paleoclimatology
The task of paleoclimatology is to use measurements, analyzes and data series from so-called climate archives ( proxy data ) to reconstruct the climatic conditions of the geological past (the paleoclimate ) in the form of a climate history and the mechanisms of climate change events in the various geological or historical ages Decipher epochs. Methodologically, it has always been a sub-area of historical geology , but it also shows parallels to modern, physically shaped climatology . As an interdisciplinary science, the scope of which covers almost the entire history of the earth , paleoclimatology is based on findings from paleontology , paleogeography , meteorology , and oceanography and cooperates with fields such as atmospheric chemistry and geophysics . Research results from astronomy and astrophysics have been increasingly taken into account in the last few decades .
With the instruments of paleoclimatology, well-founded statements can be made to a greater extent about past and future climate developments on earth . The latter concerns both developments that have already started, such as global warming , and events further in the future, such as the recurrence of a cold period . In addition, paleoclimatologically determined data are used to more precisely determine the climate sensitivity as well as to research the causes and consequences of abrupt climate changes .
Research history
The development from the 17th to the 19th century
As early as the 17th century, Nicolaus Steno found a well-founded explanation of the formation of sedimentary rocks with the Stratigraphic Basic Law . He correctly recognized that different layers of rock represent different stages in the history of the earth . Fossil finds in the high mountains, for example from sea shells, provided clear indications that the history of the earth was not static, but was shaped by profound dynamic processes. Also in the 17th century, geological and paleontological climate witnesses were associated with long-term climate changes for the first time . In 1686 , the English polymath Robert Hooke suspected on the basis of fossils from the Jura that the southern English climate must have been considerably warmer in the distant past.
Against the then firmly rooted belief in the biblical creation myth with the flood as a global "primordial catastrophe", to which all deposits known at the time, including the fossils, were ascribed, the idea of a primeval epoch could only prevail a century later. In the course of the Enlightenment and with the development of geology into modern science from 1750, the idea of prehistoric times gradually gained ground. At the end of the 18th century, this resulted in the then revolutionary realization that the prehistoric times of the earth had to encompass a considerably larger period than the historically documented human history. The layers, sediments and fossils analyzed on the basis of the first stratigraphic profiles led to the assumption of a long-lasting deposition and fossilization process. In addition, unusual relics in the form of moraines , drumlins and erratic blocks ( boulders ), which indicated large-scale glaciation , were found especially in the foothills of the Alps, in the north German lowlands or in Scandinavia . In the first decades of the 19th century, the initially rough division of the earth's history into different geological periods fell . At the same time, these periods were classified in the geological time scale , although the true temporal dimensions were greatly underestimated until the 20th century due to inadequate geochronological analysis methods. In addition, the first scientific descriptions of prehistoric habitats including their climatic conditions emerged .
The botanist and geologist Karl Friedrich Schimper (1803–1867) is considered to be the pioneer of paleoclimatology in the German-speaking world . He was the first to describe fossil-based traces of weather influences such as rain, wind and hailstones. In addition, he advocated the theory of a “world winter” that covered large parts of northern and central Europe with ice. The Swiss palaeontologist and botanist Oswald Heer (1809-1883) created his main work, the seven-volume “Flora fossilis arctica - The fossil flora of the polar countries” , a much-noticed standard work on paleobotany . With the successful, generally understandable book Die Urwelt der Schweiz (1865), he succeeded in attracting a broader audience to the idea of prehistoric times. Heer was one of the first to propose using fossil-preserved plants as proxies for determining the climate of primeval habitats . The Swiss natural scientist Louis Agassiz (1807–1873), who emigrated to the USA in 1846 and continued his scientific career there , also achieved international fame . On the basis of intensive field studies in the Swiss Alps, Agassiz came to the conclusion that certain terrain formations could only have developed under the influence of general icing. This thesis sparked a long, controversial discussion. Many researchers were opposed to the new Ice Age theory and preferred alternative explanatory models instead. The boulders that are often found in some areas were considered to be volcanic ejections. This was all the more astonishing, as the much older permocarbon glaciation , well documented by geological studies in India, South Africa and Australia, had long since found its way into specialist literature.
It was not until between 1870 and 1880, given an abundance of consistent evidence, that the existence of the Quaternary Ice Age was generally accepted. From now on, the last glacial period - also with regard to the climate - was the central topic of much geoscientific research for decades. The Swedish physicist and chemist Svante Arrhenius (1859–1927) made a significant contribution to the understanding of climate mechanisms in geological periods . In his work On the Influence of Carbonic Acid in the Air on the Ground Temperature (1896) he was the first to calculate the exact greenhouse effect of carbon dioxide, pointed to fluctuations in the concentration of this gas during the Ice Age cycles and assumed an upcoming global warming due to industrial CO 2 emissions. Arrhenius' work on this issue has long been considered uncertain and speculative, but with some delay it was fully confirmed in the 1950s.
The 20th century

The 20th century brought an influx of new knowledge to the geosciences , from which paleoclimatology also benefited, which now had an increasingly broader and more reliable base. The three-volume standard work The Alps in the Ice Age , published by Albrecht Penck and Eduard Brückner between 1901 and 1909 , which comprehensively described the four Alpine Ice Ages Günz , Mindel , Riss and Würm and provided a pioneering stratigraphic basis, became a milestone in Ice Age and Quaternary research established this subject area. In 1911, the British geologist Arthur Holmes used the uranium-lead decay series for the first time to determine the absolute age of rock layers. His measurements showed an age of around 600 million years for the beginning of the Cambrian (currently 541 million years). Holmes' results were initially questioned many times, but correspond relatively precisely with the modern geological timescale.
Another pioneer of paleoclimatology was the meteorologist and polar researcher Alfred Wegener (1880–1930), who also found posthumous recognition as a geoscientist as the founder of the continental drift theory , which was not received until the 1960s . In order to support his thesis, according to which the current arrangement of the continents is only a geological snapshot, Wegener collected a large number of "climate witnesses" that were supposed to prove that the large land masses in earlier geological periods were far from their current position and probably parts of what was once the ancient continent Pangea were. Among other things, he referred to the coal deposits formed under warm-time conditions in the Antarctic , to the fossil finds of subtropical tree species on Svalbard or to the discovery that the Sahara was partly covered by glaciers in the late Ordovician .
Martin Schwarzbach (1907–2003) is considered the father of modern, systematically operated paleoclimatology . His textbook Das Klima der Vorzeit (first edition 1950) was updated and revised over a period of four decades. As a representative of classical actualism , Schwarzbach only marginally considered the diverse new approaches in paleoclimatology and their rapid development into a broad, interdisciplinary science.
The current foundations of paleoclimatology
Until well into the second half of the 20th century, paleoclimatology , like most other geosciences, was a predominantly descriptive (descriptive) science. For this purpose, she made use of a growing number of constantly refined dating and detection methods such as dendrochronology , which is also frequently used in historical climatology and archeology . However, in order to be able to make well-founded statements not only about climate events, but also about their physical causes and interactions as well as their significance for the present, the essential factors of the earth's climate system first had to be understood. This could only be done within the framework of a broad-based, interdisciplinary science that fully takes into account all climate-relevant control mechanisms and is in intensive exchange with other scientific disciplines. For example, the astrophysicist and book author Carl Sagan and his co-author George Mullen pointed out in a study in 1972 that the sun emitted about 30 percent less heat radiation at the beginning of Earth's history 4.5 billion years ago than it does today. The Faint Young Sun Paradox ( paradox of the weak young sun ) arose elementary questions about the origin and continuity of earthly life, which not only concern paleoclimatology, but are currently being discussed on a broad basis in an interdisciplinary manner, especially in the atmospheric sciences .

Interest similar to the Paradox sparked in 1980 with the discovery of a globally documented Iridium anomaly at the Cretaceous-Paleogene boundary . The increased concentration of the precious metal iridium, which is very rare on earth, in a thin layer of sediment (the so-called "boundary tone") led to the assumption of an asteroid impact at the end of the Middle Ages 66 million years ago, which severely polluted the entire ecosystem and caused worldwide mass extinction . The most likely location of the impact is the Chicxulub crater in the Gulf of Mexico near the Yucatán peninsula . In the meantime, dozens of other impact craters have been located with the help of satellites (some of them almost completely eroded or buried), which clearly show that the earth collided relatively frequently with cosmic objects of different sizes in geological time periods. At the same time, there was increasing evidence that severe climatic fluctuations and the associated mass extinctions occurred more often than originally assumed. The causes and consequences of such crises are currently being intensively researched in order to find possible parallels to anthropogenic global warming.
Model calculations using computers have established themselves as an important instrument in the representation of past and future climate scenarios. Due to their complex structure and their number of additional parameters, climate models place high demands on the computing capacity of a computer system. Most of the models are calibrated using real climate processes, both in the present and in the past, so that they can not only reproduce current developments, but also, for example, the climate cycle of the last Ice Age to a large extent correctly. When modeling climatic developments, the Earth's orbit parameters, the so-called Milanković cycles , which change over the course of millennia , have become a significant influencing factor. With the inclusion of these cycles, it was possible to put the characteristic course of the Quaternary Ice Age with its warm and cold periods, including the greenhouse effect and the ice-albedo feedback , on a solid theoretical foundation.
The progress made in radiometric dating over the past few decades has led to a considerable increase in measurement accuracy and thus to a partial re-evaluation of geological, geophysical and biological events. With the help of modern dating methods, it has become possible to narrow down climate fluctuations or mass extinctions more precisely and to reconstruct them in increasing detail.
Methods and analysis tools
Reliable and relatively complete data on weather and climate are only available to meteorology and climatology for the period of the last 150 years. In order to be able to make well-founded statements about the climates of earlier epochs, modern paleoclimatology has a number of special measuring and determination methods, some of which have only recently been developed. Standard instruments include climate proxies as indirect climate indicators that can be found in natural archives such as tree rings , stalagmites , ice cores , corals , sea or ocean sediments , pollen or written records. Climate proxies are not only used to reconstruct past climate zones, but also provide information on solar activity, rainfall intensity, air composition and chemical composition of prehistoric seas. In order to rule out incorrect results as far as possible, climate proxies must be compared with modern, instrumentally determined data series and calibrated on them.
Climate proxies and climate witnesses
In the course of intensive research into the Quaternary Ice Age from the middle of the 19th century, an abundance of geological relics were discovered that indicated a long cold phase. Above all, the Fennoscan Ice Sheet , which covers large parts of Central and Northern Europe, and the Alpine foreland glaciers left characteristic traces in the most varied of terrain formations or were directly involved in the formation of these formations in the form of trough valleys or ground moraines . These processes are the subject of research on glacial morphology , which also examines numerous other witnesses to Ice Age glacier movements and glacial processes, such as glacial till , glacial cut , dropstones , loess sediments , periglacial layers and (with restrictions) so-called ice wedges . With the help of geological, paleontological and radiometric methods, six ice ages with a total duration of 525 million years have been detected during the last 2.4 billion years, with relatively extensive glaciers occasionally occurring even in warmer phases of the earth's history.
Geological and palaeontological detection methods are also used for earlier warm periods, with an additional distinction being made here between moist (humid) and dry (arid) climates. The location and extent of primeval coral reefs , the composition of clay minerals including layered silicates , deposits of lignite (shale coal), the sedimentary rock evaporite and the various forms of physical, chemical and biotic weathering in geological periods are suitable as dating and research objects .
Below are a number of other climate practices that are commonly used in paleoclimatology.
- With the dendrochronology , the annual tree growth can be reconstructed depending on the weather, the environment and the climate by analyzing the annual rings. In this way, complete annual ring tables were created for individual European tree species over a period of 10,000 years. The current “record holder” is the Hohenheim Tree Ring Calendar , which shows the Central European climate development from the present to the Younger Dryas period 14,600 years ago. Under optimal conditions it is possible to assign the exact year of its creation to each tree ring. For example, the weather anomaly of the years 535 and 536 was scientifically confirmed using dendrochronology .
- The palynology (pollen analysis) under the name Pollenstratigraphie a portion of Paleontology , and most recently in paleoclimatology won also important. Thanks to their global distribution and their great resistance to environmental influences and geological processes, primeval pollen, spores and microfossils (summarized under the term palynomorphs ) from the early Phanerozoic to the geological present are very suitable as guide fossils. In addition, not only the climatic conditions of the time, but also complex ecosystems can be reconstructed from the local abundance and species diversity of the palynomorphs .
- The varven chronology , also called band tone dating , is based on the exact counting of deposit layers (varves) in still and flowing waters such as lakes or rivers. Waters that are regularly affected by heavy snowmelt are particularly suitable for this. If the count can be integrated into an absolute time frame, this enables the age to be specified in varve years . With appropriate calibration and comparison of the varven years with other chronological methods, palaeoclimatological detailed analyzes based on small-scale time periods are possible , similar to dendrochronology . The scope of the varven chronology extends over a time frame of several hundred to about 30,000 years and in individual cases extends beyond this.

