and weathering processes and the associated carbon sequestration became a climatic factor that further intensified the gradual cooling process. A sharp climatic change occurred at the Eocene- Oligocene border (33.9 mya) with the beginning of the Cenozoic Ice Age . Within a very short period of time, possibly only a few millennia, there was a rapid drop in atmospheric CO 2 concentration with global cooling including the oceans and the beginning of the formation of the Antarctic ice sheet .
The Quaternary Cold Age , the youngest part of the Cenozoic Ice Age , began around 2.7 million years ago with extensive glaciations in the northern hemisphere. In science, the prevailing view is that the increasing arctic glaciation is associated with a significant decrease in the global CO 2 concentration, which means that the summer months in particular are cooler. Some studies state a first cooling phase in the end of the Pliocene (3.2 mya) and a second in the early Pleistocene (2.4 mya), with the CO 2 content falling from originally 375 to 425 ppm to 275 to 300 ppm, with another Decrease during the subsequent cold-time cycles. For probably the first time during the Phanerozoic , the polar near mainland areas of both hemispheres were covered by ice sheets.
The last 11,700 years of the Quaternary form the interglacial ( interglacial ) of the Holocene and thus the geological present. This period includes all known advanced civilizations as well as the entire historically documented human history including modern civilization. Until recently, during the Holocene there was a stable global climate according to geological standards with a temperature corridor of approximately ± 0.6 ° C. The absence of geophysical, biological and climatic crises is seen as a guarantee that apart from regionally limited cuts, a relatively uniform cultural and technological development of human societies could take place.
Causes of natural climate changes in the earth system
In the history of the earth, climate changes were often based on a combination of several factors. Most of these climatic factors are now scientifically precisely understood and in some cases proven by measurements, others are generally recognized as a fundamental causal relationship , and some are obvious due to the good correlation of the assumed influencing variables with certain climatic developments, but not yet finally clarified in detail. A general distinction is made between positive and negative feedbacks, positive feedbacks being referred to as self-reinforcing feedbacks (such as ice-albedo feedbacks or water vapor feedbacks ) and negative feedbacks being referred to as self-weakening or stabilizing feedbacks. A negative feedback system will thus compensate for disturbances in its energetic balance and return to the original climatic condition.
Even during a period with little geological events, the climate was never really stable and, even apart from major environmental crises, it was subject to significant fluctuations over periods of tens of thousands or 100,000 years. The main reasons for this are changes in vegetation cover with repercussions on the albedo and carbon cycle, in addition to this, longer-lasting volcanic activities with the corresponding release of CO 2 , aerosols and sulfur dioxide or regionally occurring plate tectonic processes such as the opening or closing of ocean roads, each connected with a shift, intensification or weakening of atmospheric and oceanic circulation patterns.
Some hypotheses take the view that the course of the climate on the scale of the earth's history is not only controlled by terrestrial factors, but also by varying cosmic radiation influences . According to this assumption, the glacial periods of the Phanerozoic should correlate with regular spiral arm passages of the sun and its heliosphere . Periodically occurring cosmic influences on biological and climatic development are, however, only poorly documented according to the current state of research and at best play a subordinate role.
Sun
Of the factors that have shaped the earth's climate from the beginning and continue to determine it today, the external influence of the sun on the earth's climate system plays the most important role. The solar energy generated and radiated in a thermonuclear fusion process is the basis for the origin and development of life on earth. The radiation intensity averaged over many years in the form of the solar constant is currently 1367 W / m². Due to the eccentricity of the earth's orbit, its strength varies between 1325 W / m² and 1420 W / m² over the course of a year. However, the amount of solar radiation on the earth's surface is much lower than outside the atmosphere. The irradiation of the summer midday sun in Central Europe amounts to around 700 W / m² when the sky is clear, in contrast to just under 250 W / m² in winter.
The term solar constant is somewhat misleading, as it is subject to cyclical fluctuations - albeit within narrow limits - (around 0.1 percent both in the visible range and in the total radiation) and is causally related to the maximum and minimum periods of the sunspots and thus to the different periods of activity coupled to the sun.
These fluctuations are based on more or less regular changes in the solar magnetic field and are accompanied by a visible fluctuation of the sunspots. The two main cycles are the Schwabe cycle (11 years) and the Hale cycle (22 years). In addition to the Gleißberg cycle (85 ± 15 years), a number of longer-term cycles were postulated. They are essentially
- the Suess or de Vries cycle (180–210 years),
- a 1470-year cycle that may correlate with the Dansgaard-Oeschger events of the last ice age,
- the Hallstatt or Bray cycle (2400 ± 200 years), possibly the effect of a constellation of the large planets ( gas giants ) in the solar system that takes place every 2318 years .
However, the sun can also experience reduced activity for decades and remain in a “standstill phase”, so to speak. The English astronomer Edward Maunder examined the historically documented number of sunspots in 1890 and found a pause in the 11-year cycles between 1645 and 1720 ( Maunder minimum ), which was roughly in the middle of the so-called " Little Ice Age ". However, cooler climates in historical times (as well as warm periods such as the medieval climate anomaly ) were regionally and temporally unevenly distributed and only occurred globally and only rarely and only for a few decades. Accordingly, the core phase of the Little Ice Age - from the end of the 16th to around the middle of the 19th century - was very likely limited to the northern hemisphere to varying degrees. This relativizes the influence of the sun insofar as, in addition to the fluctuations in solar radiation, factors such as volcanic activity, changes in the atmospheric circulation and the North Atlantic oscillation have to be taken into account.
For earlier epochs, the magnetic activity of the sun can be determined with the help of the cosmogenic radionuclides 14 C and 10 Be formed by cosmic radiation . In principle, the C14 method delivers more precise results when using a calibration curve ( DeVries effect ), but can not be used for longer time scales due to the comparatively short half-life of the 14 C isotope of 5,730 years. In contrast, the half-life of the beryllium isotope 10 Be is 1.51 million years and is therefore suitable for an analysis period of up to 10 million years. The concentration of 10 Be correlates with cosmic radiation and thus indirectly with the strength of the earth's magnetic field and solar activity. In addition, high 10 Be contents - synonymous with low solar activity - also indicate increased aerosol concentrations in the atmosphere.
The changes in the solar constant and solar activity measured with satellites since 1978 are too small to be an explanation for the temperature development of the last decades.All data sets indicate that global temperature development has largely been decoupled from solar activity since the middle of the 20th century Has. According to this, the additional radiative forcing from the sun has been around 0.11 W / m² since the beginning of industrialization, while anthropogenic greenhouse gases are currently contributing around 2.8 W / m² to warming, and the trend is increasing.
The development of the sun as the main sequence star in the Hertzsprung-Russell diagram is of primary importance on the entire time scale of earth and climate history . After a relatively short phase as a protostar , it began to convert energy 4.6 billion years ago through the process of nuclear fusion , in which the supply of hydrogen in the sun's core is gradually converted into helium through the proton-proton reaction . This stage lasts around 11 billion years, during which time the luminosity and the radius of the sun increase constantly. This means that the sun at the beginning of its existence (and at the same time at the beginning of the earth's history) had only 70 percent of the current radiation output and that this radiation increases on average every 150 million years by about 1 percent to the current value and will continue to do so . This so-called paradox of the weak young sun (English Faint Young Sun Paradox ) not only embodies an elementary climate factor over billions of years, but also leads to fundamental questions about the origin and continuity of earthly life, which are currently being discussed on a broad basis in an interdisciplinary manner, especially in the atmospheric sciences .
Earth orbit, precession and axis tilt
The earth's orbit around the sun, the precession of the earth 's axis of rotation and the inclination of the earth's axis and thus the changing angles of incidence of solar radiation on the northern and southern hemisphere are subject to different cycles with a duration of 25,800 to around 100,000 or 405,000 years. They were first examined and calculated by the Serbian astrophysicist and mathematician Milutin Milanković (1879–1958) with regard to geoscientific issues. The fluctuations in the insolation on the earth's surface caused by the Milanković cycles are relatively minor, but act as “impulses” in the climate system and are considered to be the main cause of the alternation between the warm and cold phases within the current Ice Age . For example, the slight increase in temperature induced by the orbital parameters caused an increase in the atmospheric CO 2 concentration, which subsequently led to further warming and a transition from a cold to a warm period, with both increases in many cases almost according to recent studies ran synchronously.
Although the process of gradually changing insolation takes considerable time, it can be proven by measurement over thousands of years. Sediment cores from the deep sea show a Holocene climatic optimum around 8,000 to 6,000 years ago, the temperature of which on a global basis was not reached again until the 21st century. Due to the decrease in solar radiation in northern latitudes during the summer maximum, coupled with the periodicity of the Milanković cycles, there has since been a slight decrease in temperature averaging 0.1 to 0.15 ° C per millennium. This cooling trend would normally result in the interglacial of the Holocene being followed by a new glacial period in 30,000 to 50,000 years. Whether this event will occur as predicted or whether the current warm period will be of a longer duration depends largely on the extent to which anthropogenic and natural greenhouse gases will enter the atmosphere in the future.
The periodic changes in the Earth's orbit parameters can be demonstrated as a stable influencing variable over large parts of the Phanerozoic , even in the predominantly tropical climates of the Cretaceous period . New analyzes have shown that the major cycle of 405,000 years can be traced back to the Upper Triassic around 215 million years ago and classified chronologically. The Milanković cycles are also ascribed a significant influence on the climatic fluctuations occurring during the Permocarbon Ice Age in the late Carboniferous (about 315 to 299 mya) . According to more recent findings, the periodic changes in eccentricity could also influence the carbon cycle within the various earth spheres .
For decades, experts took little notice of Milanković's calculations, which were judged to be speculative. Since the 1980s, however, the theory has been an integral part of paleoclimatology and Quaternary research in a modified and expanded form and is often used as an important geological influencing factor and as an instrument for reconstructing the phases of the cold ages. The following table summarizes the most important key data of the Milanković cycles.