- Ice cores are among the most accurate climate archives and are therefore very methodically analyzed and evaluated. In addition to mountain glaciers, whose drill cores can be used to reconstruct the exact regional climatic processes over the last millennia under favorable conditions, the Greenland and Antarctic ice sheets are suitable for detailed climate analyzes over longer periods of time. While the oldest Greenland ice examined so far covers around 123,000 years and thus includes the Eem warm period ,an Antarctic core with a total age of over 800,000 years was recovered aspart of the EPICA project. The "fossil" air bubbles within an ice core are reliable climate archives for the composition of the atmosphere during the Quaternary Ice Age and especially for the carbon dioxide and methane concentrations that were subject to strong fluctuations within an ice age cycle with its cold and warm phases. Ice cores also provide data on solar activity, air temperatures, evaporation and condensation processes, and anomalies in the earth's magnetic field. Dust particles trapped in the ice are indicators of wind and atmospheric circulation and also store the traces of possible volcanic eruptions and meteorite impacts.
- Oceanic sediments . Thedeposit layers that have formedon the continental shelves or in the deep sea over millions of yearsare divided into biogenic (dead organisms), lithogenic (rocks) and hydrogen (soluble chemical compounds) sediments with regard to their origin. The drill core samples of biogenic sediments allow conclusions to be drawn about the geographical distribution of certain living beings in different geological epochs, lithogenic sediments are an archive for changes in the state of ocean currents , while hydrogen sediments often contain information on past climatic fluctuations. By evaluating iron-bearing sediments and magma layers in the oceanic crust , a number of polar reversals could also be detected. Studies of this kind are the subject of research in paleomagnetism . The age of all marine sediments islimitedby the plate tectonic process of subduction . Since ocean floors are constantly "plunging" into the depths of the earth's mantle and, on the other hand, are constantly being formedat the spreading zones , the average age of the entire oceanic crust is around 80 million years. Individual regions reach an age of around 200 million years (only in the eastern Mediterranean there is a major exception, 340 million year old sediment layers from the Carboniferous period ). Because of this natural time barrier, the impact craters of large asteroid or comet impacts in the Precambrian or Paleozoic seas are no longer detectable. The exact dating of oceanic drill core samples usually varies greatly and is dependent on their age and the speed of the respective sedimentation processes. Deposits from the Holocene allow, under favorable conditions, a temporal resolution of several decades, whereby very recent stratifications arerather unsuitable for reliable analyzesdue to influences such as bioturbation .
- Dripstones such as stalagmites and stalactites (not always properly called speleothems ) occur worldwide and are almost inevitably to be found in the caves of karst and limestone areas. Stalactites arise from surface water enriched with carbon dioxide (e.g. rain or meltwater), which on its way through crevices and porous material absorbs organic acids which, in combination with the carbon dioxide,dissolve the calcium carbonate contained in the rock. As long as the environmental conditions of the respective cave remain constant, thin layers of limestone are formed by seeping water droplets, which over time“grow”into stalagmites (from the cave floor) or stalactites (from the cave ceiling). The ratio of the oxygen isotopes in stalactite limestone, the thickness of the growth layers and the proportions of various trace elements add up to a reliable, decades-accurate climate calendar, which alsorecordsabrupt and brief changes such as the Dansgaard-Oeschger events of the last ice age. Dripstones can - depending on the duration of the water and thus the calcium carbonate supply - grow for a very long time and sometimes reach an age of several hundred thousand years.
Isotope analysis and biogeochemical detection method
Dating methods
- Zircon crystals are often used to achieve the most precise absolute age possible . Due to their heat resistance and their lattice structure, which has remained stable as a result, they are suitable for analyzing the radioactive nuclides enclosed therein (such as 235 U, 238 U or 232 Th). This dating method has a very low margin of error and covers the entire period of earth's history. For example, zircons can prove the existence of early plate tectonic processes (and thus the time when the first oceans were formed) as well as the exact age of impact craters .
- The 40 Ar / 39 Ar dating is a modified and more accurate method of the conventional potassium-argon dating and has been used extensively in the geosciences for some time to determine the age of minerals and rocks. Their scope extends from a few millennia to well into the Precambrian.
- The crypto-dating with the isotope 81 Kr in conjunction with the stable isotope 83 Kr is used in practice only since 2011 on. The breakthrough came with a new detector technology based on Atom Trap Trace Analysis . With a half-life of 230,000 years, 81 Kr is particularly suitable for examining glaciers and old ice layers, such as those found in the Antarctic, and provides considerably more precise results than previous methods.
- The radiocarbon method , also called 14 C dating , is a method for determining the age of organic substances. From the natural fluctuations of the radioactive carbon isotope 14 C and the stable isotope 12 C, the cycles of solar activity, changes in the geomagnetic dipole field and the exchange between carbon sinks and the atmosphere can be calculated. Application range: 300 to about 57,000 years. Due to its time limit, 14 C dating only plays a subordinate role in paleoclimatology , but is widely used in historical climatology and archeology . According to a recent study, the rapidly increasing anthropogenic CO 2 emissions lead to a significant reduction in the 14 C content in the atmosphere. This effect will make future radiocarbon dating with high probability considerably more difficult or significantly falsify it.
Methods for the reconstruction of climate and environment
- δ 13 C (Delta-C-13) is the measure of the ratio of the stable carbon isotopes 13 C / 12 C between a sample and a defined standard. The shift in the δ 13 C ratio discovered in this way in rock formations that are 3.5 billion years old is a strong indicator of the existence of early life forms. The δ 13 C signature also allows the atmospheric carbon dioxide concentration to be determined in different geological ages. The release of large amounts of methane hydrate, such as during the Paleocene / Eocene temperature maximum , also has a significant impact on the global δ 13 C signature.
- δ 18 O (Delta-O-18) describes the ratio of the stable oxygen isotopes 18 O / 16 O. This versatile measuring method is suitable for the reconstruction of precipitation temperatures and also serves as an indicator of processes of isotope fractionation such as methanogenesis . In paleoclimatology, 18 O / 16 O data is used as a temperature proxy for fossil corals and foraminifera, as well as ice cores , dripstones and freshwater sediments. Temporal scope: Cenozoic to Paleozoic, partly beyond (at least 600 million years).
- δ 15 N (Delta-N-15) is the measure of the ratio of the stable nitrogen isotopes 15 N to 14 N. This methodology examines various forms of the nitrogen cycle , for example the rate at which nitrogen is absorbed by an ecosystem and is implemented.
- TEX86 (tetraether index of 86 carbon atoms) describes a biogeochemical method for determining the sea surface temperature of previous climates. For this purpose, the cell membrane of certain marine protozoa is analyzed. Temporal scope: Jurassic, Cretaceous and entire Cenozoic (approx. The last 200 million years).
In addition to the methods mentioned above, there are a number of other analytical methods, such as strontium isotope analysis . The radioactive beryllium isotope 10 Be, which is only present in traces on earth, correlates with cosmic radiation and with high aerosol concentrations. 10 Be isotopes in ice cores are also analyzed with regard to the relationship between solar activity and temperature development. In addition, a number of iron, chromium and noble gas isotopes are used for geological and palaeoclimatological investigations. A very young method, which is currently still in its early stages, is the special use of the argon isotope 39 Ar for the analysis of glacial ice and oceanic deep water using Atom Trap Trace Analysis (ATTA) . This method is based on a magneto-optical “atomic trap” (MOT) using laser physics for trace analysis of rare noble gas isotopes, whereby each atom of the sample material is detected individually.
Long-term effective climate factors in the context of geological history
The sun

Of all the factors that have shaped the earth's climate from the beginning and continue to determine it today, the influence of the sun plays the most important role. The solar energy generated and emitted in a thermonuclear fusion process is the basic requirement for the origin and development of life on earth. The radiation intensity averaged over many years in the form of the solar constant is currently 1367 W / m². Due to the eccentricity of the earth's orbit , the strength of the incident solar radiation outside the atmosphere varies between 1325 W / m² and 1420 W / m² over the course of a year. The term solar constant is somewhat misleading because it is subject to cyclical fluctuations - albeit within narrow limits (around 0.1 percent both in the visible range and in the total radiation). These fluctuations are causally linked to the maximum and minimum periods of the sunspots and thus to the different activity cycles of the sun.
The development of the sun as the main sequence star in the Hertzsprung-Russell diagram is of primary importance on the entire time scale of earth's history . After a relatively short period as protostar she began 4.6 billion years ago with the energy released by the exothermic process of nuclear fusion , in which the intercalated in the solar core supply of hydrogen through the proton-proton reaction gradually in helium is converted. This stage lasts around 11 billion years, during which time the luminosity and radius of the sun will constantly increase or have already increased significantly. This means that the sun at the beginning of its existence (and at the same time at the beginning of the earth's history) emitted only 70 percent of the current thermal radiation and that this radiation increased by an average of 1 percent every 150 million years up to the current value.
The atmosphere
When it was formed, the earth was likely to have had a primordial atmosphere , the main components of which were hydrogen and helium and which also contained traces of methane , ammonia and some noble gases . This gas mixture only existed for a relatively short time, because the thermal effects of several impact catastrophes and the influence of the solar wind and the solar magnetic field , especially the light elements, quickly evaporated into the interplanetary space.
The earth's first atmosphere was created more than four billion years ago and was essentially the result of extremely strong volcanism with correspondingly intense outgassing of carbon dioxide , nitrogen and sulfur dioxide . Since precipitation evaporated immediately on the heated surface of the earth, water vapor dominated the very dense and hot atmosphere with a share of around 80 percent. This was followed by carbon dioxide and hydrogen sulfide in proportions of around 10 and 6 percent, respectively.
Presumably there was already large amounts of liquid water on the young earth at an early stage, so that towards the end of the Hadean , around 4 billion years ago, the first oceanic basins were formed. There are several theories about the origin of terrestrial water, whereby in addition to a purely terrestrial origin, extraterrestrial sources such as protoplanetary disks , comets or meteorites are also increasingly being discussed. With the emergence and spread of life in the course of the Eoarchean 4 to 3.6 billion years ago, single-celled organisms such as the archaea first had a direct influence on the atmosphere by gradually increasing the methane content with their metabolic products. At the same time, carbon dioxide was withdrawn from the atmosphere and dissolved in large quantities in seawater. Since the carbon dioxide made a significant contribution to the build-up of biomass, the pH of the oceans gradually increased in a multi-stage process , which resulted in the precipitation and extensive deposition of carbonates . The inert ( inert ) nitrogen was not involved in these biochemical processes, so its concentration rose steadily over time until it became its main component 3.4 billion years ago when the development of the second atmosphere came to an end.
The formation of the third atmosphere was closely linked to the appearance of free oxygen . It is very likely that cyanobacteria that used oxygen-phototrophic photosynthesis already existed more than 3 billion years ago . The oxygen released did not initially get into the atmosphere, but was consumed in the oxidation of various iron compounds and sulphides dissolved in the water . Only after this long-lasting oxidation process was completed could the available excess penetrate into the atmosphere as free oxygen. There it triggered a breakdown in methane concentration 2.4 billion years ago due to its oxidative effect. This turning point, known as the Great Oxygen Catastrophe, led to the mass extinction of almost all anaerobic life forms and subsequently to serious climate change. It is very likely that the 300 million year Paleoproterozoic glaciation (also known as the Huronian Ice Age ) was the direct consequence of methane shortages and an increase in oxygen.
Towards the end of the Precambrian , possibly a little later, oxygen diffused into the stratosphere in significant amounts , and an ozone layer formed on the basis of the ozone-oxygen cycle . From then on, this protected the earth's surface from the sun's UV radiation and thus enabled the subsequent colonization of the continents by flora and fauna. Shortly after the beginning of the ancient world , the atmospheric oxygen content increased rapidly. At the beginning of the Carboniferous around 350 million years ago, it first reached today's value of 21 percent and rose to 35 percent towards the end of the period. In the further course of the earth's and climatic history, the atmosphere was repeatedly subject to strong changes, depending on biological and geophysical influences. The oxygen, carbon dioxide and methane concentrations fluctuated considerably and played a direct or indirect role in a number of climate change events.
Greenhouse gases
Although there are a number of climate-relevant greenhouse gases such as nitrous oxide (laughing gas), sulfur hexafluoride or carbonyl sulfide , almost exclusively carbon dioxide (CO 2 ) and methane (CH 4 ) are of importance with regard to climatic development over geological periods . In contrast to nitrogen, oxygen and all noble gases, greenhouse gases are infrared radiation active thanks to their molecular structure , which means that they can absorb heat energy at wavelengths of 4.26 µm and 14.99 µm and re-emit it towards the ground . Due to this greenhouse effect , the near-surface average temperature in the mathematical-physical model increases by around 33 ° C to +15 ° C. Without the greenhouse effect, the lower atmosphere would only have a global mean of −18 ° C and lead to a complete icing of the planet (whereby the temperature level would probably drop even further due to several interactions).