Orbit parameters | Cycle duration | Fluctuation range | Current status |
---|---|---|---|
Precession of the earth's axis of rotation | approx. | 25,800 years360 ° (full circle) within a complete cycle | Development for the more concise formation of the seasons in the northern hemisphere with longer winters |
Angle of inclination of the earth's axis to the ecliptic | approx. | 41,000 yearsbetween 22.1 ° and 24.5 ° | 23.43 ° (tending towards the minimum) |
Eccentricity of the earth's orbit | approx. 100,000 or 405,000 years 1) | from 0.0006 (almost circular) to 0.058 (slightly elliptical) | 0.016 (with a tendency to circular orbit) |
Greenhouse gases
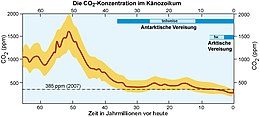
More than 20 greenhouse gases of natural and anthropogenic origin can be detected in the earth's atmosphere, including highly effective greenhouse gases such as nitrous oxide (laughing gas), sulfur hexafluoride and carbonyl sulfide . Although, with regard to significant climate change events in the past, almost exclusively carbon dioxide and methane played a primary role in addition to water vapor , the importance of the other greenhouse gases is definitely relevant, as their overall effect currently has almost the same global warming potential as carbon dioxide.
In contrast to nitrogen, oxygen and all noble gases , greenhouse gases are active in infrared radiation thanks to their molecular structure . For example, CO 2 can absorb solar thermal energy at wavelengths of 4.26 µm and 14.99 µm and re-emit it towards the earth's surface . Due to this greenhouse effect , which was first described by Joseph Fourier in 1824 , the near-surface average temperature in the mathematical-physical model increases by approximately 33 ° C to +14 to +15 ° C. Without the greenhouse effect, the lower atmosphere would only have a global mean of −18 ° C and lead to a complete icing of the planet (whereby the temperature level would probably drop even further due to several interactions).
The most important and, in terms of its influence, the strongest greenhouse gas is water vapor , whose share in the natural greenhouse effect fluctuates between 36 and 70 percent depending on the geographic conditions or climatic zone . Since the atmospheric water vapor content depends directly on the air temperature, its concentration decreases at lower average temperatures and increases during a warming phase ( water vapor feedback ), whereby according to the Clausius-Clapeyron equation the atmosphere can absorb 7 percent more water vapor per degree increase in temperature.
The atmospheric concentration of carbon dioxide is usually given in ppm (= parts per million), that of methane in ppb (= parts per billion). Due to human influences , the content of carbon dioxide has increased to over 400 ppm (previously 280 ppm) and that of methane to almost 1,900 ppb (previously 800 ppb) since the beginning of the industrial age . These are the highest concentrations in at least 800,000 years. There is a high probability that no significantly higher CO 2 values than in the previous 21st century have occurred during the last 14 million years (since the climatic optimum of the Middle Miocene ) . Nevertheless, there were geological epochs with considerably larger CO 2 proportions, such as in the Cambrian around 500 million years ago, when the carbon dioxide concentration was in the range of 5,000 to 6,000 ppm. Conclusions about the present are problematic, however, because the conditions at that time (including the 4 to 5 percent less solar radiation compared to today, the complete lack of land plants and the associated changed organic carbon cycle ) are in no way transferable to the Holocene .
Carbon dioxide and / or methane were not always the main drivers of climate change. In the history of the earth, they sometimes acted as “feedback links” that strengthened, accelerated or weakened developments depending on the geophysical constellation. In this context, in addition to the Earth's orbit parameters , feedback such as ice-albedo feedback , vegetation cover , weathering processes and the variability of the water vapor content in the atmosphere must be taken into account.
Considered over the entire duration of the Phanerozoic , the CO 2 concentration decreased over the course of 540 million years; it swayed greatly. For example, around 300 million years ago during the Permocarbon Ice Age , at the transition from the Carboniferous to the Permian , the CO 2 values averaged 300 ppm and possibly fell to around 100 ppm in the early Permian. 50 million years later, during the super-greenhouse phase at the Permian-Triassic border , however, the CO 2 equivalent reached a level of around 3,000 ppm in a very short geological time due to extensive flood basalt outflows and other feedback processes.
Based on the findings and data of paleoclimatology , it is unanimously assumed in the scientific community that the currently observable climate change will proceed faster in the predicted further course than all known warming phases of the Cenozoic era (i.e. during the last 66 million years). Even during the thermal anomaly of the Paleocene / Eocene Temperature Maximum (PETM), the atmospheric carbon input and the associated temperature increase had significantly lower annual average rates of increase than at present. In contrast to earlier assumptions, the additional anthropogenic CO 2 input will only decrease gradually, even if emissions are largely halted, and will still be detectable to a significant extent in several thousand years. Building on this, some studies postulate a longer warm period in the range of 50,000 to 100,000 years , taking into account the Earth system's climate sensitivity . Various tipping elements in the earth system were identified as additional hazard potentials, which would trigger a number of irreversible processes in the short term if the temperature continued to rise. A simulation published in 2019 suggests that at a CO 2 concentration above 1,200 ppm stratocumulus clouds could disintegrate, which would contribute to the intensification of global warming. Under these conditions, this process could have come into its own both during the strong warming phases in the Eocene and during the climatic optimum of the Upper Cretaceous .
plate tectonics
The plate tectonics as "drive motor" of all large-scale processes in the outer earth sheath ( lithosphere is) in erdgeschichtlichem scale one of the major environmental factors with a plurality of associated processes and impacts. These include the formation of fold mountains ( orogenesis ), the various forms of volcanism ( hotspots or mantle diapirs , magmatic large provinces, etc.), the formation of mid-ocean ridges , the "submerging" of oceanic crust under continental lithospheric plates ( subduction ) and continental drift, each with direct Consequences for the atmospheric concentration of greenhouse gases and thus for the climate of the earth.
According to the geographical definition, there are seven continents on earth, whereby their current position and number are the result of a development that began more than 150 million years ago. During the Paleozoic Era and over parts of the Mesozoic Era , however, large and supercontinents shaped the topographical image of the earth. A land mass that unites almost all continental plates is considered a supercontinent . The geologically youngest supercontinent Pangea , formed by the merger of the two major continents Laurussia and Gondwana , existed from the Upper Carboniferous to the Mesozoic (310 to 150 million years ago). The collision of the continental plates led to a folding of the crustal rocks and the formation of a high mountain range along the plate boundaries. When the conditions stabilized, weathering and erosion processes became a relevant climate factorː They withdrew large amounts of carbon dioxide from the atmosphere and thus tended to contribute to global cooling. Millions of years later, after a phase of tectonic calm, the continental shields broke apart again at their “seams” with a considerable increase in flood basalt volcanism , which led to a renewed increase in the CO 2 concentration.
Large continents and supercontinents are characterized by a pronounced continental climate with an annual temperature amplitude of up to 50 ° C, large arid and desert areas in the interior, and a relatively low biodiversity in the fauna area . At the height of its expansion, Pangea stretched from the northern polar region to Antarctica and had an area of 138 million km², including all shelf seas, of which 73 million km² was accounted for by the southern continental Gondwana. The large continent of Gondwana, which had dominated the southern hemisphere for a long time , was formed around 600 million years ago and encompassed the core areas ( cratons ) of South America , Africa , Antarctica , Australia , Arabia , Madagascar , New Guinea and India . In the course of its geological history, large areas of Gondwana were covered several times by glaciers and ice sheets, first during the Ordovician Ice Age (also known as the Hirnantic Ice Age or Andean-Sahara Ice Age ). It began around 460 million years ago in the Upper Ordovician , reached its climax at the last Ordovician stage of the Hirnantium and ended in the Lower Silurian 430 million years ago.
During the Permocarbon Ice Age ( Karoo Ice Age ) Gondwana again became the center of extensive glaciation. This affected what is now southern Africa and large parts of South America between 359 and 318 million years ago. In a second glacial phase in the Pennsylvania 318 to 299 million years ago, the ice sheets shifted to the cratons of India and Australia, before southern Africa glaciated again during the Dwyka Glacial (up to 280 million years ago). The Permocarbone Ice Age was the second longest ice age in the history of the earth. It comprised a large part of the Carboniferous and ended during the Permian about 265 million years ago. The position of Gondwana around the Antarctic , which has hardly changed over millions of years , contributed significantly to the formation of the two Paleozoic glacial periods, since the polar near mainland ice more quickly and more effectively than open sea zones due to the relatively high albedo and this process gains momentum through the ice-albedo feedback .
Like almost all natural climate change, the event of the Permocarbon Ice Age was based on several factors. In addition to the mainland freezing described above, these were the following mutually reinforcing mechanisms:
- Due to the increasing vegetation cover during the Carboniferous in connection with the spread of deep-rooted plants that split the soil and extensive coalification processes , the atmospheric CO 2 concentration fell to a hitherto unique low. This development contributed significantly to the fact that towards the end of the epoch and in the early Permian there were several pronounced climatic changes, with a fluctuation range of the CO 2 level of 150 to 700 ppm, coupled to the various cold and warm phases .
- Due to the extremely high oxygen content of 33 to 35 percent, the probably most devastating forest and wildfires in the history of the earth occurred in the Upper Carboniferous, with the possible side effect of a global smoke and haze that dampens sunlight. P. 443 f.
- After Laurussia and Gondwana had united to form the supercontinent Pangea and thus to a huge continental barrier, the water and heat exchange of the equatorial ocean currents stalled, and Antarctic cold water flowed north along the coasts of Gondwana. This contributed to intensifying the already prevailing cooling trend again.
Another example of the climatic relevance of plate tectonics is provided by the recent geological history with the formation of the Drake Strait , which is around 480 nautical miles wide today and connects the Atlantic with the Pacific Ocean . Until the late Eocene , Antarctica and South America - as an extensive remainder of the former great continent Gondwana - were connected by a land bridge, before the Drake Strait began to open and deepen. This created in the Southern Ocean the strongest ocean current of the earth, the Antarctic Circumpolar Current , the Antarctica circled from now on in a clockwise direction, the continent from the supply warmer sea water section and the basis for the formation of the Antarctic ice sheet created. Thus, Antarctica was not only geographically isolated, but also thermally. The first significant glaciation at the Eocene- Oligocene border, 33.9 million years ago, was synonymous with the beginning of the Cenozoic Ice Age , and during the Pliocene the ice cover reached the current extent of around 14 million km² for the first time.
Volcanism
Volcanic eruptions of magnitude 5 or 6 on the volcanic explosivity index have the potential to cause aerosol-related global cooling of around -0.3 to -0.5 ° C over a number of years, associated with several feedbacks, as was the case with the eruption of the Pinatubo was detected in 1991. In particular, gases can reach the stratosphere (17 to 50 km altitude). Via three processes, known as gas-to-particle (GPC, gas-to-particle conversion), drop-to-particle (DPC, drop-to-particle conversion) or lump-to-particle conversion (BPC , bulk-to-particle conversion), ejected particles and gases are converted into aerosols . As a result of the high-altitude currents (strong winds), these spread into the stratosphere, where they change the transmitted solar radiation through absorption , scattering and reflection . These processes have a direct influence on the temperature in all air layers.
The effects of a volcanic eruption can vary greatly over time. Depending on the development process, aerosols have typical radii of r <0.1 μm to r> 1 μm. Depending on the radii and the corresponding cleaning mechanisms, aerosols have a residence time ranging from a few minutes to a few years before they combine through leaching (ice, snow or rain), deposition through gravitation or coagulation (coagulation, small particles into a large particle) from the atmosphere. This results in a time-variable net effect on the air temperature. First the large particles absorb solar radiation and thus warm the atmosphere (positive Netteo effect), but then quickly fall out of the air column . After that, the small and medium-sized particles gain in importance because they reflect and scatter solar radiation and thus lower the air temperature (negative net effect). This negative net effect is also called volcanic winter when it is more pronounced .
The eruption of the Laki crater on Iceland in the summer of 1783 probably caused the extremely cold winter of 1783/84 in Northern Europe and North America. In April 1815, the eruption of the Tambora volcano on the island of Sumbawa, which is now part of Indonesia, played a key role in the “ Year Without a Summer ” (1816). Large areas of North America as well as Western and Southern Europe were particularly affected by the cold snap. Currently, the annual volcanic CO 2 emissions are between 180 and 440 megatons . The anthropogenic CO 2 emissions are several orders of magnitude higher and have reached around 36 gigatons each in recent years.
Super volcanoes
Due to their ejection quantity of over 1000 km³ of lava , ash and aerosols ( tephra ), super volcanoes in prehistoric times influenced the climate for decades and triggered an abrupt global cooling . They are classified in the highest category on the volcano explosion index with the value VEI-8. In contrast to most other volcanoes, super volcanoes do not leave volcanic cones behind after an eruption, due to the size of their magma chamber , but huge calderas . The last eruption of a super volcano occurred on the northern main island of New Zealand around 26,500 years ago in the area of what is now Lake Taupo . Another eruption occurred in Sumatra with the Toba explosion 74,000 years ago. There are currently several potential supervolcanoes that could reach VEI-8 if they erupt again. The most famous of them is located under Yellowstone National Park in the US state of Wyoming . The history of this hotspot can be traced back over 17 million years and has seen a number of eruptions during this time, including two super-eruptions in the Younger Miocene (8.99 and 8.72 mya). Since the beginning of the Oligocene (33.9 mya) over 40 such events have been clearly proven worldwide. However, permanent climatic and ecological consequences from super volcanoes have not been proven.
Magmatic Greater Provinces
In geological terms, so-called Magmatic Large Provinces (English Large Igneous Provinces ) were the cause of far-reaching and relatively rapid climate change events. This is the large-volume escape of igneous rocks from the earth's mantle , mainly in the form of flood basalts , which over the course of several hundred thousand years occasionally spread over millions of km². Depending on the extent and duration of the flood basalt release, considerable amounts of carbon dioxide were released into the atmosphere, along with significant amounts of hydrogen chloride , fluorine and sulfur dioxide . In contrast to "normal" volcanism, the activities of a magmatic large province did not cause aerosol-related cooling, but instead led to a worldwide temperature increase, in extreme cases coupled with an additional warming spiral with the help of methane or methane hydrate from oceanic deposits. Most of the mass extinctions in the history of the earth are very likely to be directly related to the large-scale outflow of flood basalts and the subsequent destabilization of terrestrial and marine biotopes.
Well-known magmatic large provinces , which exerted an influence on climate and biodiversity in different ways , are the Siberian Trapp ( Perm-Triassic border , 252 mya), the Dekkan-Trapp in today's West India ( Chalk-Paleogene border , 66 mya) and the North American Columbia plateau basalt ( Middle Miocene , main activity 16.7 to 15.9 mya).
Other climate-affecting factors

Other factors that can have a lasting impact on the climate or have influenced it in the past:
- The albedo as a measure of the reflectivity of non-luminous surfaces, in the earth system dependent on the extent of the oceans, ice sheets, deserts and vegetation zones
- Organisms that have caused climatic effects through the fixation or production of greenhouse gases in the course of the earth's history, such as corals , methane-forming agents , phytoplankton and plants such as the floating fern Azolla
- Changes in atmospheric circulation , monsoons
- Changes in ocean currents : Thermohaline Circulation , North Atlantic Oscillation , Southern Oscillation Index , El Niño ( ENSO )
- Sea level fluctuations (eustasia), caused either by the binding of water in continental ice sheets (or their melting) or by changes in the ocean basin volume as a result of tectonic shifts
- The heat content of the oceans
- The moon through its influence on the tides and thus on the great ocean currents
- The temperature-dependent water vapor content of the atmosphere and cloud formation
- The vegetation cover in its function as a carbon sink
- Weathering processes bind atmospheric CO 2 in the lithosphere over longer periods of time ( carbonate-silicate cycle ) and have different effects depending on the respective environmental conditions such as warm or cold periods
- The positive feedback process of ice albedo feedback
Anthropogenic climate change

In addition to natural factors, humans have influenced the climate to a considerable and increasing extent , especially since the beginning of industrialization : The " Intergovernmental Panel on Climate Change " (IPCC), which monitors the state of science on behalf of the United Nations summarized, came to the conclusion in 2007 that the warming of the atmosphere and the oceans since the beginning of industrialization is mainly due to the release of greenhouse gases by humans, with the increasing carbon dioxide concentration and its measurable influence on the radiation balance being the main factor in the warming process . Current analyzes come to the conclusion that the annual average anthropogenic greenhouse gas emissions in the 21st century to date have exceeded those of the Paleocene / Eocene temperature maximum by about ten times. By the end of the 21st century, the IPCC anticipates a temperature increase in the probable range of 2.6 ° C, depending on various factors such as the further development of emissions in the worst case ( representative concentration path RCP 8.5) , which relies heavily on the use of fossil fuels up to 4.8 ° C (mean value = 3.7 ° C). In the best scenario (RCP 2.6) , which models very ambitious climate protection measures , the probable range is 0.3 ° C to 1.7 ° C (mean value = 1.0 ° C).
In its fifth assessment report published in 2014/2015, the IPCC writes that it is extremely likely that humans caused more than 50 percent of the warming observed between 1951 and 2010. The best guess is that the human impact on warming is roughly consistent with the overall warming observed during this period. An analysis from 2014 puts the probability that the rise in global temperature registered over the past 60 years would have been similarly high without anthropogenic greenhouse gas emissions, at just 0.001%. Several studies agree that, in contrast to pre-industrial climate fluctuations, the current warming process occurs simultaneously on all continents, has not been surpassed in its rapid development by any climate change of the last two thousand years and is probably also without a comparable example in recent geological history. A detailed analysis of palaeoclimatological data series showed that the warming that has taken place in the 21st century to date is very likely to exceed the temperature values of the Holocene climatic optimum (around 8000 to 6000 years ago).
The climate change, which is expected to intensify in the next few decades, has the potential, in addition to serious environmental changes, to trigger global conflicts and large-scale migration movements (“climate” or “ environmental flight ”). A key aspect of the current development is climate protection as an overarching term for those measures that are intended to mitigate the foreseeable consequences of global warming and, if possible, prevent them. The primary task here is the sustainable and rapid reduction of anthropogenic CO 2 emissions.
The Secretary General of the World Meteorological Organization (WMO) Petteri Taalas stated at the end of 2018 that “we are the first generation to fully understand climate change and the last generation to be able to do something about it”.
Scientific journals on climate change
In the following, some internationally renowned specialist journals with an interdisciplinary focus are listed, the impact factor of which is well above the average. The thematic focal points of the individual publications differ, but in total they comprehensively describe all climate change events of the Phanerozoic and the Precambrian , including related subject areas. This begins with the current global warming with a special focus on meteorology , atmospheric sciences and oceanography , extends to the presentation of earlier climatic effects on the biosphere ( paleontology and paleobiology ) to various climate-relevant aspects of geology and geophysics in geological time periods.
- Nature Climate Change ,Editorː Nature Publishing Group (GB), Languageː English, Frequency Monthly, Linkː official website , ISSN 1758-678X
- Nature Geoscience ,Editorː Nature Publishing Group (GB), Languageː English, Frequency Monthly, Linkː official website , ISSN 1752-0894
- Geophysical Research Letters ,Editorː American Geophysical Union (USA), Languageː English, Frequency Erschein fortnightly, Linkː official website , ISSN 0094-8276
- Geology ,Editorː Geological Society of America (USA), Languageː English, Frequency Monthly, Linkː official website , ISSN 0091-7613
- Palaeogeography, Palaeoclimatology, Palaeoecology ("Palaeo3") , Verlagː Elsevier , languageː English, frequency of publicationː 56 issues per year, Linkː official website , ISSN 0031-0182
- Gondwana Research , Verlagː Elsevier, languageː English, frequency of publicationː monthly, Linkː official website , ISSN 1342-937X
- Earth and Planetary Science Letters , Verlagː Elsevier, languageː English, frequency of publicationː 2 issues per month, Linkː official website , ISSN 0012-821X
- Quaternary Science Reviews , Verlagː Elsevier, Languageː English, Frequencyː fortnightly, Linkː official website , ISSN 0277-3791
See also
literature
Reference works (English)
- William F. Ruddimann: Earth's Climate - Past and Future. Palgrave Macmillan, 2001, ISBN 0-7167-3741-8 .