The most important and, in terms of its overall effect, the strongest greenhouse gas is water vapor , whose share in the natural greenhouse effect fluctuates between 36 and 70 percent. Since the atmospheric water vapor content depends to a large extent on the air temperature, its concentration decreases at lower average temperatures and increases during a warming phase ( water vapor feedback ).
The atmospheric concentration of carbon dioxide is usually given in ppm (= parts per million), that of methane in ppb (= parts per billion). Due to human influence , the content of carbon dioxide has increased to over 400 ppm (previously 280 ppm) and that of methane to 1800 ppb (previously 800 ppb) since the beginning of the industrial age . These are the highest concentrations for at least 800,000 years, but there were epochs with considerably larger proportions, such as in the Paleozoic around 500 million years ago, when the CO 2 concentration was at times in the range of 5000 to 6000 ppm. Comparisons and conclusions to the present are problematic, however, since the conditions at that time (including the reduced radiation output of the sun and the complete absence of land plants) cannot be transferred to the Holocene in any way .
Carbon dioxide and / or methane were not always the main drivers of climate change. Sometimes they functioned in the history of the earth as "feedback links" that strengthened, accelerated or weakened developments. In this context, in addition to the Earth's orbit parameters , feedback processes such as ice-albedo feedback , vegetation cover and the variability of the water vapor content in the atmosphere must be taken into account.
Above all, the carbon dioxide, in the form of a “CO 2 thermostat”, made a significant contribution to the fact that the earth's temperature corridor remained relatively constant over billions of years, so that liquid water and associated life could exist under these conditions. Nevertheless, there were always borderline situations, such as the snowball-earth events in the Neoproterozoic or the super greenhouse on the Permian-Triassic border , which caused serious environmental changes.
Volcanism
While methane is produced by various biotic , chemical and geological processes, the atmospheric CO 2 originally comes from the outgassing of volcanic and plate tectonic activities. In return, carbon dioxide is continuously stored in the earth's crust through weathering and sedimentation and thus withdrawn from the atmosphere or the ocean. This creates several interlinked cycles of different duration in which litho- , hydro- , bio- and atmosphere are involved. In the lithosphere , the earth's outer rock layer, over 99 percent of the global carbon supply of an estimated 75 million gigatons is stored.
The earth's volcanoes currently emit a “moderate” volume of CO 2 of 180 to 440 megatons per year. The anthropogenic CO 2 emissions are several orders of magnitude higher and have reached around 36 gigatons in recent years.
A short phase of intense volcanism or individual eruptions with the strength VEI-7 (like that of Tambora in 1815) cause a global cooling over several years, which is mainly based on the dampening of sunlight by ash and aerosol particles . On geological time scales, however, volcanoes have been an important factor in the long-term inorganic carbon cycle since the beginning of the earth's history . There were times, like during the Snowball Earth events in the Neoproterozoic or the Cambrian , when the carbon cycles came to an almost complete standstill or were at least significantly disrupted and were only reactivated by the permanent volcanic CO 2 influx into the atmosphere. On the other hand, long-lasting eruption processes can considerably destabilize the terrestrial biosphere. Below are some momentous climatic and biological crises of the last 540 million years, in which volcanic events played a decisive role.
- Kalkarindji volcanic province (Western Australia), approx. 510 million years ago ( Cambrian , transition from 4th to 5th stage). The Kalkarindji event, triggered by a series of large-scale eruptions, is likely to be directly related to the first major mass extinction of multicellular organisms due to severe climate and environmental changes. The flood basalts released at that time still cover an area of 2 million km². Due to the strong increase in anoxic zones in the oceans, it is estimated that half of all marine life fell victim to the disaster.
- Siberian Trapp (west and north Siberian lowlands, central Siberian mountains), approx. 250 million years ago ( Perm-Triassic border ). The Siberian Trapp originally extended over an area of probably 7 million km² and was active for at least 600,000 years. There are many indications that the volcanic outgassing, which in addition to carbon dioxide also released huge amounts of sulfur dioxide and nitrogen oxides , contributed significantly to the largest known mass extinction in geological history at the transition from Permian to Triassic .
- Dekkan-Trapp (Dekkan region, western India), approx. 66 million years ago ( Upper Cretaceous / Maastrichtian ). The original extent of the trap is likely to have been 1.5 million km². There are various reports about the duration of its formation, ranging from 500,000 to several million years. According to predominantly scientific opinion, the Dekkan Trapp is probably out of the questionas the primary cause of the mass extinction on the Cretaceous-Paleogene border , but it is often assumed that it caused the terrestrial ecosystemto a still unknown extentbefore the impact of the Chicxulub meteorite was affected.

In terms of their explosive power and the amount of lava , ash and aerosols they emit, super volcanoes areamong the most devastating events in recent geological history. They are classified in the highest categoryon the volcano explosion index with the value VEI-8. In contrast to most other volcanoes, super volcanoesdo not leave volcanic conesbehind after an eruption, due to the size of their magma chamber , but huge calderas . The last super volcano eruption occurred on the northern main island of New Zealand around 26,500 years ago in the area of what is now Lake Taupo . Another eruption occurred in Sumatrawith the Toba explosion 74,000 years ago. According to the controversially discussed Toba catastrophe theory , humanity at that time was on the verge of extinction and had topassa so-called “ genetic bottleneck ”. There are several potential supervolcanoes that could hit VEI-8 if they erupt again. The most famous of them is located under Yellowstone National Park in the US state of Wyoming . This hot spot has been active for at least 17 million years, with its most recent eruptions occurring on average about every 650,000 years. The magma chamber of the Yellowstone super volcano has a volume of at least 15,000 km³.
Since all supervolcano eruptions took place in prehistoric times, the consequences can only be documented in outline. The occurrence of violent earthquakes and - depending on the geographic location of the volcano - the development of tsunamis are likely . Through the ejection of pyroclastic material , every life was destroyed within a radius of at least 100 km within a very short time. The area covered with volcanic ash was, however, much larger and should have covered millions of square kilometers. Events of this magnitude have changed the global climate for decades or more and triggered a volcanic winter due to the aerosols and dust particles dispersed in the atmosphere . It is very likely that this resulted in local mass extinctions with a sharp decrease in biodiversity .
Position and arrangement of the continents
By geographical definition, there are seven continents on earth (North and South America are counted separately). The drifting apart of the continental plates over geological periods and their widely distributed arrangement are the result of a development that began more than 150 million years ago. During the Paleozoic Era and over parts of the Mesozoic Era , however, major and supercontinents shaped the topographical image of the earth. As a result of this amalgamation, air and ocean currents emerged that deviated significantly from the current weather systems and climatic zones.
A land mass that combines nearly all continental plates or cratons is called a supercontinent . A number of supercontinents have been known since the Precambrian , some of which are only hypothetical due to insufficient stratigraphic evidence. Nevertheless, it is considered likely that the formation and disintegration of supercontinents are embedded in plate tectonic cycles of several hundred million years each. The geologically youngest supercontinent, Pangea, existed from the late Paleozoic to the Mesozoic (310 to 150 million years ago), although the first signs of disintegration appeared as early as the late Triassic . At the height of its expansion, Pangea extended from the northern polar region to Antarctica and had an area of 138 million km², including all shelf seas , of which 73 million km² were in the southern hemisphere with the former major continent Gondwana .
Characteristic for large and super continents are a pronounced continental climate with an annual temperature amplitude of up to 50 ° C, large arid and desert areas in the interior as well as a low biodiversity in the fauna area . In the case of Pangea , a seasonally occurring, very strong monsoon influence (“mega-monsoons”) developed parallel to the equator between latitude 30 ° north and 30 ° south , from whose precipitation the coastal regions in particular benefited.
In addition, can be a basic condition for the emergence of a wide-ranging icing - namely the coverage of at least one polar region through large areas of land - using the geographic position of Gondwana or Pangea clearly prove some regions of these continents were over a period of at least 80 million years ago in Antarctica or in their immediate vicinity, in the Mississippium 359 to 318 million years ago, today's southern Africa and large areas of South America. In the second glaciation phase (in Pennsylvania 318 to 299 million years ago) the core zones of the ice sheets shifted in the course of the gradual rotation from Pangea to the cratons of India and Australia, before the southern one during the Dwyka glacial (up to 280 million years ago) Africa ( Namibia ) again became the center of an icing. The Permocarbone Ice Age was the second longest ice age in the history of the earth. It comprised a large part of the Carboniferous and ended in the course of the Permian about 265 to 260 million years ago.
A collision of continental shields always caused an unfolding of the crustal rocks and the formation of mountain chains (collision mountains). Long-term volcanism at the plate boundaries regularly occurred, with a corresponding impact on the global climate. As soon as conditions stabilized and volcanism subsided, weathering and erosion processes became the dominant climatic factor They withdrew large amounts of carbon dioxide from the atmosphere and thus contributed to global cooling. This process was reinforced by the fact that erosion-inhibiting grasslands are a relatively late development and only appeared worldwide in the Cenozoic . After a more or less long phase of tectonic calm, the continental shields broke apart again at their “seams” under violent volcanic eruptions, whereby new climatic zones and oceanic currents could establish themselves.
An example of this is the Drake Strait , which is now 480 nautical miles wide , which connects the Atlantic with the Pacific Ocean . Up until 40 million years ago there was a land bridge between Antarctica and South America , before the Drake Strait gradually began to open and deepen. This created the strongest ocean current on earth in the Southern Ocean , the Antarctic Circumpolar Current , which from now on orbited Antarctica in a clockwise direction, cut off the continent from the supply of warmer seawater and created the basis for the formation of the Antarctic Ice Sheet . Thus, Antarctica was not only geographically isolated, but also thermally. The first significant glaciation in the Oligocene , more than 30 million years ago, was synonymous with the beginning of the Cenozoic Ice Age , and in the course of the Pliocene the ice cover reached the current extent of around 14 million km² for the first time.
Orbit parameters
The assumption that long-term fluctuations in the global climate could be based on cyclical changes in the Earth's axis and orbit was discussed on various occasions as early as the second half of the 19th century. The geophysicist and mathematician Milutin Milanković (1879–1958) was able to provide an in-depth presentation on the basis of complex calculations . His work provided for the first time an explanatory model for serious climate change events, such as those last occurred during the Quaternary Ice Age and which are apparently closely related to the variability of the Earth's orbit parameters . Milanković's explanatory model, which was created over many years and whose summary was published in 1941, takes into account the periodic fluctuations of the elliptical earth's orbit ( eccentricity ), the inclination of the earth's axis and the gyration of the earth's body around its axis of rotation ( precession ). The precession is essentially caused by gravitational interactions between the sun, earth and moon; the planets Jupiter , Saturn and Venus are also involved in the different degrees of eccentricity of the earth's orbit . The cycles named after Milanković have one thing in common: Each of their changes automatically influences the distribution and, in some cases, the intensity of solar radiation on earth. Since, according to the current state of knowledge, the Milanković cycles are too weak to be considered as the primary drive for the entire climate history, they seem to function primarily as "impulses" in the climate system. When modeling climate processes, additional factors and feedback effects must therefore be taken into account.
The following table summarizes the most important key data of the Milanković cycles.
Orbit parameters | Cycle duration | Fluctuation range | Current status |
---|---|---|---|
Precession of the earth's axis of rotation | approx. | 25,800 years360 ° (full circle) within a complete cycle | Development for the more concise formation of the seasons in the northern hemisphere with longer winters |
Angle of inclination of the earth's axis to the ecliptic | approx. | 41,000 yearsbetween 22.1 ° and 24.5 ° | 23.43 ° (tending towards the minimum) |
Eccentricity of the earth's orbit | approx. 100,000 or 405,000 years 1) | from 0.0006 (almost circular) to 0.058 (slightly elliptical) | 0.016 (with a tendency to circular orbit) |
The Milanković cycles can be demonstrated as a significant climate factor over a period of 500 million years, even in the predominantly tropical climates of the Cretaceous period . Above all, the 405,000-year cycle that controls eccentricity formed a stable cosmic “clock” over large parts of the Phanerozoic and, according to new analyzes , can be traced back to the Upper Triassic around 215 million years ago and classified chronologically. According to more recent findings, the periodic changes in eccentricity could also influence the carbon cycle within the various earth spheres . The cycles developed a lasting effect, especially during different glacial periods, whereby their influence on the course of the Quaternary Ice Age can be well modeled due to their temporal proximity. This led science to consider whether a high atmospheric proportion of carbon dioxide, as recorded almost continuously in the history of the earth, could buffer the potential for change in the orbit parameters above a certain threshold value and dampen it accordingly.
For decades, experts hardly noticed the Milankovic cycles , which were judged to be speculative . Since the 1980s, however, the theory has become an integral part of paleoclimatology and Quaternary research in a modified and expanded form (including the earth's orbital plane, which Milutin Milanković did not take into account ) and is widely used to reconstruct the Ice Age cycles.
Hypothetical extraterrestrial influences
Supernovae and gamma-ray bursts

As early as 1954, the German paleontologist Otto Heinrich Schindewolf suspected that cosmic catastrophes could be responsible for rapid climate and fauna changes in the history of the earth, which is why drastic environmental changes should be examined under the aspect of radiation exposure from supernovae outbreaks. The high-energy radiation of a near-earth supernova would probably have considerable consequences for the earthly atmosphere, for example through the conversion of nitrogen into nitrogen oxides and the resulting destruction of the ozone layer. Especially the Ordovician mass extinction (late Ordovician 440 million years ago) is occasionally associated with an extraterrestrial cause. A supernova signature in the form of the iron isotope 60 Fe is an indication of such an event . This isotope, which cannot form under terrestrial conditions and which has a half-life of 2.62 million years (according to a new definition from 2009), was found in oceanic sediments of the early Quaternary ( Gelasium stage) 2.2 million years ago and detected in the Lower Pleistocene (Calabrian) 1.5 million years ago. According to astrophysical analyzes, the 60 Fe anomalies originate from supernovae eruptions about 300 light years away. Whether and to what extent the terrestrial fauna was affected by hard radiation at that time has not yet been sufficiently clarified.
Since the first secure evidence of a gamma-ray burst ( gamma-ray burst , abbreviated GRB ) in 1973 gamma-ray burst scenarios are discussed as triggers of previous environmental crises. The origin and origin of most gamma-ray bursts have not yet been fully clarified. They often only last a few seconds, but during this period they release more energy than the sun has during its entire existence. Therefore, at least in theory, there is the possibility that even more distant gamma-ray sources have the potential to permanently damage the terrestrial biosphere .
Cosmic rays
Under cosmic rays (or cosmic rays ) refers to the relatively uniform normally particle flow from various regions of the universe, the collided with the gas molecules of the outer atmosphere, resulting in a shower of secondary particles formed. In recent times, various attempts have been made to prove the influence of cosmic rays on the climate in geological periods. Although such a connection cannot be ruled out in principle, the idea was not accepted by experts due to the lack of a reliable database. Thus, the assumed long-term effect of cosmic rays on the earthly climate remains a weakly confirmed hypothesis for the time being. In the CLOUD experiment at the CERN nuclear research center , the influence of ions on the nucleation of aerosols is currently being investigated under the aspect of climatic relevance.
Other climate-affecting factors
In addition to the points described above, there are a number of other mechanisms which, depending on the prevailing climatic conditions, were differently pronounced at different times. Some of these mechanisms are integrated into a long-term cycle, such as the various forms of weathering processes that have been an important influencing factor throughout the history of the earth. Other factors, however, only seldom came into play over the course of several hundred million years, but were able to permanently reshape the terrestrial biosphere within geologically short periods of time . Many air conditioning components fulfill the function of “adjusting screws” in a complex system that reacts to every partial change with a change in the overall structure. For this reason, climatic events on a monocausal basis are practically excluded, since even a global temperature rise primarily caused by greenhouse gases (as is currently the case with global warming ) is linked to a large number of interactions. Of the factors listed below, the effect of the weakening ice albedo feedback is currently of particular importance, especially with regard to polar amplification .
- Aerosols are liquid or solid suspended particles linked to a carrier gas (mineral dust, volcanic ash, natural and industrial combustion products). In the form of hygroscopic particles, aerosols as condensation nuclei caninfluencethe formation of clouds . In addition, depending on the concentration, chemical composition and atmospheric distribution, they contribute briefly to a cooling or, more rarely, to a warming of the climate (aerosol feedback) .
- Albedo is the measure of the reflectivity of non-luminous surfaces. In the earth system, the albedo is an important factor in the radiation balance . For example, ice and snow surfaces have an albedo in the range of 0.85 (which corresponds to a reflection of 85 percent), while free seasurfaces havean albedo of around 0.2 and consequently absorb more heat energy than they reflect. The total albedo of the earth depends essentially on the extent of the oceans, ice sheets, deserts and vegetation zones and can therefore change in the medium or long term together with the radiation balance .
- The biotic climate factors include the mass distribution or extensive reduction of organisms that cause climate-relevant effects through the fixation or production of greenhouse gases. In the history of the earth these were, for example, corals , various methane generators , phytoplankton , foraminifera or plants such as the floating fern Azolla .
- The carbonate and silicate weathering is a major, acting for extended periods of climate factor that comes to different degrees depending on the prevailing environmental conditions such as hot or cold periods to advantage. The atmosphere continuously withdraws carbon dioxide by chemical weathering processes and in the lithosphere bound (lithogenic carbon cycle) . Part of the stored CO 2 is returned to the atmosphere over the course of hundreds of thousands or millions of years in the course of the carbonate-silicate cycle through the outgassing of continental or oceanic volcanoes.
- The ice-albedo feedback describes a positive feedback effect in the climate system, through which the snow and ice cover (especially in the polar regions) continues to increase during atmospheric cooling. The ice-albedo feedback is particularly important during the transition from a warm to a cold period, as it accelerates and intensifies the icing and cooling processes.
- Impact events on a large scale can not only destabilize the biosphere to a considerable extent andcausemass extinctions such as that at the Cretaceous-Paleogene border , but also influence the climate over longer periods of time ( impact winter that sets in abruptlyfollowed by a strong warming phase over several tens of thousands of years, possibly longer ). So far, around 180 impact structures with a size of more than 5 to 10 km have been detected on Earth, only around two dozen of them in oceanic sediments. There is therefore reason to assume that a relatively large number of impact events are still unknown and can only be indirectly substantiated, if at all, by a sudden mass extinction or an abrupt climate change. These are likely to include several very large impacts that have had a significant global impact.
- Sea level fluctuations (eustasia) are based on two main causes, which have often overlapped in the course of the earth's history 1. Changes in sea water volumedue to the binding of water in continental ice sheets or due to their melting (glacial eustasia) ; 2. Changes in the volume of the ocean basin as a result of tectonic shifts, for example through the formation of new oceanic crust . As a result of these processes, significant increases or decreases in sea level in the range of 100 to 200 meters are possible with corresponding climatic consequences.
- The plate tectonics in a sense is the driving force for climate change over geological time. Their influence on the earth's climate is not limited to the formation of volcanic zones; factors such as mountain formations, the location and size of the continents and the associated weather systems or oceanic currents are also directly related to plate tectonics .
Significant paleoclimatic events
The earth formed 4.57 billion years ago from several colliding protoplanets of different sizes. According to the collision theory , it is said to have obtained its current mass from a collision with a Mars-sized celestial body called Theia 4.52 billion years ago. The meeting of Theias with the proto-earth happened according to computer calculations with the low speed according to cosmic standards of 4 km / s and was not a frontal collision (which would have destroyed both planets), but a hard scratching. As a result, parts of the earth's mantle and numerous fragments of Theia were thrown into orbit , from which the initially glowing moon formed within 10,000 years . Its distance to the earth was initially only 60,000 km (according to other simulations even less and therefore only just above the Roche limit ). The lunar gravitational effect exceeded today's value by at least 125 times and exerted a strong formative influence on the still unstable earth's mantle. This effect was reinforced by the fact that the duration of an earth's rotation and thus the length of the day during the Hadaicum was in the range of ten to twelve hours. When the first oceans and probably also the first "mainland islands " formed four billion years ago, the tidal change generated extreme tidal waves that rolled incessantly across the earth.
At about the same time, a series of impact events began, triggered by numerous planetesimals (precursors of protoplanets ). This Large bombardment (English Late Heavy Bombardment ) happened from 4.1 to 3.8 billion years ago and was named after the analysis of moon rocks postulated that during the Apollo missions was collected. A calculation based on the number of known lunar craters showed that over 20,000 planetesimals between 1 km and 50 km in size could have crashed to Earth within this period. However, in more recent studies, both the intensity and the relatively tight timeframe of the Great Bombardment are increasingly being questioned.
No reliable statements can be made about the climatic conditions of the earliest geological history due to a lack of usable data. Fossil traces and geological proxies only existed from 3.8 billion years ago, from which mostly hypothetical conclusions about the climate system can be derived. On the basis of this fragmentary evidence, it is assumed that, with the exception of the presumably local Pongola glaciation 2.9 billion years ago , a relatively warm climate prevailed in the entire Archean , due to high greenhouse gas concentrations. This phase ended in the early Proterozoic with the transition into a long ice age.
Paleoproterozoic glaciation
Aeonothem | Arathem | system | Age ( mya ) |
|
---|---|---|---|---|
Phanerozoic Duration: 541 Ma |
Cenozoic Modern Earth Duration: 66 Ma |
quaternary | 0 ⬍ 2.588 |
|
Neogene | 2,588 ⬍ 23.03 |
|||
Paleogene | 23.03 ⬍ 66 |
|||
Mesozoic Middle Ages Duration: 186.2 Ma |
chalk | 66 ⬍ 145 |
||
law | 145 ⬍ 201.3 |
|||
Triad | 201.3 ⬍ 251.9 |
|||
Paleozoic Paleozoic period: 288.8 Ma |
Perm | 251.9 ⬍ 298.9 |
||
Carbon | 298.9 ⬍ 358.9 |
|||
Devon | 358.9 ⬍ 419.2 |
|||
Silurian | 419.2 ⬍ 443.4 |
|||
Ordovician | 443.4 ⬍ 485.4 |
|||
Cambrian | 485.4 ⬍ 541 |
|||
P r ä k a m b r i u m Length: 4,059 Ma |
Proterozoic Duration: 1,959 Ma |
Neoproterozoic Young Proterozoic Duration: 459 Ma |
Ediacarium | 541 ⬍ 635 |
Cryogenium | 635 ⬍ 720 |
|||
Tonium | 720 ⬍ 1000 |
|||
Mesoproterozoic Middle Proterozoic Duration: 600 Ma |
Stenium | 1000 ⬍ 1200 |
||
Ectasium | 1200 ⬍ 1400 |
|||
Calymmium | 1400 ⬍ 1600 |
|||
Paleoproterozoic Ancient Proterozoic Duration: 900 Ma |
Statherium | 1600 ⬍ 1800 |
||
Orosirium | 1800 ⬍ 2050 |
|||
Rhyacium | 2050 ⬍ 2300 |
|||
Siderium | 2300 ⬍ 2500 |
|||
Archean Period: 1,500 Ma |
Neo-Archaic Duration: 300 Ma |
2500 ⬍ 2800 |
||
Mesoarchean Duration: 400 Ma |
2800 ⬍ 3200 |
|||
Paleoarchean Duration: 400 Ma |
3200 ⬍ 3600 |
|||
Eoarchic Duration: 400 Ma |
3600 ⬍ 4000 |
|||
Hadaikum Duration: 600 Ma |
4000 ⬍ 4600 |
The Paleoproterozoic Glaciation or Huronian Ice Age (after the Huron Sea on the border between the USA and Canada) began 2.4 billion years ago and was the longest ice age in the history of the earth with a duration of about 300 million years. Geological evidence of the climate from this era can be found in North America, Scandinavia, India and southern Africa, among others, and point to a global cold snap. A number of studies also assume at least one snowball earth event that resulted in complete ice cover of the earth including the equatorial zone and the oceans.
The climate mechanisms of the Paleoproterozoic glaciation are only incompletely documented, as there is no reliable knowledge about the type and scope of the plate tectonic processes at that time such as mountain formation or the size ratio between the oceanic and continental crust . Also, due to the large time gap, the alternation of different cold and warm periods typical for the later Ice Ages is hardly detectable. However, general acceptance is the hypothesis that the glacial climate of the early Paläoproterozoikums closely with the Grand oxygen disaster (English Great Oxygenation Event could be linked) before 2.4 billion years ago.
At the beginning of the Paleoproterozoic , the terrestrial atmosphere had a relatively high methane concentration, but only small traces of free oxygen. Although cyanobacteria produced large amounts of O 2 as a "waste product" of their metabolism by means of oxygenic photosynthesis more than 3 billion years ago, the oxygen was converted into trivalent iron ions during the oxidation of organic compounds, hydrogen sulfide and divalent iron ions Fe 2+. Ions Fe 3+ completely consumed. This process was directly related to the formation of bands of iron ore linked (English Banded Iron formation ), an iron-containing sedimentary rock that was mainly deposited in Precambrian and that could not be formed under the present conditions.
After this intensive oxidation phase was over, an excess of free oxygen occurred, which began to accumulate in both the atmosphere and the ocean. The latter led to the mass extinction of obligate anaerobic organisms in the previously oxygen-free biotopes, almost all of which fell victim to the toxic effects of oxygen. This event is one of the greatest crises in the history of life, nevertheless it opened up new paths for evolution with regard to a more efficient use of the energy metabolism in many forms of life.