- Raymond T. Pierrehumbert: Principles of Planetary Climate. Cambridge University Press, 2010, ISBN 978-0-521-86556-2 .
- Thomas N. Cronin: Paleoclimates: understanding climate change past and present. Columbia University Press, New York 2010, ISBN 978-0-231-14494-0 .
- Raymond S. Bradley: Paleoclimatology. Reconstructing Climates of the Quaternary. Academic Press (Elsevier Inc.) Oxford, Amsterdam, Waltham, San Diego, Third Edition 2015, ISBN 978-0-12-386913-5 .
German-language literature
- Monika Huch, Günter Warnecke, Klaus Germann (eds.): Climatic testimonies of geological history. Perspectives for the future . With contributions by Wolfgang H. Berger, Arthur Block, Werner von Bloh, Werner Buggisch, Klaus Germann, Monika Huch, Gerhard Petschel-Held, Hans-Joachim Schellnhuber, Torsten Schwarz, Hansjörg Streif, Otto H. Wallner, Günter Warnecke, Gerold Wefer . Springer, Berlin / Heidelberg 2001, ISBN 3-540-67421-7 .
- Wolf Dieter Blümel: 20,000 years of climate change and cultural history - from the Ice Age to the present . In: Interactions, yearbook from teaching and research of the University of Stuttgart . University of Stuttgart, 2002, ISSN 0724-3324 , p. 3–19 ( digitized version [PDF; 1.8 MB ]).
- József Pálfy: Disasters in the history of the earth. Global extinction? Schweizerbart, Stuttgart 2005, ISBN 3-510-65211-8 .
- Peter Ward , Joe Kirschvink : A New Story of Life. How catastrophes determined the course of evolution. Deutsche Verlags Anstalt, Munich 2016. ISBN 978-3-421-04661-1 .
- Mojib Latif ː Climate Change and Climate Dynamics. Ulmer, Stuttgart 2009, ISBN 978-3-8252-3178-1 .
- Jens Boenigk, Sabina Wodniok: Biodiversity and Earth History . Springer Verlag, Berlin - Heidelberg 2014 (Springer Spectrum), DOIː 10.1007 / 978-3-642-55389-9 , ISBN 978-3-642-55388-2 (textbook on the emergence of diversity in the geological context).
- Christoph Buchal, Christian-Dietrich Schönwiese: Climate. The earth and its atmosphere through the ages . Ed .: Wilhelm and Else Heraeus Foundation, Helmholtz Association of German Research Centers, 2nd edition. Hanau 2012, ISBN 978-3-89336-589-0 .
- Christian-Dietrich Schönwiese: climatology. 4th, revised and updated edition. UTB, Stuttgart 2013, ISBN 978-3-8252-3900-8 .
- Stefan Rahmstorf, Hans Joachim Schellnhuber: Climate change: diagnosis, prognosis, therapy. 7. completely revised & updates Edition 2012. CH Beck, Munich 2012. ISBN 978-3-406-63385-0 [book]; ISBN 978-3-406-63593-9 [eBook]
- Jochem Marotzke , Martin Stratmann (Hrsg.): The future of the climate. New insights, new challenges. A report from the Max Planck Society. Beck, Munich 2015, ISBN 978-3-406-66968-2 .
- Harald Meller , Thomas Puttkammer (Hrsg.): Klimagewalten. Driving force of evolution. wbg Theiss, Darmstadt 2017, ISBN 978-3-8062-3120-5 .
Web links
- Sigrun Laste: Climate makes history (1): From the Ice Age to ancient times. (YouTube) Story House Productions, 2015 (also in the ZDF media library ).
- Sigrun Laste: Climate makes history (2): From antiquity to the present. (YuoTube) Story House Productions, 2015 (also in the ZDF media library ).
- Climateactiontracker (English) the website of the Climate Action Tracker
- Climate change and its consequences , online course from WWF and the German Climate Consortium on iversity
- Climate change - information from the Federal Department of the Environment, Transport, Energy and Communication
Collection portals
- Climate change information portal of the Central Institute for Meteorology and Geodynamics in Austria
- Research on climate change from the Potsdam Institute for Climate Impact Research
- Climate navigator from an editorial committee of German climate researchers headed by the Climate Service Center Germany and the Helmholtz Center Geesthacht
- Document server Climate Change of the Climate Service Center Germany and the Helmholtz Center Geesthacht
- Klimawiki on the German education server
- Climate change on the information portal of the Federal Working Group on Political Education Online
- Climate change in a special topic in the magazine Geo
- Climate and climate change in the environmental accounts of the Federal Ministry for Sustainability and Tourism and Federal Statistics Austria
- Climate change in the forests of Rhineland-Palatinate from the Ministry for the Environment, Energy, Food and Forests Rhineland-Palatinate
Individual evidence
- ^ A b Richard A. Muller, Gordon J. MacDonald: Spectrum of 100-kyr glacial cycle: Orbital inclination, not eccentricity . In: Proceedings of the National Academy of Sciences . tape 94 , no. 16 , August 5, 1997, ISSN 0027-8424 , p. 8329-8334 , PMID 11607741 , PMC 33747 (free full text) - ( pnas.org [accessed March 8, 2017]).
- ↑ A. Berger, M. Cruci, DA Hodell, C. Mangili, JF McManus, B. Otto-Bliesner, K. Pol, D. Raynaud, LC Skinner, PC Tzedakis, EW Wolff, QZ Yin, A. Abe-Ouchi , C. Barbante, V. Brovkin, I. Cacho, E. Capron, P. Ferretti, A. Ganopolski, JO Grimalt, B. Hönisch, K. Kawamura, A. Landais, V. Margari, B. Martrat, V. Masson-Delmotte, Z. Mokeddem, F. Parrenin, AA Prokopenko, H. Rashid, M. Schulz, N. Vazquez Riveiros (Past Interglacials Working Group of PAGES): Interglacials of the last 800,000 years . (PDF) In: Reviews of Geophysics (AGU Publications) . 54, No. 1, March 2016, pp. 162-219. doi : 10.1002 / 2015RG000482 .
- ↑ ZDF / Terra X / Gruppe 5 / Luise Wagner, Jonas Sichert, Andreas Hougardy - Nov. 2019 ( description of the media file on Commons ). The underlying climate model and emission scenario are unknown.
- ^ Franz Mauelshagen: Climate history of the modern age . Scientific Book Society, Darmstadt 2010, ISBN 978-3-534-21024-4 , pp. 13 .
- ↑ Climate fluctuation. In: Lexicon of Geosciences. Spectrum academic publisher, accessed August 12, 2016 .
- ↑ Brockhaus Encyclopedia, Volume 26, 1996.
- ↑ Martin Kappas: Climatology. Challenge for the natural and social sciences in the 21st century . Spectrum Academic Publishing House, 2009, ISBN 978-3-8274-1827-2 .
- ↑ Climate optimum. In: Lexicon of Geosciences. Spectrum academic publisher, accessed August 12, 2016 .
- ↑ Christian-Dietrich Schönwiese : Climate changes: data, analyzes, forecasts . Springer, 1995, ISBN 3-540-59096-X , pp. 79-80 .
- ^ Richard B. Alley, Anna Maria Ágústsdóttir: The 8k event: Cause and consequences of a major Holocene abrupt climate change. In: Quaternary Science Reviews. Geosciences, May 2005, accessed May 11, 2019 .
- ↑ Robin M. Canup: Simulations of a late lunar-forming impact (PDF), Icarus, Vol. 168, 2004, pp. 433-456.
- ↑ James F. Kasting, Shuhei Ono: Palaeoclimates: the first two billion years . (PDF): The Royal Society Publishing, Philosophical Transactions B . June 2006. doi : 10.1098 / rstb.2006.1839 .
- ^ Adam R. Sarafian, Horst R. Marschall, Francis M. McCubbin, Brian D. Monteleone: Early accretion of water in the inner solar system from a carbonaceous chondrite-like source . (PDF) In: Science . 346, October 2014, pp. 623–626. doi : 10.1126 / science.1256717 .
- ^ Alan D. Rooney, Justin V. Strauss, Alan D. Brandon, Francis A. Macdonald: A Cryogenian chronology: Two long-lasting synchronous Neoproterozoic glaciations . (PDF) In: Geology . 43, No. 5, May 2015, pp. 459-462. doi : 10.1130 / G36511.1 .
- ↑ Galen P. Halverson, Ross K. Stevenson, Michelle Vokaty, André Poirier, Marcus Kunzmann, Zheng-Xiang Li, Steven W. Denyszyn, Justin V. Strauss, Francis A. Macdonald: Continental flood basalt weathering as a trigger for Neoproterozoic Snowball Earth . (PDF) In: Earth and Planetary Science Letters . 446, July 2016, pp. 89-99. doi : 10.1016 / j.epsl.2016.04.016 .
- ^ Richard J. Twitchett: The palaeoclimatology, palaeoecology and palaeoenvironmental analysis of mass extinction events . (PDF) In: Palaeogeography, Palaeoclimatology, Palaeoecology . 232, No. 2-4, March 2006, pp. 190-213. doi : 10.1016 / j.palaeo.2005.05.019 .
- ↑ David PG Bond, Stephen E. Grasby: On the causes of mass extinctions . In: Palaeogeography, Palaeoclimatology, Palaeoecology . 478, July 2017, pp. 3–29. doi : 10.1016 / j.palaeo.2016.11.005 .
- ↑ F. Jourdan, K. Hodges, B. Sell, U. Schaltegger, MTD Wingate, LZ Evins, U. Söderlund, PW Haines, D. Phillips, T. Blenkinsop: High-precision dating of the Kalkarindji large igneous province, Australia , and synchrony with the Early-Middle Cambrian (Stage 4-5) extinction . (PDF) In: Geology . 42, No. 6, June 2014, pp. 543-546. doi : 10.1130 / G35434.1 .
- ^ Benjamin C. Gill, Timothy W. Lyons, Seth A. Young, Lee R. Kump, Andrew H. Knoll, Matthew R. Saltzman: Geochemical evidence for widespread euxinia in the Later Cambrian ocean . In: Nature . 469, January 2011, pp. 80-83. doi : 10.1038 / nature09700 .
- ↑ Jennifer L. Morris, Mark N. Puttick, James W. Clark, Dianne Edwards, Paul Kenrick, Silvia Pressel, Charles H. Wellman, Ziheng Yang, Harald Schneider, Philip CJ Donoghue: The timescale of early land plant evolution . In: PNAS . 115, No. 10, March 2018, pp. E2274 – E2283. doi : 10.1073 / pnas.1719588115 .
- ↑ Timothy M. Lenton, Michael Crouch, Martin Johnson, Nuno Pires, Liam Dolan: First plants cooled the Ordovician . (PDF) In: Nature Geoscience . 5, February 2012, pp. 86-89. doi : 10.1038 / ngeo1390 .
- ↑ P. Porada, TM Lenton, A. Pohl, B. Weber, L. Mander, Y. Donnadieu, C. Beer, U. Pöschl, A. Kleidon: High potential for weathering and climate effects of non-vascular vegetation in the Late Ordovician . (PDF) In: Nature Communications . August 7, 2016. doi : 10.1038 / ncomms12 .
- Jump up ↑ Thijs RA Vandenbroucke, Howard A. Armstrong, Mark Williams, Florentin Paris, Jan A. Zalasiewicz, Koen Sabbe, Jaak Nõlvak, Thomas J. Challands, Jacques Verniers, Thomas Servais: Polar front shift and atmospheric CO 2 during the glacial maximum of the Early Paleozoic Icehouse . (PDF) In: PNAS . 107, No. 34, August 2010, pp. 14983-14986.
- ↑ David AT Hapera, Emma U. Hammarlund, Christian M. Ø. Rasmussen: End Ordovician extinctions: A coincidence of causes . (PDF) In: Gondwana Research (Elsevier) . 25, No. 4, May 2014, pp. 1294–1307. doi : 10.1016 / j.gr.2012.12.021 .
- ↑ Thijs RA Vandenbroucke, Poul Emsbo, Axel Munnecke, Nicolas Nuns, Ludovic Duponchel, Kevin Lepot, Melesio Quijada, Florentin Paris, Thomas Servais, Wolfgang Kiessling: Metal-induced malformations in early Palaeozoic plankton are harbingers of mass extinctions . In: Nature Communications . 6, August 2015. doi : 10.1038 / ncomms8966 .
- ↑ Bradley D. Cramer, Daniel J. Condon, Ulf Söderlund, Carly Marshall, Graham J. Worton, Alan T. Thomas, Mikael Calner, David C. Ray, Vincent Perrier, Ian Boomer, P. Jonathan Patchett, Lennart Jeppsson: U -Pb (zircon) age constraints on the timing and duration of Wenlock (Silurian) paleocommunity collapse and recovery during the “Big Crisis” . (PDF) In: Geological Society of America (Bulletin) . 124, No. 11-12, October 2012, pp. 1841-1857. doi : 10.1130 / B30642.1 .
- ↑ Grzegorz Racki, Michał Rakociński, Leszek Marynowski, Paul B. Wignall: Mercury enrichments and the Frasnian-Famennian biotic crisis: A volcanic trigger proved? . (PDF) In: Geology . 46, No. 6, June 2018, pp. 543-546. doi : 10.1130 / G40233.1 .
- ↑ Daizhao Chen, Jianguo Wang, Grzegorz Racki, Hua Li, Chengyuan Wang, Xueping Ma, Michael T. Whalen: Large sulfur isotopic perturbations and oceanic changes during the Frasnian – Famennian transition of the Late Devonian . (PDF) In: Journal of the Geological Society (Lyell Collection) . 170, No. 3, May 2013, pp. 465-476. doi : 10.1144 / jgs2012-037 .
- ^ David De Vleeschouwer, Micha Rakociński, Grzegorz Racki, David PG Bond, Katarzyna Sobień, Philippe Claeys: The astronomical rhythm of Late-Devonian climate change (Kowala section, Holy Cross Mountains, Poland) . (PDF) In: Earth and Planetary Science Letters . 365, March 2013, pp. 25-37. doi : 10.1016 / j.epsl.2013.01.016 .
- ^ Sarah K. Carmichael, Johnny A. Waters, Cameron J. Batchelor, Drew M. Coleman, Thomas J. Suttner, Erika Kido, LM Moore, Leona Chadimová: Climate instability and tipping points in the Late Devonian: Detection of the Hangenberg Event in an open oceanic island arc in the Central Asian Orogenic Belt . (PDF) In: Gondwana Research . 32, April 2016, pp. 213-231. doi : 10.1016 / j.gr.2015.02.009 .
- ↑ Sandra Isabella Kaiser, Markus Aretz, Ralph Thomas Becker: The global Hangenberg Crisis (Devonian – Carboniferous transition): review of a first-order mass extinction . (PDF) In: Geological Society, London, Special Publications . 423, August 2016, pp. 387-437. doi : 10.1144 / SP423.9 .
- ^ A b Georg Feulner: Formation of most of our coal brought Earth close to global glaciation . In: PNAS . 114, No. 43, October 2017, pp. 11333–11337. doi : 10.1073 / pnas.1712062114 .
- ^ A b Alexander J. Hetherington, Joseph G. Dubrovsky, Liam Dolan: Unique Cellular Organization in the Oldest Root Meristem . In: Current Biology . 26, No. 12, June 2016, pp. 1629–1633. doi : 10.1016 / j.cub.2016.04.072 .
- ^ A b Peter Franks: New constraints on atmospheric CO 2 concentration for the Phanerozoic . (PDF) In: Geophysical Research Letters . 31, No. 13, July 2014. doi : 10.1002 / 2014GL060457 .
- ↑ a b c d Isabel P. Montañez, Jennifer C. McElwain, Christopher J. Poulsen, Joseph D. White, William A. DiMichele, Jonathan P. Wilson, Galen Griggs, Michael T. Hren: Climate, pCO 2 and terrestrial carbon cycle linkages during late Palaeozoic glacial – interglacial cycles . (PDF) In: Nature Geoscience . 9, No. 11, November 2016, pp. 824–828. doi : 10.1038 / ngeo2822 .
- ↑ Michael M. Joachimski, Xulong Lai, Shuzhong Shen, Haishui Jiang, Genming Luo, Bo Chen, Jun Chen, Yadong Sun: Climate warming in the latest Permian and the Permian – Triassic mass extinction . (PDF) In: Geology . 40, No. 3, March 2012, pp. 195-198. doi : 10.1130 / G32707 .
- ^ Yadong Sun, Michael M. Joachimski, Paul B. Wignall, Chunbo Yan, Yanlong Chen, Haishui Jiang, Lina Wang, Xulong Lai: Lethally Hot Temperatures During the Early Triassic Greenhouse . (PDF) In: Science . 338, No. 6105, October 2012, pp. 366-370. doi : 10.1126 / science.1224126 .
- ↑ Shu-Zhong Shen, Jahandar Ramezani, Jun Chen, Chang-Qun Cao, Douglas H. Erwin, Hua Zhang, Lei Xiang, Shane D. Schoepfer, Charles M. Henderson, Quan-Feng Zheng, Samuel A. Bowring, Yue Wang , Xian-Hua Li, Xiang-Dong Wang, Dong-Xun Yuan, Yi-Chun Zhang, Lin Mu, Jun Wang, Ya-Sheng Wu: A sudden end-Permian mass extinction in South China . (PDF) In: GSA Bulletin (The Geological Society of America) . 131, September 2018, pp. 205-223. doi : 10.1130 / B31909.1 .
- ^ Michael J. Benton: Hyperthermal-driven mass extinctions: killing models during the Permian-Triassic mass extinction . In: Philosophical Transactions of the Royal Society A . 376, No. 2130, October 2018. doi : 10.1098 / rsta.2017.0076 .
- ↑ a b c Michael J. Benton, Andrew J. Newell: Impacts of global warming on Permo-Triassic terrestrial ecosystems . (PDF) In: Gondwana Research . 25, No. 4, May 2014, pp. 1308-1337. doi : 10.1016 / j.gr.2012.12.010 .
- ↑ Zhong-Qiang Chen, Michael J. Benton: The timing and pattern of biotic recovery following the end-Permian mass extinction . (PDF) In: Nature Geoscience . 5, No. 6, June 2012, pp. 375-383. doi : 10.1038 / ngeo1475 .
- ↑ Terrence J. Blackburn, Paul E. Olsen, Samuel A. Bowring, Noah M. McLean, Dennis V. Kent, John Puffer, Greg McHone, E. Troy Rasbury, Mohammed Et-Touhami: Zircon U-Pb Geochronology Links the End -Triassic Extinction with the Central Atlantic Magmatic Province . (PDF) In: Science . 340, No. 6135, May 2013, pp. 941-945. doi : 10.1126 / science.1234204 .
- ↑ JHFL Davies, H. Bertrand, N. Youbi, M. Ernesto, U. Schaltegger: End-Triassic mass extinction started by intrusive CAMP activity . In: Nature Communications . May 8, 2017. doi : 10.1038 / ncomms15596 .