With the help of UV radiation, the oxygen in the atmosphere oxidized most of the methane deposits to carbon dioxide and water. Since methane has a significantly greater global warming potential than CO 2 , the result was an abrupt climate change , and temperatures dropped to ice-age levels for 300 million years. The atmospheric oxygen content was subject to only insignificant fluctuations until the Neoproterozoic and mostly fluctuated between 2 and 3 percent. It was not until the beginning of the Cambrian , around 540 million years ago, that a significant increase gradually occurred.
The snowball-earth hypothesis
The pronounced Ice Age cycles in the late Proterozoic left a large number of clear traces on almost all continents. A precise chronological classification of these cold phases was fraught with great uncertainty until recently and has only recently been determined more precisely. The following four neoproterozoic glacial events are generally recognized:
- Kaigas Ice Age , about 740 million years ago (probably only regional)
- Sturtic Ice Age , about 717 to 660 million years ago (global glaciation, possibly in several phases)
- Marino Ice Age , about 640 to 635 million years ago (global glaciation)
- Gaskiers Ice Age , about 580 million years ago (probably only regional, duration less than 1 million years)
Some evidence suggests that a series of snowball earth events occurred during the Sturtischen and the Marino Ice Age , with a complete icing of all land masses and oceans over a period of several million years each. An essential support of this relatively young hypothesis, which has been extensively tested since the 1990s, are the mighty glacial deposits that can be found in many places on earth and that, according to paleomagnetic studies, have partially formed in the immediate vicinity of the equator. A cyclical climatic constellation, including plate tectonics, weathering, erosion and carbon dioxide binding, is assumed to be a possible cause of the recurring icing processes, which was repeated until one or more of its components (e.g. due to continental shifts) left the cycle. In addition, self-reinforcing ice-albedo feedback could have promoted and accelerated the global cooling down to -50 ° C. The natural carbon cycle almost came to a standstill in this way, and biomass production in the oceans sank to a minimum. This only changed when the unused atmospheric reservoir of volcanic CO 2 emissions reached an extremely high threshold (possibly in the region of 100,000 ppm), which tipped the permafrost climate and triggered a global thaw. Within about 40,000 years, the earth changed from a deep-frozen snowball under chaotic environmental conditions ( heavy rain , hurricanes , extremely rapid sea level rise of several hundred meters) into a "super greenhouse" with tropical temperatures of at least 40 ° C.
Although the striking image of the earth as a giant snowball gained a certain popularity and also circulated outside of the specialist literature, the number of voices contradicting the hypothesis increased. In the opinion of the critics, some of the data sets determined are not sufficiently verified or can be interpreted ambiguously, which among other things led to the alternative design of a “mud ball earth” . Above all, however, complete freezing over millions of years would have prevented the photosynthesis of oxygen-producing organisms and led to the extinction of almost all marine life. Like most of the details of the snowball scenario, this criticism is a subject of controversial scientific discussion. The only thing that is certain at present is that the snowball-earth hypothesis has neither been confirmed nor refuted and therefore requires further examination.
The Gaskiers Ice Age was followed by a series of smaller, spatially limited glaciation phases, the more detailed exploration of which is only just beginning with regard to duration and temporal classification. During the entire Ediacarium and into the early Paleozoic , climatic conditions evidently fluctuated strongly, indicating increased plate tectonic activity with permanent volcanism. Overall, there was a trend towards global warming, and the oxygen content rose significantly , first in the oceans and, from the Cambrian, also in the atmosphere. This increase is considered to be a prerequisite for the appearance of the first complex eukaryotes and for the development of the Ediacaran fauna .
Mass extinction in the Paleozoic
According to the Australian marine biologist John Veron , mass extinction events can be classified into two different categories: namely whether they were caused by a direct contribution to the carbon cycle or whether they occurred independently of it. A biological crisis caused by an extremely rapid increase or decrease in greenhouse gas concentration, for example, would fall under the first category, while impact disasters or a lowering of the sea level caused by plate tectonics would be assigned to the second group. Comprehensive analyzes of known mass extinctions have recently led to a deeper understanding of the mechanisms and relationships between these events. The specialist literature on this topic has increased almost tenfold since the 1980s and is increasingly taking interdisciplinary research into account. This led to the realization that mass extinctions do not necessarily have to be linked to long-term geological processes, but have often taken a catastrophic and time-limited course. In addition, there is a growing body of evidence supporting the assumption that almost all known mass extinctions in the history of the earth or a rapid reduction in biodiversity were directly linked to serious climate change events and their consequences.
Cambrian
The Paleozoic era began with the Cambrian geological system 541 million years ago . During the Cambrian Explosion that followed immediately , the representatives of almost all animal phyla that existed today, including their morphological blueprints, which have not changed since then , emerged within only 5 to 10 million years . This rapid evolutionary development is very likely to be closely related to a profound change in climatic and environmental conditions. At the beginning of the Cambrian, the “short-lived” supercontinent of Pannotia broke into several parts after only 50 million years with a strong increase in plate tectonic activities. This released considerable amounts of carbon dioxide and other volcanic gases into the atmosphere, and also new climatic zones and ocean currents formed.
From a climatic point of view, the Cambrian was a period with sometimes extremely increased volcanism, with average temperatures around 20 ° C and an atmospheric CO 2 concentration of over 5000 ppm. These factors had a lasting influence on the chemical composition of the sea water, so that the oceanic communities often reached their biological limits due to sulfur dioxide input, oxygen shortage and acidification and the associated drop in the pH value. In addition, recent studies have shown that there was significant carbon cycle disruption in the late Cambrian .
The rapid increase in biodiversity in the course of the Cambrian Explosion also led to a rapid increase in the so-called background extinction , which reached a very high level as a permanent feature of biological evolution in the first half of the Paleozoic, and especially during the Cambrian . It is therefore difficult to distinguish between the natural exchange of species and mass extinction, especially since some Cambrian strata contain only sparse material with regard to fossil records. Two large and several small extinction waves are assumed for the Cambrian , about the duration and intensity of which little is known. However, a Cambrian mass extinction 510 million years ago was recently reconstructed, with large-scale volcanic eruptions and the formation of anoxic zones in the oceans apparently contributing to at least 50 percent of all marine species becoming extinct.
Ordovician
The Andean-Sahara Ice Age began around 460 million years ago in the Upper Ordovician , reached its peak at the last Ordovician stage of the Hirnantium and ended in the Silurian 430 million years ago. The movement of the large continent of Gondwana over the South Pole can be reconstructed in chronological order using ice age deposits . The core area of the glaciation lay in today's Sahara 450 to 440 million years ago, then migrated westwards towards South America (Brazil and the lower Amazon region) and expanded 430 million years ago to the region of the then non-existent Andes chain .
A special feature of the Andes-Sahara Ice Age is that, regardless of a CO 2 level of initially 4000 to 5000 ppm, a long-term global cooling set in. The explanations given are the continental cover of the Antarctic, rapid carbon dioxide binding and an increased weathering effect due to the increasing spread of land vegetation as well as a possibly greater fluctuation range of the earth's axis. In addition to the shorter day length of 21.5 hours, which, according to model simulations, also represented a cooling factor under the conditions at that time, the solar radiation that has been reduced by 4.5 percent compared to the present must be taken into account (solar constant in the Ordovician 1306 W / m², currently 1367 W / m²).
At the height of the glaciation phase, 443 million years ago, one of the most momentous mass extinctions in the history of the earth occurred. The estimates of the extinction rate of the affected species vary considerably and amount to up to 85 percent. The reason is usually given as a combination of various influences such as general cooling, a drop in sea level and strong volcanism.
According to some recent studies, not only the glacial climate and the associated lowering of the sea level (with a decline in marine shallow water biotopes) are the primary extinction factors, but also geochemical changes such as the extensive release of toxic heavy metals or the extensive reduction of trace elements played a serious role. The concentration of the vital trace element selenium at the transition from Ordovician to Silurian apparently only reached a fraction of the current level and was also at the minimum in some later mass extinctions. Alternatively, an extraterrestrial cause in the form of a gamma-ray flash has been suggested on various occasions . Although the rapid decimation of the organisms that inhabit the upper sea zones agrees with the radiation hypothesis, there is no further factual evidence. Section 2.2
Devon
The Devonian is characterized by a double biological crisis: the Kellwasser event at the Frasnian-Famennian boundary 372 million years ago and on the threshold from the Upper Devonian to the Carboniferous 13 million years later the similarly pronounced Hangenberg extinction . 70 percent of all marine life forms were affected by the events, especially the fauna groups of shallow tropical seas, in which anoxic conditions occurred periodically . The biodiversity of phytoplankton decreased so much that the original biodiversity was only reached again in the Jura (phytoplankton blackout) .
According to the current state of research, the two Devonian mass extinctions are based on a combination of various factors, including a brief alternation of several warm and cold phases. These were possibly caused by the cyclical changes in the earth's orbit and were decisively increased by the reduced buffer effect of the atmospheric CO 2 . It is very likely that the falling carbon dioxide content, along with abrupt sea level fluctuations and volcanic influences, played an important role in the crisis time of the Kellwasser and Hangenberg events . At the beginning of the Devonian Mountains, it was far beyond the 1000 ppm threshold, and considerable amounts of CO 2 were withdrawn from the atmosphere and stored in the gradually expanding forest vegetation. It is possible that some large impact events such as the Australian Woodleigh or the Alamo impact in today's Nevada were directly involved in the extinction waves and the climatic changes in the Upper Devonian . The fossil-poor faunal situation (Romer gap, English Romer's gap ), which has long been considered puzzling and named after the paleontologist Alfred Romer (1894–1973) and extends over 15 million years into the Lower Carboniferous , could be directly related to the previous mass extinctions .
The carbonic tropical forest collapse
In popular representations, the "Carboniferous" is the Carboniferous often a synonym for hot, humid climates and tropical jungles, covered all the continents. This picture only partially corresponds to the circumstances at that time. The tendency , which has existed since the Devonian , towards the formation of extensive forest and marsh landscapes, continued into the Upper Carboniferous , with club moss plants like the shed trees reaching a size of 40 meters. But even at the height of the carbonic vegetation expansion there were large arid areas with a desert-like character in the continental central areas. The global temperature was 20 ° C at the beginning of the Carboniferous , but decreased steadily over the duration of the period and with an average value of 14 ° C was roughly the current level. This downward trend is closely related to the Permocarbon Ice Age ( Karoo Ice Age ), which was heralded in the Lower Carboniferous with the beginning of glaciation of the land masses lying within the southern polar circle.
Parallel to the Caledonian mountain formation , the two continents Laurentia (North America) and Baltica (Northern Europe and Russian table ) already merged to form the new major continent Laurussia in the Silurian , while in the southern hemisphere the major continent Gondwana dominated , reaching into the Antarctic regions . In the course of the Devonian , Laurussia and Gondwana moved closer and closer together in order to unite in the Upper Carboniferous to form the supercontinent Pangea . The collision of the two continental plates caused on the one hand the formation of the Variscan high mountains and on the other hand interrupted the water and heat exchange of the equatorial ocean currents as a huge continental barrier. As a result of the restricted oceanic circulation, the cooling trend prevailing in the Carboniferous increased . The carbon dioxide released by the plate-tectonic process of the Variscan mountainous formation was quickly withdrawn from the atmosphere due to accelerated weathering processes and above all through the biomass production of the carbonic flora with subsequent coalification . As a result, atmospheric carbon dioxide fell below 400 ppm for the first time in geological history towards the end of the epoch and continued to decrease at the beginning of the Permian . In contrast, the oxygen content reached the record level of 35 percent. The high O 2 concentration made it possible for various arthropods to grow in size, such as the giant dragonfly Meganeura or the millipede Arthropleura , but posed the risk of large-scale forest fires.
In the last million years of carbon there was a relatively rapid change in different climatic conditions , which were largely controlled by the cyclical changes in the Earth's orbit parameters , with strongly fluctuating CO 2 concentrations from 150 to 700 ppm and corresponding fluctuations in sea level. Taking into account the 3 percent reduction in solar radiation compared to today , the global average temperatures reached 12 to 14 ° C during a warm phase and rose only slightly above freezing point in a glacial period.
305 million years ago, severe climatic cuts and increasing drought caused the carboniferous rainforest collapse in the Kasimovium to collapse, resulting in the first mass extinction of plants. The tropical forests were decimated to a few islands of vegetation within a geologically very short period of time, and many wetlands and marshlands also disappeared. Many of the amphibians of the time , most of which became extinct, were particularly hard hit by the loss of these habitats .
In the late Carboniferous and during the transition to the Permian , new forest biotopes emerged that were adapted to an arid climate with seasonal temperature fluctuations, such as the cold-resistant and deciduous Glossopteris flora in the southern regions of Gondwana , which developed into the predominant plant type there. In the earliest Permian, the atmospheric CO 2 levels fell briefly to a level of 100 ppm or even below, according to a 2017 study. If this assumption is confirmed, the earth system would then move into the immediate vicinity of the tipping point that would have brought the planet into the climatic state of global icing, comparable to the snowball earth events in the Neoproterozoic .