- ↑ Yannick Donnadieu, Gilles Dromart, Yves Goddéris, Emmanuelle Pucéat, Benjamin Brigaud, Guillaume Dera, Christophe Dumas, Nicolas Olivier: A mechanism for brief glacial episodes in the Mesozoic greenhouse . In: Paleoceanography (American Geophysical Union) . 26, No. 3, September 2011. doi : 10.1029 / 2010PA002100 .
- ↑ Hubert Wierzbowski, Mikhail A. Rogov, Bronisław A. Matyja, Dmitry Kiselev, Alexei Ippolitov: Middle – Upper Jurassic (Upper Callovian – Lower Kimmeridgian) stable isotope and elemental records of the Russian Platform: Indices of oceanographic and climatic changes . (PDF) In: Global and Planetary Change . 107, 2013, pp. 196-212. doi : 10.1016 / j.gloplacha.2013.05.011 .
- ^ Bilal U. Haq: Jurassic Sea-Level Variations: A Reappraisal . (PDF) In: GSA Today (Geological Society of America) . 28, No. 1, January 2018, pp. 4–10. doi : 10.1130 / GSATG359A.1 .
- ^ NF Alley, SB Hore, LA Frakes: Glaciations at high-latitude Southern Australia during the Early Cretaceous . (PDF) In: Australian Journal of Earth Sciences (Geological Society of Australia) . April 2019. doi : 10.1080 / 08120099.2019.1590457 .
- ↑ Yongdong Wang, Huang Chengmin, Bainian Sun, Cheng Quan, Wu Jingyu, Zhicheng Lin: Paleo-CO 2 variation trends and the Cretaceous greenhouse climate . (PDF) In: Earth-Science Reviews . 129, February 2014, pp. 136–147. doi : 10.1016 / j.earscirev.2013.11.001 .
- ↑ Mingzhen Zhang, Shuang Dai, Baoxia Du, Liming Ji, Shusheng Hu: Mid-Cretaceous Hothouse Climate and the Expansion of Early Angiosperms . (PDF) In: Acta Geologica Sinica (Geological Society of China) . 92, No. 5, October 2018, pp. 2004–2025. doi : 10.1111 / 1755-6724.13692 .
- Jump up ↑ Madison East, R. Dietmar Müller, Simon Williams, Sabin Zahirovic, Christian Heine: Subduction history reveals Cretaceous slab superflux as a possible cause for the mid-Cretaceous plume pulse and superswell events . (PDF) In: Gondwana Research . 79, March 2020, pp. 125-139. doi : 10.1016 / j.gr.2019.09.001 .
- ↑ James S. Eldrett, Ian Jarvis, John S. Lignum, Darren R. Grätze, Hugh C. Jenkyns, Martin A. Pearce: Black shale deposition, atmospheric CO 2 drawdown, and cooling during the Cenomanian-Turonian Oceanic Anoxic Event . In: Paleoceanography and Paleoclimatology . 26, No. 3, September 2011. doi : 10.1029 / 2010PA002081 .
- ↑ Vanessa C. Bowman, Jane E. Francis, James B. Riding: Late Cretaceous winter sea ice in Antarctica? . (PDF) In: Geology . 41, No. 12, December 2013, pp. 1227-1230. doi : 10.1130 / G34891.1 .
- ↑ a b Margret Steinthorsdottir, Vivi Vajda, Mike Poled: Global trends of pCO 2 across the Cretaceous – Paleogene boundary supported by the first Southern Hemisphere stomatal proxy-based pCO 2 reconstruction . (PDF) In: Palaeogeography, Palaeoclimatology, Palaeoecology . 464, December 2016, pp. 143–152. doi : 10.1016 / j.palaeo.2016.04.033 .
- ↑ Stephen L. Brusatte, Richard J. Butler, Paul M. Barrett, Matthew T. Carrano, David C. Evans, Graeme T. Lloyd, Philip D. Mannion, Mark A. Norell, Daniel J. Peppe, Paul Upchurch, Thomas E. Williamson: The extinction of the dinosaurs . In: Biological Reviews, Cambridge Philosophical Society (Wiley Online Library) . 90, No. 2, May 2015, pp. 628–642. doi : 10.1111 / brv.12128 .
- ↑ Michael J. Henehan, Andy Ridgwell, Ellen Thomas, Shuang Zhang, Laia Alegret, Daniela N. Schmidt, James WB Rae, James D. Witts, Neil H. Landman, Sarah E. Greene, Brian T. Huber, James R. Super, Noah J. Planavsky, Pincelli M. Hull: Rapid ocean acidification and protracted Earth system recovery followed the end-Cretaceous Chicxulub impact . In: PNAS . 116, No. 43, October 2019. doi : 10.1073 / pnas.1905989116 .
- ↑ Julia Brugger, Georg Feulner, Stefan Petri: Baby, it's cold outside: Climate model simulations of the effects of the asteroid impact at the end of the Cretaceous . In: Geophysical Research Letters . 44, No. 1, January 2017, pp. 419-427. doi : 10.1002 / 2016GL072241 .
- ↑ Jennifer B. Kowalczyk, Dana L. Royer, Ian M. Miller, Clive W. Anderson, David J. Beerling, Peter J. Franks, Michaela Grein, Wilfried Konrad, Anita Roth ‐ Nebelsick, Samuel A. Bowring, Kirk R. Johnson, Jahandar Ramezani: Multiple Proxy Estimates of Atmospheric CO 2 From an Early Paleocene Rainforest . (PDF) In: Paleoceanography and Paleoclimatology . 33, No. 12, December 2018, pp. 1427–1438. doi : 10.1029 / 2018PA003356 .
- ↑ a b c d Richard E. Zeebe, Andy Ridgwell, James C. Zachos : Anthropogenic carbon release rate unprecedented during the past 66 million years . (PDF) In: Nature Geoscience . 9, No. 4, April 2016, pp. 325–329. doi : 10.1038 / ngeo2681 .
- ↑ Camilla M. Wilkinson, Morgan Ganerød, Bart WH Hendriks, Elizabeth A. Eide: Compilation and appraisal of geochronological data from the North Atlantic Igneous Province (NAIP) . In: Geological Society, London, Special Publications (Lyell Collection) . 447, November 2016, pp. 69-103. doi : 10.1144 / SP447.10 .
- ↑ Alexander Gehler, Philip D. Gingerich, Andreas Pack: Temperature and atmospheric CO 2 concentration estimates through the PETM using triple oxygen isotope analysis of mammalian bioapatite . In: PNAS . 113, No. 28, July 2016, pp. 7739-7744. doi : 10.1073 / pnas.1518116113 .
- ^ Francesca A. McInerney, Scott L. Wing: The Paleocene-Eocene Thermal Maximum: A Perturbation of Carbon Cycle, Climate, and Biosphere with Implications for the Future . (PDF) In: Annual Review of Earth and Planetary Sciences . 39, May 2011, pp. 489-516. doi : 10.1146 / annurev-earth-040610-133431 .
- ↑ Henk Brinkhuis, Stefan Schouten, Margaret E. Collinson, Appy Sluijs, Jaap S. Sinninghe Damsté, Gerald R. Dickens, Matthew Huber, Thomas M. Cronin, Jonaotaro Onodera, Kozo Takahashi, Jonathan P. Bujak, Ruediger Stein, Johan van der Burgh, James S. Eldrett, Ian C. Harding, André F. Lotter, Francesca Sangiorgi, Han van Konijnenburg-van Cittert, Jan W. de Leeuw, Jens Matthiessen, Jan Backman, Kathryn Moran: Episodic fresh surface waters in the Eocene Arctic Ocean . (PDF) In: Nature . 441, 2006, pp. 606-609. doi : 10.1038 / nature04692 . Retrieved May 25, 2017.
- ^ Dennis V. Kent, Giovanni Muttoni: Equatorial convergence of India and Early Cenozoic climate trends . In: PNAS . 105, No. 42, October 2008, pp. 16065-16070. doi : 10.1073 / pnas.0805382105 .
- ↑ Mark Pagani, Matthew Huber, Zhonghui Liu, Steven M. Bohaty, Jorijntje Henderiks, Willem Sijp, Srinath Krishnan, Robert M. DeConton: The Role of Carbon Dioxide During the Onset of Antarctic Glaciation . (PDF) In: Science . 334, No. 6060, December 2011, pp. 1261-1264. doi : 10.1126 / science.1203909 .
- ↑ Simone Galeotti, Robert DeConto, Timothy Naish, Paolo Stocchi, Fabio Florindo, Mark Pagani, Peter Barrett, Steven M. Bohaty, Luca Lanci, David Pollard, Sonia Sandroni, Franco M. Talarico, James C. Zachos: Antarctic Ice Sheet variability across the Eocene-Oligocene boundary climate transition . (PDF) In: Science . 352, No. 6281, April 2016, pp. 76-80. doi : 10.1126 / science.aab0669 .
- ↑ KT Lawrence, S. Sosdian, HE White, Y. Rosenthal: North Atlantic climate evolution through the Pliocene-Pleistocene climate transitions . (PDF) In: Earth and Planetary Science Letters . 300, No. 3-4, December 2010, pp. 329-342. doi : 10.1016 / j.epsl.2010.10.013 .
- ^ Matteo Willeit, Andrey Ganopolski, Reinhard Calov, Alexander Robinson, Mark Maslin: The role of CO 2 decline for the onset of Northern Hemisphere glaciation . (PDF) In: Quaternary Science Reviews . 119, July 2015, pp. 22–34. doi : 10.1016 / j.quascirev.2015.04.015 .
- ↑ a b Peter Marcott, Jeremy D. Shakun, Peter U. Clark, Alan C. Mix: A Reconstruction of Regional and Global Temperature for the Past 11,300 Years . (PDF) In: Science . 6124, No. 269, March 2013, pp. 1198-1201. doi : 10.1126 / science.1228026 .
- ^ Nir J. Shaviv: Toward a solution to the early faint Sun paradox: A lower cosmic ray flux from a stronger solar wind . In: Journal of Geophysical Research . 108, no.A12 , December 2003. doi : 10.1029 / 2003JA009997 .