The Permian Triassic Crisis
The first Permian ecological crisis occurred 262 million years ago shortly after the end of the Permocarbon Ice Age in Capitanium . The reduction in fauna in tropical regions, which has been known for a long time from this epoch, seems, according to recent studies, to have been a global crisis that culminated in mass extinction. The cause is assumed to be an extensive carbon and sulfur dioxide entry into the oceans with the formation of anoxic zones and strong acidification of the seawater. There may be a connection with the simultaneous volcanic activities of the Emeishan Trapp in what is now southern China.
Almost 10 million years later, at the Permian-Triassic border, what is probably the most extensive mass extinction in the history of the earth happened , accompanied by a rapid climate change in geological terms with serious effects on the biodiversity of flora and fauna. The main cause favored are violent volcanic activities with extremely high emissions in the area of today's Siberia , which lasted several hundred thousand years and covered seven million km² with basalt (which corresponds to about 80 percent of the area of Australia). By the beginning of the Triassic , 96 percent of all marine life and 75 percent of land life were extinct. Numerous species of insects were also affected - a unique event to this day .

The reconstruction of the events suggests several warming phases. The igneous activities of the Siberian Trapp are estimated to have released more than 100 trillion tons of carbon dioxide over a long period of time, increasing the global temperature by 5 ° C in a relatively short time. The Siberian Trapp also emitted considerable amounts of hydrogen chloride and sulfur dioxide , which, as sulfuric acid in rainwater, damaged both oceanic and continental biotopes. Analyzes of the 18 O / 16 O isotopes from this period document a rapid warming of the upper sea layers by at least 8 ° C. The rise in temperature not only favored the formation and spread of anoxic zones , but also increasingly acidified the oceans. The rapid drop in pH is considered to be one of the main causes of the almost complete disappearance of oceanic life forms. Another effect occurred as a result of the destabilization of the methane hydrate deposits in the oceanic shelf areas, as a result of which large amounts of methane diffused into the atmosphere. The additional global warming potential corresponded to a CO 2 equivalent value of over 3000 ppm and led to a temperature jump of another 5 ° C in the next phase. The oxygen content sank to a minimum in the range of 10 to 15 percent due to the meanwhile strongly decimated vegetation cover.
Another possible cause of the destabilization of the biosphere is the proliferation of marine protozoa, which released their metabolic products into the atmosphere in the form of hydrogen sulfide or methane. Until recently, around 200,000 years was considered a realistic value for the duration of the Permian Triassic mass extinction. According to a study from 2014, this period is reduced to about 60,000 years (± 48,000 years). A paper published in 2018, on the other hand, postulates a narrow time window of a few millennia to a maximum of 30,000 years for the biological crisis on the basis of new findings. The global collapse of almost all ecosystems could be dated to the lowest Triassic 251.94 million years ago with the help of precise detection methods. The current data suggests a catastrophe that will break out suddenly according to geological standards and almost excludes gradual environmental changes.
There are currently several hypotheses about the trigger (s) of the Permian Triassic Crisis , including the assumption of a large meteorite impact. In 2006, a gravity anomaly was detected using satellite data in the southern polar Wilkesland region . Radar images provided evidence of the existence of a 480 km large impact crater deep under the Antarctic ice sheet with a presumed age of 250 million years. This would make the Wilkesland crater the largest known impact in the history of the earth, whose destructive potential would have significantly exceeded that of the Chicxulub meteorite on the Cretaceous-Paleogene border . However, as long as there is no direct evidence, for example through deep geological drilling , the Wilkesland impact is initially considered a hypothetical event.
Another major mass extinction took place before 201,500,000 years at the Triassic-Jurassic boundary instead (English Triassic-Jurassic Extinction Event ). Megavolcanism is also assumed to be the primary cause for this event (Central Atlantic Magmatic Province) , with climatic effects comparable to the eruptions of the Siberian Trapps . In this case too, many groups of fauna disappeared within a few 10,000 years.
Oceanic anoxic events
Oceanic anoxic events (English Anoxic Oceanic Events , abbreviated to OAE ) in the history of the earth were based on an oxygen deficit (below 2 mg / l), especially in tropical shallow seas. Only the top mixed water layer was excluded from this. To the best of our knowledge, the last OAEs occurred during the Paleocene-Eocene Temperature Maximum (PETM) more than 50 million years ago. In the Mesozoic, and most likely also in the Paleozoic, OAEs were often linked to a series of extinction events. A significant anoxic event is usually based on several prerequisites:
- an atmospheric carbon dioxide concentration well above 1000 ppm
- a worldwide subtropical to tropical climate and simultaneous warming of the oceans with correspondingly reduced oxygen binding (from the Upper Ordovician , however, an OAE during a global cooling is documented)
- a stalled deep water circulation between the polar regions and the equator
- the over-fertilization of the seas with continental weathering products due to rapid erosion processes
There are currently several hundred hypoxic or anoxic zones (often called dead zones in English ) with a total area of around 250,000 km², such as the Baltic Sea , the northern Adriatic or the Mississippi delta in the Gulf of Mexico . For the Cretaceous OAE 2 it is assumed that 5 percent of the total seawater volume was oxygen-free, although the anoxic milieu may have been considerably more extensive in the course of the great mass extinction during the Permian Triassic crisis .
Pronounced anoxic events lasted at least several hundred thousand years, but in exceptional cases could last more than 2 million years. Regular side effects of an oxygen shortage were an algal bloom that turned the seawater green and the bacterial production of large amounts of hydrogen sulfide (H 2 S), the smell of rotten eggs of which is likely to have spread across large coastal areas. An indicator for the determination of anoxic events are the marine black slate sediments that are formed in an oxygen-free environment , which are formed from digested sludge at the bottom of the ocean and occur in clusters in Cretaceous deposits. Since the age of the oceanic crust is limited, the search for OAEs more than 150 to 200 million years ago is mostly limited to sedimentary layers that are now on the mainland today.
Cretaceous-Paleogene boundary (formerly Cretaceous-Tertiary boundary)
In June 1980, the research team led by the physicist and Nobel Prize winner Luis Walter Alvarez and his son, the geologist Walter Alvarez , published the discovery of an iridium anomaly at the Cretaceous-Paleogene boundary . The resulting assumption of a large asteroid impact that led to the extinction of dinosaurs , among others , was the beginning of a long discussion about the pros and cons of the hypothesis presented by father and son Alvarez.
In search of the possible impact point of the impactor in 1991 on the Mexican Yucatán peninsula , a 180 km large crater was found below the village of Chicxulub Puerto and was covered by younger sediments . This did not end the scientific controversy about the so-called Chicxulub impact . Even if the crater corresponded to the requirements of a “ global killer ” in terms of age and size , several counter-hypotheses were put forward, including that it was not the impact, but the magmatic eruption of the Indian Dekkan Trapps that had accelerated mass extinction at the CP border . In addition, sediment studies appeared to confirm that the Chicxulub crater was formed 300,000 years before the actual KP boundary layer.
This "pre-dating" met with criticism from the start and is classified as very unlikely in view of the latest research results. The application of refined dating methods and analysis techniques with very low tolerance ranges led to the result that the impact event and the KP boundary layer coincide precisely in time. The impact winter following the impact is now considered factually secured. Until recently, there was largely agreement in science that at the end of the Cretaceous, biodiversity and the stability of ecosystems were on the decline. There are now increasing indications that the ecological situation in the late Maastrichtian was more stable than assumed for a long time. Thus it was left to the impact to mark the end of the Mesozoic fauna. More recent studies have therefore come to the conclusion that the Chicxulub impact alone triggered the mass extinction on the Cretaceous-Paleogene border.
The currently most likely scenario assumes that 66.04 million years ago (± 32,000 years) an approximately 10 km large asteroid with a speed of about 20 km / s (72,000 km / h) in the area of today's Gulf of Mexico in one tropical shallow sea detonated. The impactor evaporated within a second, but due to the force of the explosion, which was likely to be heard all over the globe, it hurled several thousand cubic kilometers of carbonate and evaporite rock over long distances as a glowing ejecta into the stratosphere . In addition to the immediate effects of the impact such as megatsunamis , a supersonic pressure wave and earthquakes with a magnitude of 11 or 12, wildfires occurred around the world, the extent and duration of which is currently still being discussed. Within a few days, a large amount of soot and dust particles were distributed throughout the atmosphere, absorbing sunlight for months and causing a global drop in temperature. An additional cooling factor was possibly an atmospheric layer of sulfuric acid - aerosols , which, according to a recent study, could have caused a temperature drop of 26 ° C and played a decisive role in keeping the global average temperature below freezing for a few years , with dramatic consequences for the whole Biosphere.
The oceanic and mainland ecosystems were equally affected by this crisis. 75 percent of the species fell victim to mass extinction within a period that cannot be precisely determined, including not only the dinosaurs, but also the ammonites , almost all calciferous foraminifera and, to a large extent, the birds. After a cold phase presumably lasting several decades, rapid warming began, leading to heat stress , due to billions of tons of carbon dioxide released by the impact as a result of the evaporation of oceanic floors. The duration of the extreme greenhouse effect is estimated at around 50,000 years before the climate probably only finally stabilized after several hundred thousand years.
A hypothesis presented by several well-known geoscientists in April 2015 assumes that the long “smoldering” Dekkan-Trapp in what is now West India recorded a considerable increase in its activity due to the impact energy of 3 × 10 23 joules and the tectonic shock waves triggered by it . According to this hypothesis, the short-term discharge of 70 percent of all Dekkan-Trapp flood basalts is due to the Chicxulub impact. In addition, extensive magma outflows could have occurred in the area of the plate tectonic boundaries or fracture zones on the sea floor. The previously neglected possibility of a direct connection between asteroid impact and increased flood basalt volcanism is currently being discussed intensively in the geosciences. A study published in 2020 comes to the conclusion that the likely impact angle of the impactor of 45 to 60 degrees showed the maximum destructive effect under all impact scenarios.
The New Earth Age (Cenozoic) Climate
Arathem | system | series | Age ( mya ) |
---|---|---|---|
K ä n o z o i k u m |
quaternary | Holocene | 0 ⬍ 0.0117 |
Pleistocene | 0.0117 ⬍ 2,588 |
||
Neogene | Pliocene | 2,588 ⬍ 5.333 |
|
Miocene | 5.333 ⬍ 23.03 |
||
Paleogene | Oligocene | 23.03 ⬍ 33.9 |
|
Eocene | 33.9 ⬍ 56 |
||
Paleocene | 56 ⬍ 66 |
||
earlier | earlier | earlier |
Paleogene
With the Cenozoic Era (largely identical to the earlier Tertiary ), the Earth's Modern Age began 66 million years ago. At the beginning of the Paleogene, with the connected land areas of Australia , Antarctica and South America, there was still an extensive remainder of the great continent of Gondwana . Its final disintegration happened 45 million years ago when Australia broke away from Antarctica and South America followed this trend a little later. This established a system of ocean currents in the southern hemisphere that was already very similar to the current one.
In the northern hemisphere in connection with the formation and expansion of the created North Atlantic , the North Atlantic Magmatic Province United (English North Atlantic Igneous Province ). The igneous or volcanic processes began as early as the lower Paleocene (about 64 to 63 mya), extended in a much weaker form into the early Miocene and recorded several increased activity cycles, with intrusive and effusive phases alternating along the diverging plate edges. The flood basalts rising from the earth's mantle had an area of approximately 1.3 to 1.5 million km² and covered parts of Greenland, Iceland, Norway, Ireland and Scotland.
The paleogene with the three series Paleocene , Eocene and Oligocene is important in several ways. On the one hand, some of the developments that began during this period continue to have an impact on the geological present, and on the other hand, research focuses on a number of events from that time due to distinctive features. From a climatological perspective, the Paleocene / Eocene temperature maximum is of particular interest, as it is a striking example of an abrupt climate change and could therefore show parallels to current global warming and its consequences.
The table below shows a chronological breakdown of those environmental changes that occurred in relatively close succession between 55 and 33 million years ago.
designation | Beginning | Duration | Impact of the event |
---|---|---|---|
Paleocene / Eocene temperature maximum (PETM) | 55.8 million years ago | Max. 200,000 years | Extremely high and rapid global warming including the oceans |
Eocene Thermal Maximum 2 (ETM-2) | 53.7 million years ago | Max. 200,000 years | Significant global warming |
Azolla event | 49.0 million years ago | 800,000 years | Mass reproduction of the swimming fern Azolla in the Arctic Ocean, decrease in the atmospheric CO 2 concentration |
Chesapeake Bay Impact (North America) | 35.5 (± 0.3) million years ago | probably short-term cooling | Differing information on the size of the crater (40 to 90 km), influence on the climate therefore uncertain, Megatsunami |
Popigai impact (Siberia) | 35.7 (33.7?) million years ago | Possibly longer lasting impact consequences | Crater size 90 to 100 km, probably temperature drop with impact winter |
Eocene-Oligocene mass extinction ( Grande Coupure ) | about 33.5 million years ago | approx. 300,000 years | Global cooling and extinction event followed by a change of fauna |
- Paleocene / Eocene maximum temperature (PETM). After the caesura of mass extinction on the Cretaceous-Paleogene border, the Paleocene initially had a dry, relatively temperate climate that became increasingly tropical and humid towards the end of the epoch. At the transition to the Eocene , the earth warmed by about 4 ° C in equatorial areas and up to 10 ° C at higher latitudes over a likely 4,000 year period, with annual carbon inputs in the order of 0.6 to 1.1 petagrams parallel to that coupled heating proceeded. Several studies show that the oceans stored significant amounts of heat during the PETM . For subpolar waters (western Siberian Sea) 27 ° C were determined, and sediment cores from the coastal region off Tanzania show a temperature maximum of around 40 ° C. This led to a rapid acidification of the seas and the emergence of anoxic milieus with lasting consequences for the oceanic biotopes. The exact cause of the PETM is still unknown, although it is widely suspected that released methane hydrate accelerated and intensified the event significantly. Although the climatic emergency of the PETM was short-lived according to geological standards, it had a lasting impact on the biodiversity and paleoecology of the entire planet. More recent studies seem to show that climate sensitivity increases accordingly during a global warming phase . A range of 3.7 to 6.5 ° C is estimated as the most likely value for the PETM.
- The thermal anomaly of the Eocene Thermal Maximum 2 was similar in its duration and effects to the better researched PETM , but could have reached a slightly lower temperature level than this. From the time 53.6 to 52.8 million years ago there are indications of three other and less pronounced heat anomalies, the scientific investigation of which, however, is only just beginning. Information on the atmospheric CO 2 concentration in the Lower Eocene is fraught with great uncertainties due to the serious and short-term climatic fluctuations. A study published in 2016, based on a precision measurement including the stable boron isotope δ 11 B (Delta-B-11), postulates a carbon dioxide content of around 1,400 ppm for the time of the Eocene climatic optimum .
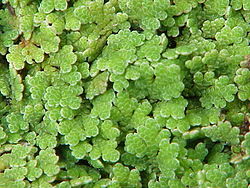
- The Azolla event was a turning point in the climatic history of the Cenozoic and has had far-reaching consequences up to the present day. The family of salviniaceae scoring Azolla ( Azolla ) can store large amounts of nitrogen and carbon dioxide and massively proliferate under favorable conditions. This case occurred due to a series of special circumstances when Azolla "colonized" the then Arctic Ocean over an area of 4 million km² 49 million years ago . Since in the Eocene the Arctic Sea was isolated from other oceanic currents and, due to a lack of mixing, became a kind of stagnant water , a thin but nutrient-rich freshwater layer could have formed on its surface due to rain and the entry of the rivers, which enabled the explosive growth of Azolla . The floating vegetation island of the algae ferns existed for several hundred thousand years and during this time brought about a relatively rapid reduction in CO 2 to around 1,000 to 650 ppm through the uptake of carbon dioxide and its integration into sedimentation processes in combination with a few other factors . This began a gradual global cooling that eventually passed into the Cenozoic Ice Age .
- The Chesapeake Bay crater on the east coast of the USA is representative of around a dozen impact craters with a diameter of well over 10 km that were formed during the paleogene . With a focus on the Eocene , a series of impact events occurred at short intervals, although meteorite hits in the oceans have so far hardly been documented and are therefore likely to have a high number of unreported cases. In contrast to this, the Ries event 14.6 million years ago only shows a larger impact from the entire Neogene . Similar to the Chesapeake Bay impact, the dimensions of which are still being discussed, there is largely uncertainty about similar impact events from this epoch with regard to their impact on the environment and climate. In the more recent specialist literature, this problem is discussed in increasing detail with the help of extensive data material.
- The Popigai impact in northern Siberia left a 90 to 100 km large crater and, with the Chicxulub impact and the Manicouagan event from the Triassic, is one of the largest scientifically proven impact disasters in the Phanerozoic . Depending on the structure and composition of the meteorite, its size should be 5 to 8 km. The age of the crater has so far been given as 35.7 million years, a more recent date gives 33.7 million years as the most likely value. Thereafter, the Popigai impact would coincide with the extinction of species of the Grande Coupure on the border between Eocene and Oligocene (English Eocene-Oligocene Extinction Event ). In addition to the sudden extinction of 60 percent of European mammal species, the abrupt cooling of the oceans around 34 million years ago could also be linked to one or more impacts. However, this possibility is rejected by other studies and rated as low. Corresponding analyzes are made more difficult by the fact that of the approximately 180 larger terrestrial impact structures, only a dozen of them know the exact time they were formed with sufficient certainty.
Neogene
The change from warm to cold-age climates that began with the Azolla event (often referred to internationally as "transition from greenhouse to icehouse conditions") led to the first glaciations in Antarctica during the Eocene-Oligocene transition around 34 million years ago. During this time, the C 4 plants adapted to arid conditions began to spread (especially grasses ), which also require significantly less carbon dioxide for photosynthesis than C 3 plants . The global cooling trend, coupled with a gradual reduction in atmospheric carbon dioxide, was not linear, but was interrupted first by a warming phase in the late Oligocene and then by a climatic optimum in the Miocene 17 to 15 million years ago. At the height of the Miocene climatic optimum, the CO 2 content rose briefly from 350 ppm at the beginning of the Miocene to 500 ppm (according to other sources to over 600 ppm), and the average annual temperature for Central Europe rose to 22 ° C.
In the course of global warming, in which the massive CO 2 emissions from the Columbia Plateau basalt were likely to play a major role, the forest habitats were pushed back, and steppes and grasslands took their place. At the same time, the Antarctic glaciers of that time lost some of their mass, but without melting completely. Simulations including the CO 2 level at the time indicate that the core areas of the East Antarctic Ice Sheet were hardly affected by the warming in the Middle Miocene. Under the influence of strong erosion and weathering processes, the CO 2 concentration sank again below 400 ppm towards the end of the optimum 14.8 million years ago, and with an abrupt temperature drop of 7 ° C in Central Europe, a cooler climate phase began globally with a renewed increase in the Antarctic inland freezing. However, 14 to 12.8 million years ago, the temperatures in Antarctica were still 25 ° C to 30 ° C above current levels before the region was hit by a cold snap.
In the further course of the Miocene, large parts of Europe had a relatively mild and dry climate. However, in the period from 10.2 to 9.8 million years ago and then from 9.0 to 8.5 million years ago, two "laundry room phases" developed in which the climate became significantly more subtropical and more humid (with annual rainfall of some over 1500 mm). The main causes of these warming peaks are suspected to be large-scale shifts in oceanic circulation patterns in the area of the Atlantic . The Miocene is regarded as a “model case” for understanding rapid climate change events and for the long-term interaction of silicate weathering, erosion, carbon sequestration and atmospheric CO 2 .
The Neogene and its changeable climate history also developed into a field of research for determining climate sensitivity . This is about the scientifically and climate-politically relevant question of how high global warming would be if the pre-industrial CO 2 value doubled from 280 ppm to 560 ppm. Laboratory measurements, excluding all external factors, resulted in a temperature increase of 1.2 ° C; when quick-acting feedbacks are included (e.g. water vapor, ice-albedo and aerosol feedback), a climate sensitivity of 3 ° C is currently most likely. In addition, an attempt is made to determine the climate sensitivity based on various climatic conditions , taking into account all short-term and long-term feedback mechanisms over the duration of geological periods. According to this, the so-called Earth system climate sensitivity is in the range of 4 to 6 ° C.

In the Early and Middle Pliocene , global temperatures were around 2.5 to 4 ° C above pre-industrial levels, with sea levels around 20 meters higher than current levels, and CO 2 levels fluctuating between 365 and 415 ppm over the same period. A geologically significant event with far-reaching climatic effects was the repeated drying out of the Mediterranean and its temporary transformation into a salt desert ( Messinian salinity crisis ) on the border between Miocene and Pliocene 6 to 5 million years ago.
Global consequences in terms of the intensifying cooling process at the transition from the Pliocene to the Quaternary had the formation of the Isthmus of Panama when the Pacific plate collided with the Caribbean plate , whereby the connection between the Pacific Ocean and the Atlantic was interrupted. It is generally assumed that the water exchange between the two oceans decreased significantly for the first time more than 3 million years ago and finally came to a standstill 2.76 million years ago with the complete closure of the isthmus. A study from 2015, however, came to the conclusion that the isthmus could have formed as early as the Middle Miocene around 15 million years ago. However, a study published in August 2016 based on geological, palaeontological and molecular biological findings confirmed the previous assumptions. The final closure of the land bridge immediately resulted in the emergence of the Gulf Stream , which from then on transported seawater from tropical latitudes to the north, increasing the humidity in the Arctic and thus the potential for precipitation. However, the initial warming of the North Atlantic regions quickly turned into the climatic state of the Quaternary Ice Age when the inclination angle of the earth's axis approached a new minimum. With the trend towards snowy winters and cooler summer months in the northern hemisphere, a cold phase intensified by ice-albedo feedback began, which, interrupted by several interglacials , shaped the global climate for 2.7 million years until the Holocene .
quaternary

Due to its young and very extensive deposits, the Quaternary Ice Age has been the most intensively researched epoch in the history of the earth for well over a hundred years, with a wealth of geological, paleontological and climatic evidence.
- A summary of the last 30 million years can be found under Cenozoic Ice Age , the keyword Quaternary research provides an interdisciplinary inventory, while the articles Pleistocene and Quaternary focus on paleontology (Ice Age fauna) and the geological-stratigraphic classification.
- Information on abrupt climate fluctuations and climate change events in connection with the Quaternary Ice Age can be found in the Younger Dryas Period , the Dansgaard-Oeschger Event and the Heinrich Event .
- Detailed descriptions of individual warm periods (interglacials) within the Quaternary Ice Age are contained in the articles Waal Warm Period , Cromer Complex , Holstein Warm Period and Eem Warm Period . The same applies to the complex of the northern European / northern German cold ages Elbe , Elster , Saale and Weichsel as well as to their alpine equivalents Günz , Mindel , Riss and Würm .
- The youngest section of the Quaternary , the Holocene , is identical to the geological present and is considered a field of research in historical climatology , especially with regard to short-term weather anomalies and the influence of climate on human cultural development. In addition, historical climatology also has interfaces to several specialist areas of archeology , such as glacier , coastal and geoarchaeology . These factors are also covered in the relevant sections of the Climate History article .
The future of the earth's climate
Possible duration of anthropogenic global warming

The Holocene as the youngest chapter in the earth's history began after the end of the last cold phase , the Quaternary Ice Age , 11,700 years ago. This period includes all known advanced civilizations as well as the entire historically documented human history including modern civilization. During the Holocene there was a consistently stable global climate with a temperature corridor of approximately ± 0.5 ° C. The absence of geophysical, biological and climatic crises is seen as a guarantee that apart from regionally limited cuts, a relatively uniform cultural and technological development of human societies could take place.
Sediment cores from the deep sea show a Holocene climatic optimum around 8000 to 6000 years ago, the temperature of which was only exceeded in the 21st century. Due to the decrease in solar radiation in northern latitudes during the summer maximum, there has since been a slight decrease in temperature of 0.1 ° C per millennium. This cooling trend, coupled with the periodicity of the Milanković cycles , would normally lead to the interglacial of the Holocene being followed by a new glacial period in around 30,000 years. Whether this event occurs as predicted depends, among other things, on future climatic developments in connection with the release of anthropogenic and natural greenhouse gases, whereby in addition to the increase in CO 2 , increasing outgassing of methane from oceanic methane hydrate deposits is observed. According to the emission scenarios of the Intergovernmental Panel on Climate Change (IPCC) in the current Fifth Assessment Report , the global average temperature could in the worst case increase by more than 4 ° C by the end of the 21st century. While a warming from the current 1 ° C to 2 ° C is considered to be reasonably manageable with regard to economic, sociological and ecological consequences, a development beyond the 2 ° C limit value would make the risks from factors such as tilting elements with short-term feedback effects less controllable Grow wisely. However, according to some researchers , the so-called two-degree target should also be subjected to a critical review with regard to underestimated risk potential.