- ↑ Anatoly D. Erlykin, David AT Harper, Terry Sloan, Arnold W. Wolfendale: Mass extinctions over the last 500 myr: an astronomical cause? . In: Palaeontology . 60, No. 2, March 2017, pp. 159–167. doi : 10.1111 / pala.12283 .
- ^ Dana L. Royer, Robert A. Berner, Isabel P. Montañez, Neil J. Tabor, David J. Beerling: CO 2 as a primary driver of Phanerozoic climate . (PDF) In: GSA Today (American Geophysical Union) . 14, No. 3, March 2004, pp. 4-10. doi : 10.1130 / 1052-5173 (2004) 014 <4: CAAPDO> 2.0.CO; 2 .
- ↑ Daniel Siegel: Global climate change caused by the sun? Fluctuations in radiation intensity (PDF). Kiepenheuer Institute for Solar Physics, Freiburg 2010.
- ↑ Holger Braun, Marcus Christl, Stefan Rahmstorf, Andrey Ganopolski, Augusto Mangini, Claudia Kubatzki, Kurt Roth, Bernd Kromer: Possible solar origin of the 1,470-year glacial climate cycle demonstrated in a coupled model . (PDF) In: Nature . 438, November 2005, pp. 208-211. doi : 10.1038 / nature04121 .
- ↑ Nicola Scafetta, Franco Milani, Antonio Bianchini, Sergio Ortolanic: On the astronomical origin of the Hallstatt oscillation found in radiocarbon and climate records throughout the Holocene . (PDF) In: Earth-Science Reviews . 162, June 2016, pp. 24–43. doi : 10.1016 / j.earscirev.2016.09.004 .
- ^ Klaus Dethloff et al .: Nonlinear Dynamics of the Climate System . In: Hubertus Fischer et al. (Ed.): The Climate in Historical Times . Springer, 2004, ISBN 3-540-20601-9 , chap. 2 , p. 33 .
- ^ John A. Matthews, Keith R. Briffa: The 'Little Ice Age': Re-evaluation of an Evolving Concept . In: Geografiska Annaler: Series A, Physical Geography . 2005, doi : 10.1111 / j.0435-3676.2005.00242.x .
- ↑ Mathew J. Owens, Mike Lockwood, Ed Hawkins, Ilya Usoskin, Gareth S. Jones, Luke Barnard, Andrew Schurer and John Fasullo: The Maunder Minimum and the Little Ice Age: an update from recent reconstructions and climate simulations . In: Journal of Space Weather and Space Climate . tape 7 , A33, 2017, doi : 10.1051 / swsc / 2017034 .
- ↑ Friedhelm Steinhilber, Jose A. Abreu, Jürg Beer, Irene Brunner, Marcus Christl, Hubertus Fischer, Ulla Heikkilä, Peter W. Kubik, Mathias Mann, Ken G. McCracken, Heinrich Miller, Hiroko Miyahara, Hans Oerter, Frank Wilhelms: 9,400 years of cosmic radiation and solar activity from ice cores and tree rings . In: PNAS . 109, No. 16, April 2012, pp. 5967-5971. doi : 10.1073 / pnas.1118965109 .
- ↑ P. Foukal, C. Fröhlich, H. Spruit, TML Wigley: Variations in solar luminosity and their effect on the Earth's climate . (PDF) In: Nature . 443, September 2006, pp. 24-43. doi : 10.1038 / nature05072 .
- ↑ Mike Lockwood, Claus Fröhlich: Recent oppositely directed trends in solar climate forcings and the global mean surface air temperature . In: Proceedings of the Royal Society A . 463, No. 2086, October 2007. doi : 10.1098 / rspa.2007.1880 .
- ↑ Judith L. Lean, David H. Rind: How natural and anthropogenic influences alter global and regional surface temperatures: 1889 to 2006 . In: Geophysical Research Letters . 35, No. 18, September 2008. doi : 10.1029 / 2008GL034864 .
- ^ Antonello Pasini, Umberto Triacca, Alessandro Attanasio: Evidence of recent causal decoupling between solar radiation and global temperature . In: Environmental Research Letters . 7, No. 3, September 2012. doi : 10.1088 / 1748-9326 / 7/3/034020 .
- ↑ G. Myhre, D. Shindell et al. a .: Anthropogenic and Natural Radiative Forcing . In: TF Stocker u. a. (Ed.): Climate Change 2013: The Physical Science Basis. Contribution of Working Group I to the Fifth Assessment Report of the Intergovernmental Panel on Climate Change . 2013, p. 661, 688-691 ( ipcc.ch [PDF; 19.4 MB ]).
- ↑ Jacob D. Haqq-Misra, Shawn D. Domagal-Goldman, Patrick J. Kasting, James F. Kasting: A Revised, Hazy Methane Greenhouse for the Archean Earth. In: Astrobiology. Vol. 8, No. 6, pp. 1127-1137 (2008). doi: 10.1089 / ast.2007.0197 .
- ↑ F. Parrenin, V. Masson-Delmotte, P. Köhler, D. Raynaud, D. Paillard, J. Schwander, C. Barbante, A. Landais, A. Wegner, J. Jouze: Synchronous Change of Atmospheric CO 2 and Antarctic Temperature During the Last Deglacial Warming . (PDF) In: Science . 339, No. 6123, March 2013, pp. 1060-1063. doi : 10.1126 / science.1226368 .
- ↑ a b A. Ganopolski, R. Winkelmann, HJ Schellnhuber: Critical insolation - CO 2 relation for diagnosing past and future glacial inception . In: Nature . 529, No. 7585, January 2016, pp. 200-203. doi : 10.1038 / nature16494 .
- ^ Edwin Kemper: The climate of the Cretaceous period . (= Geological Yearbook. Series A, Issue 96). Published by the Federal Institute for Geosciences and Raw Materials and the State Geological Offices in the Federal Republic of Germany, E. Schweizerbart'sche Verlagsbuchhandlung, Stuttgart 1987, ISBN 3-510-96400-4 , p. 105, p. 111 ff.
- ^ A b Dennis V. Kent, Paul E. Olsen, Cornelia Rasmussen, Christopher Lepre, Roland Mundil, Randall B. Irmis, George E. Gehrels, Dominique Giesler, John W. Geissman, William G. Parker: Empirical evidence for stability of the 405-kiloyear Jupiter – Venus eccentricity cycle over hundreds of millions of years . In: PNAS . 115, No. 24, June 2018, pp. 6153–6158. doi : 10.1073 / pnas.1800891115 .
- ↑ Ilja J. Kocken, Margot J. Cramwinckel, Richard E. Zeebe, Jack J. Middelburg, Appy Sluijs: The 405 kyr and 2.4 Myr eccentricity components in Cenozoic carbon isotope records . (PDF) In: Climate of the Past . January 15, 2019, pp. 91-104. doi : 10.5194 / cp-15-91-2019 .
- ^ V. Ramanathan, RJ Cicerone, HB Singh, JT Kiehl: Trace gas trends and their potential role in climate change . (PDF) In: Journal of Geophysical Research . 90, No. D3, June 1985, pp. 5547-5566. doi : 10.1029 / JD090iD03p05547 .
- ^ Stefan Rahmstorf: Climate change - some facts . In: From Politics and Contemporary History (APuZ 47/2007).
- ↑ Animation by CIRES / NOAAː Representation of the carbon dioxide concentration in the atmosphere using different time scales .
- ^ Yi Ge Zhang, Mark Pagani, Zhonghui Liu, Steven M. Bohaty, Robert DeConto: A 40-million-year history of atmospheric CO 2 . (PDF) In: The Royal Society (Philosophical Transactions A) . 371, No. 2001, September 2013. doi : 10.1098 / rsta.2013.0096 .
- ↑ James Hansen , Makiko Sato, Pushker Kharecha, David Beerling, Robert Berner, Valerie Masson-Delmotte, Mark Pagani, Maureen Raymo, Dana L. Royer, James C. Zachos : Target Atmospheric CO 2 : Where Should Humanity Aim? In: The Open Atmospheric Science Journal. Vol. 2, 2008, pp. 217-231, doi: 10.2174 / 1874282300802010217 (PDF)
- ↑ Eric Monnin, Andreas Indermühle, André Dällenbach, Jacqueline Flückiger, Bernhard Stauffer, Thomas F. Stocker , Dominique Raynaud, Jean-Marc Barnola: Atmospheric CO 2 Concentrations over the Last Glacial Termination. In: Science. Vol. 291, no. 5501, January 5, 2001, pp. 112-114, doi: 10.1126 / science.291.5501.112
- ^ Noah S. Diffenbaugh, Christopher B. Field: Changes in Ecologically Critical Terrestrial Climate Conditions . In: Science . 341, No. 6145, August 2013, pp. 486-492. doi : 10.1126 / science.1237123 .
- ↑ Frequently Asked Question 6.2: Is the Current Climate Change Unusual Compared to Earlier Changes in Earth's History? Climate Change 2007: Working Group I: The Physical Science Basis ( English ) IPCC. 2007. Retrieved May 20, 2016.
- ↑ Susan Solomon, Gian-Kasper Plattner, Reto Knutti , Pierre Friedlingstein: Irreversible climate change due to carbon dioxide emissions . In: PNAS . 106, No. 6, February 2009, pp. 1704-1709. doi : 10.1073 / pnas.0812721106 .
- ^ Richard E. Zeebe: Time-dependent climate sensitivity and the legacy of anthropogenic greenhouse gas emissions . In: PNAS . 110, No. 34, August 2013, pp. 13739-13744. doi : 10.1073 / pnas.1222843110 .
- ↑ Timothy M. Lenton, Hermann Held, Elmar Kriegler, Jim W. Hall, Wolfgang Lucht, Stefan Rahmstorf, Hans Joachim Schellnhuber: Tipping elements in the Earth's climate system . In: PNAS . 105, No. 6, 2008, pp. 1786-1793. doi : 10.1073 / pnas.0705414105 .
- ↑ a b Kyle G. Pressel, Colleen M. Kaul, Tapio Schneider: Possible climate transitions from breakup of stratocumulus decks under greenhouse warming . (PDF) In: Nature Geoscience . 12, No. 3, March 2019, pp. 163–167. doi : 10.1038 / s41561-019-0310-1 .
- ↑ Lesson 1 starter activity: Ranking the seven continents (PDF). Publication by the Royal Geographical Society.
- ^ Neil J. Tabor: Wastelands of tropical Pangea: High heat in the Permian . In: Geology . 41, No. 5, March 2013, pp. 623-624. doi : 10.1130 / focus052013.1 .
- ↑ Spencer G. Lucas, Joerg W. Schneider, Giuseppe Cassinis: Non-marine Permian biostratigraphy and biochronology: an introduction. In: Spencer G. Lucas, Giuseppe Cassinis, Joerg W. Schneider (Eds.): Non-Marine Permian Biostratigraphy and Biochronology. Geological Society, London, Special Publications, 265, London 2006, pp. 1-14. (PDF)
- ↑ Isabel P. Montañez, Neil J. Tabor, Deb Niemeier, William A. DiMichele, Tracy D. Frank, Christopher R. Fielding, John L. Isbell, Lauren P. Birgenheier, Michael C. Rygel: CO 2 -Forced Climate and Vegetation Instability During Late Paleozoic Deglaciation . (PDF) In: Science . 315, No. 5808, January 2007, pp. 87-91. doi : 10.1126 / science.1134207 .
- ^ Andrew C. Scott: The diversification of Paleozoic fire systems and fluctuations in atmospheric oxygen concentration . In: PNAS . 103, No. 29, May 2006, pp. 10861-10865. doi : 10.1073 / pnas.0604090103 .
- ↑ Peter Ward, Joe Kirschvink: A New Story of Life. How catastrophes determined the course of evolution. 2016, ISBN 978-3-421-04661-1 .
- ↑ Mark Pagani, Matthew Huber, Zhonghui Liu, Steven M. Bohaty, Jorijntje Henderiks, Willem Sijp, Srinath Krishnan, Robert M. DeConton: The Role of Carbon Dioxide During the Onset of Antarctic Glaciation . (PDF) In: Science . 334, No. 6060, December 2011, pp. 1261-1264. doi : 10.1126 / science.1203909 .
- ^ Brian J. Soden, Richard T. Wetherald, Georgiy L. Stenchikov, Alan Robock: Global Cooling After the Eruption of Mount Pinatubo: A Test of Climate Feedback by Water Vapor . (PDF) In: Science . 296, April 2002, pp. 727-730. doi : 10.1126 / science.296.5568.727 .
- ^ HR Pruppacher, JD Klett: Microphysics of Clouds and Precipitation . In: Springer Netherlands (Ed.): Atmospheric and Oceanographic Sciences Library . 2nd Edition. No. 18 . Springer Science, 2010, ISBN 978-0-306-48100-0 .
- ↑ Dennis Hartmann: Global Physical Climatology . Ed .: Elsevier. 2nd Edition. Elsevier Science, 2015, ISBN 978-0-08-091862-4 .
- ^ Anne-Lise Chenet, Frédéric Fluteau, Vincent Courtillot: Modeling massive sulphate aerosol pollution, following the large 1783 Laki basaltic eruption . (PDF) In: Earth and Planetary Science Letters . 236, No. 3-4, August 2005, pp. 721-731. doi : 10.1016 / j.epsl.2005.04.046 .
- ^ Thomas Wagner, Christoph Hörmann, Marloes Penning de Vries, Holger Sihler: Global monitoring of volcanic emissions with satellite instruments . Research report 2011, Max Planck Institute for Chemistry
- ↑ Volcanic Gases and Climate Change Overview . Volcano Hazards Program, USGS (US Geological Survey).
- ^ Robert B. Smith, Lawrence W. Braile: Crustal Structure and Evolution of an Explosive Silicic Volcanic System at Yellowstone National Park . In Geology of Yellowstone Park Area; 33rd Annual Field Conference Guidebook , 1982, pp. 233-250.
- ↑ Thomas R. Knott, Michael J. Branney, Marc K. Reichow, David R. Finn, Simon Tapster, Robert S. Coe: Discovery of two new super-eruptions from the Yellowstone hotspot track (USA): Is the Yellowstone hotspot waning ? . (PDF) In: Geology . June 2020. doi : 10.1130 / G47384.1 .
- ↑ Ben G. Mason, David M. Pyle, Clive Oppenheimer: The size and frequency of the largest explosive eruptions on Earth . (PDF) In: Bulletin of Volcanology . 66, No. 8, December 2004, pp. 735-748. doi : 10.1007 / s00445-004-0355-9 .
- ^ A b David PG Bond, Paul B. Wignall: Large igneous provinces and mass extinctions: An update . (PDF) In: The Geological Society of America (GSA) Special Paper . 505, September 2014, pp. 29-55. doi : 10.1130 / 2014.2505 (02) .
- ↑ Mark A. Richards, Walter Alvarez, Stephen Self, Leif Karlstrom, Paul R. Renne, Michael Manga, Courtney J. Sprain, Jan Smit, Loÿc Vanderkluysen, Sally A. Gibson: Triggering of the largest Deccan eruptions by the Chicxulub impact . (PDF) In: Geological Society of America Bulletin . April 2015. doi : 10.1130 / B31167.1 .
- ↑ Barbara P. Nash, Michael E. Perkins: Neogene Fallout Tuffs from the Yellowstone Hotspot in the Columbia Plateau Region, Oregon, Washington and Idaho, USA . In: PLOS One . October 2012, p. E44205. doi : 10.1371 / journal.pone.0044205 .
- ↑ Jennifer Kasbohm, Blair Schoene: Rapid eruption of the Columbia River flood basalt and correlation with the mid-Miocene climate optimum . (PDF) In: Science Advances . 4, No. 9, September 2018. doi : 10.1126 / sciadv.aat8223 .
- ^ Joel R. Norris, Robert J. Allen, Amato T. Evan, Mark D. Zelinka, Christopher W. O'Dell, Stephen A. Klein: Evidence for climate change in the satellite cloud record. In: Nature. 536, 2016, p. 72, doi: 10.1038 / nature18273 .
- ↑ World Meteorological Organization , press release 4/2017 , March 21, 2017: Climate breaks multiple records in 2016, with global impacts (March 21, 2017)
- ↑ IPCC Fourth Assessment Report: Climate Change 2007 (AR4) Intergovernmental Panel on Climate Change, 2007
- ↑ DR Feldman, WD Collins, PJ Gero, MS Torn, EJ Mlawer, TR Shippert: Observational determination of surface radiative forcing by CO 2 from 2000 to 2010 . (PDF) In: Nature . 519, March 2015, pp. 339–343. doi : 10.1038 / nature14240 .
- ↑ a b IPCC: Climate Change 2014: Synthesis Report. Contribution of Working Groups I, II and III to the Fifth Assessment Report of the Intergovernmental Panel on Climate Change . Ed .: Core Writing Team, RK Pachauri and LA Meyer. IPCC, Geneva, Switzerland 2015, ISBN 978-92-9169-143-2 , pp. 5 ( ipcc.ch [PDF]).
- ↑ Philip Kokic, Steven Crimp, Mark Howden: A probabilistic analysis of human influence on recent record global mean temperature changes. In: Climate Risk Management. 3, 2014, pp. 1–12, doi: 10.1016 / j.crm.2014.03.002 .
- ^ Raphael Neukom, Nathan Steiger, Juan José Gómez-Navarro, Jianghao Wang, Johannes P. Werner: No evidence for globally coherent warm and cold periods over the preindustrial Common Era . (PDF) In: Nature . 571, July 2019, pp. 550-554. doi : 10.1038 / s41586-019-1401-2 .
- ^ PAGES 2k Consortium: Consistent multidecadal variability in global temperature reconstructions and simulations over the Common Era . (PDF) In: Nature Geoscience . 12, No. 8, August 2019, pp. 643–649. doi : 10.1038 / s41561-019-0400-0 .
- ↑ Darrell Kaufman, Nicholas McKay, Cody Routson, Michael Erb, Christoph Dätwyler, Philipp S. Sommer, Oliver Heiri, Basil Davis: Holocene global mean surface temperature, a multi-method reconstruction approach . In: Nature Scientific Data . June 7, 2020. doi : 10.1038 / s41597-020-0530-7 .
- ↑ J. Hansen, M. Sato, P. Hearty, R. Ruedy, M. Kelley, V. Masson-Delmotte, G. Russell, G. Tselioudis, J. Cao, Eric Rignot , I. Velicogna, E. Kandiano, K. von Schuckmann, P. Kharecha, AN Legrande, M. Bauer, K.-W. Lo: Ice melt, sea level rise and superstorms: evidence from paleoclimate data, climate modeling, and modern observations that 2 ° C global warming is highly dangerous . (PDF) In: Atmospheric Chemistry and Physics (Discussions) . 15, No. 14, 2015, pp. 20059–20179. doi : 10.5194 / acpd-15-20059-2015 .
- ↑ nytimes.com , April 19, 2017, Jessica Benko: How a Warming Planet Drives Human Migration (April 23, 2017)
- ↑ Rafael Reuveny: Climate change-induced migration and violent conflict . (PDF) In: Political Geography (Elsevier) . 26, No. 6, August 2007, pp. 656-673. doi : 10.1016 / j.polgeo.2007.05.001 .
- ^ H. Damon Matthews, Ken Caldeira : Stabilizing climate requires near-zero emissions . In: Geophysical Research Letters . tape 35 , 2008, doi : 10.1029 / 2007GL032388 .
- ↑ “The last generation that is able to do something about it”. In: welt.de. November 29, 2018, accessed February 16, 2020 .