According to climatologist Stefan Rahmstorf, even a comparatively moderate rise in temperature would be an extraordinary event in the context of the last 11,000 years: "We are about to catapult ourselves far out of the Holocene." The fact of current climate change in combination with other factors such as species extinction and acidification the oceans or the reduction of natural biotopes led to the design of the Anthropocene ( ancient Greek : the man-made new ), which, according to the ideas of British geologists and the Dutch Nobel Prize winner for chemistry, Paul J. Crutzen , was to be implemented as the most recent epoch in the chronostratigraphic system of geological history. The decision on the future status of the Anthropocene lies with the International Commission on Stratigraphy (ICS), in whose Working Group on the 'Anthropocene' the various aspects of the proposal are examined in detail. At the 35th International Geological Congress in Cape Town in 2016, this working group voted for the recognition of the Anthropocene , recommending 1950 as the beginning of the new era. In May 2019, the Working Group on the 'Anthropocene' voted with a clear majority in favor of submitting a draft for the introduction of the Anthropocene to the International Commission on Stratigraphy by 2021 , along with a geologically defined starting point for the new epoch.
According to the unanimous scientific opinion, the additional anthropogenic carbon dioxide input into the atmosphere will only decrease gradually even if there is an extensive future emission stop and it will still be detectable in significant quantities in 5000 years. In contrast, the residence time of methane under the current atmospheric conditions is only about 12 years, but the oxidation of this greenhouse gas in turn produces CO 2 . Thus, human influence is likely to shape and change the climate system over the next millennia. Some studies go one step further and postulate a self-reinforcing warming phase with a duration similar to the Paleocene / Eocene temperature maximum , taking into account the Earth system's climate sensitivity and various tipping elements . A long-lasting warm period in the range of 100,000 years, as outlined in various scenarios, would seriously reshape the image of the earth, above all through the shifting of the climatic and vegetation zones and the extensive melting of the Antarctic and Greenland ice sheets with a corresponding rise in sea levels by several tens of meters.
The distant future
Predictions about the climate development of the earth for the next million years are speculative, since the mechanisms involved only allow a rough estimate. However, it is very likely that the events and processes that occurred in the past, such as climatic fluctuations , mass extinctions or the megavolcanism of a magmatic large province , will continue to shape geological history in the future. In principle, there is nothing to prevent the Cenozoic Ice Age from being replaced by a warm period with ice-free polar regions and tropical vegetation, as was the case, for example, in the Eocene 50 million years ago. The future position of the continental plates plays a decisive role here. On the basis of the current plate tectonic cycle, the following land distribution would result in 50 to 200 million years:
- Africa: The Somali Plateau , east of the Great Rift Valley, is likely to split off from Africa in just a few million years and migrate eastwards towards India. The rest of the continent will continue to move north and merge with the Eurasian Plate into a major continent that is gradually drifting northeast. Instead of the displaced Mediterranean, a new high mountain range with significantly larger dimensions than the Alps will emerge at the interface between the two continental plates.
- Antarctica: After the continent has always been positioned in the immediate vicinity of the South Pole region since the late Mesozoic , it will move north in the future and reach the equator in an estimated 150 to 200 million years. The same applies to Australia , which is moving relatively quickly further north and could collide with Japan in around 80 million years.
- North America and South America: The two continents are expected to separate again at their narrowest point, with North America (without the now detached Baja California , but together with Greenland and Newfoundland ) first turning west and then, in more than 100 million years, further south drifts. Around the same time, Greenland will occupy a position between 20 ° and 30 ° south latitude. Meanwhile, the Atlantic Ocean continues to expand along the splitting zone of the Mid-Atlantic Ridge , while the Pacific is shrinking at the same rate.
Beyond the timeframe of this projection, most studies assume that the land masses reunite to form a supercontinent in the course of the Wilson cycle , which could then possibly be the last in earth's history. It is generally expected that the plate tectonic processes will slow down and weaken in about 500 million years due to the gradual cooling of the earth's interior. Thus, a significant imbalance between erosion- related carbon sequestration and CO 2 outgassing is likely to arise. More CO 2 is withdrawn from the atmosphere than is newly added, and in the course of this development the carbon dioxide will drop to a concentration of less than 150 ppm , which is a threat to the existence of C 3 plants. On the other hand, it takes more than a billion years for C 4 plants to reach the lower limit of 10 ppm, but at that point there will almost certainly no longer be a biosphere in its present form.
When asked about how long atmospheric CO 2 will be available, the various studies give very different answers. Solar radiation will unfold its effect faster and more sustainably than the dwindling carbon cycle. In 800 to 900 million years it will warm the atmosphere so extremely that most ecosystems will inevitably collapse. From this point onwards, more highly organized life is hardly possible on the earth's surface. Any adaptation strategies of the organisms concerned are likely to be useless, since the high evaporation factor of the oceans with the corresponding water vapor feedback will cause a galloping greenhouse effect . In a billion years, the earth could become a world of bacteria that will survive for a while in protected areas such as deeper layers of the ocean. But the oceans are also an endangered habitat in the longer term. With the complete evaporation of the surface water, life, which probably only consists of prokaryotes , remains the inside of the lithosphere as the last possibility of retreat .
During its development into the red giant star , the sun will melt large parts of the earth's crust and turn them into magma lakes. This ends the biologically and climatically relevant natural history of the planet in a similar form to how it began: with the earth as a glowing, sterile celestial body, surrounded by a mantle of hot gases.
See also
literature
English language books
- William F. Ruddimann: Earth's Climate - Past and Future. Palgrave Macmillan, 2001, ISBN 0-7167-3741-8 .
- Raymond T. Pierrehumbert: Principles of Planetary Climate. Cambridge University Press, 2010, ISBN 978-0-521-86556-2 .
- Thomas N. Cronin: Paleoclimates: understanding climate change past and present. Columbia University Press, New York 2010, ISBN 978-0-231-14494-0 .
- Raymond S. Bradley: Paleoclimatology. Reconstructing Climates of the Quaternary. Academic Press (Elsevier Inc.) Oxford, Amsterdam, Waltham, San Diego, Third Edition 2015, ISBN 978-0-12-386913-5 .
German-language books
- Martin Schwarzbach: The climate of the past. An Introduction to Paleoclimatology. 5th edition. Enke, Stuttgart 1993, ISBN 3-432-87355-7 .
- Monika Huch, Günter Warnecke, Klaus Germann (eds.): Climatic testimonies of geological history. Perspectives for the future . With contributions by Wolfgang H. Berger, Arthur Block, Werner von Bloh, Werner Buggisch, Klaus Germann, Monika Huch, Gerhard Petschel-Held, Hans-Joachim Schellnhuber, Torsten Schwarz, Hansjörg Streif, Otto H. Wallner, Günter Warnecke, Gerold Wefer . Springer, Berlin / Heidelberg 2001, ISBN 3-540-67421-7 .
- József Pálfy: Disasters in the history of the earth. Global extinction? Schweizerbart, Stuttgart 2005, ISBN 3-510-65211-8 .
- Christoph Buchal, Christian-Dietrich Schönwiese: Climate. The earth and its atmosphere through the ages . Ed .: Wilhelm and Else Heraeus Foundation, Helmholtz Association of German Research Centers, 2nd edition. Hanau 2012, ISBN 978-3-89336-589-0 .
- Christian-Dietrich Schönwiese: climatology. 4th, revised and updated edition. UTB, Stuttgart 2013, ISBN 978-3-8252-3900-8 .
Specialized journals related to paleoclimatology
- PNAS (Proceedings of the National Academy of Sciences), publisherː National Academy of Sciences (USA), languageː English, frequencyː weekly, Linkː official website , ISSN 0027-8424
- Nature Geoscience ,Editorː Nature Publishing Group (GB), Languageː English, Frequency Monthly, Linkː official website , ISSN 1752-0894
- Geophysical Research Letters ,Editorː American Geophysical Union (USA), Languageː English, Frequency Erschein fortnightly, Linkː official website , ISSN 0094-8276
- Geology ,Editorː Geological Society of America (USA), Languageː English, Frequency Monthly, Linkː official website , ISSN 0091-7613
- Palaeogeography, Palaeoclimatology, Palaeoecology ("Palaeo3") , Verlagː Elsevier , languageː English, frequency of publicationː 56 issues per year, Linkː official website , ISSN 0031-0182
- Gondwana Research , Verlagː Elsevier , languageː English, frequency of publicationː monthly, Linkː official website , ISSN 1342-937X
Web links
- International Chronostratigraphic Chart 2020/03 (Regularly updated chronostratigraphic chart of the International Commission on Stratigraphy )
- Paleoclimatology Data. NOAA National Climatic Data Center, accessed June 29, 2014 .
- Information on the "Greenland Ice Core Project" of the "National Climatic Data Center" (NOAA) (English)
Individual evidence
Footnotes within a sentence or after a comma refer directly to an individual statement, footnotes at the end of a sentence or paragraph refer to the entire preceding text.
- ^ IPCC, 2013: Summary for Policymakers. In: Climate Change 2013: The Physical Science Basis. Contribution of Working Group I to the Fifth Assessment Report of the Intergovernmental Panel on Climate Change [Stocker, TF, D. Qin, G.-K. Plattner, M. Tignor, SK Allen, J. Boschung, A. Nauels, Y. Xia, V. Bex and PM Midgley (eds.)]. Cambridge University Press, Cambridge, United Kingdom and New York, NY, USA .: Summary for policymakers .
- ^ Keith Montgomeryː Book Review and Essay: The Geology and Physical Geography of Robert Hooke (1635–1703) (PDF). University of Wisconsin. (accessed on February 10, 2015)
- ↑ Urs B. Leu: Oswald Heer (1809-1883): Paleobotanist and critic of Darwin. (PDF) Quarterly publication of the Natural Research Society in Zurich (2009) 154 (3/4), pp. 83–95. (accessed on March 25, 2015)
- ↑ Edmund Blair Bolles: Ice Age. How a professor, a politician and a poet discovered the eternal ice , Argon Verlag, Berlin 2000, ISBN 3-87024-522-0 , p. 34 ff.
- ↑ Jürgen Ehlers: The Ice Age , Spectrum Academic Publishing House, Heidelberg 2011, ISBN 978-3-8274-2326-9 , p. 16.
- ^ Svante Arrhenius: On the Influence of Carbonic Acid in the Air upon the Temperature of the Ground. In: Philosophical Magazine and Journal of Science. 41, 1896, pp. 239–276 globalwarmingart.com ( Memento from October 6, 2014 in the Internet Archive ) (PDF; accessed on August 23, 2013)
- ^ Hermann Rump: The development of dendrochronology in Europe (concept for the investigation of a dating method) (PDF). Friedrichsdorf Institute for Sustainability, 2010. (accessed on March 4, 2015)
- ↑ Future challenges for the geosciences. Senate Commission for Future Tasks in Geosciences of the German Research Foundation (DFG), Bremen 2014.
- ↑ C. Sagan, G. Mullen: Earth and Mars: Evolution of Atmospheres and Surface Temperatures. (PDF). In: Science. 177, pp. 52-56 (1972). doi: 10.1126 / science.177.4043.52 . (accessed on November 18, 2014)
- ↑ Jacob D. Haqq-Misra, Shawn D. Domagal-Goldman, Patrick J. Kasting, James F. Kasting: A Revised, Hazy Methane Greenhouse for the Archean Earth. In: Astrobiology. Vol. 8, No. 6, pp. 1127-1137 (2008). doi: 10.1089 / ast.2007.0197 .
- ↑ a b L. W. Alvarez, W. Alvarez, F. Asaro, HV Michel: Extraterrestrial Cause for the Cretaceous-Tertiary Extinction . (PDF) In: Science . 208, June 1980, pp. 1095-1108.
- ↑ a b Michael M. Joachimski, Xulong Lai, Shuzhong Shen, Haishui Jiang, Genming Luo, Bo Chen, Jun Chen, Yadong Sun: Climate warming in the latest Permian and the Permian – Triassic mass extinction . (PDF) In: Geology . 40, No. 3, March 2012, pp. 195-198. doi : 10.1130 / G32707 .
- ↑ Thomas Stocker : Introduction to Climate Modeling . (PDF) In: Physikalisches Institut, University of Bern . .
- ↑ Frank Kaspar, Ulrich Cubasch: The climate at the end of a warm period. In: U. Cubasch (ed.): The animated planet II . Berlin 2007 ( PDF ).
- ^ Arno Semmel: Geomorphology of the Federal Republic of Germany . Steiner Verlag, 1996, ISBN 3-515-06897-X .
- ^ NF Alley, SB Hore, LA Frakes: Glaciations at high-latitude Southern Australia during the Early Cretaceous . (PDF) In: Australian Journal of Earth Sciences (Geological Society of Australia) . April 2019. doi : 10.1080 / 08120099.2019.1590457 .
- ^ University of Hohenheim (Institute for Botany): Dendrochronology - The Hohenheim Tree Ring Calendar.
- ↑ Marco Spurk, Michael Friedrich, Jutta Hofmann, Sabine Remmele, Burkhard Frenzel, Hanns Hubert Leuschner, Bernd Kromer: Revisions and extension of the Hohenheim oak and pine chronologies: New evidence about the timing of the Younger Dryas / Preboreal transition. Inː Radiocarbon , 40, 1998, pp. 1107-1116.
- ^ R. Dull, J. Southon, S. Kutterolf, A. Freundt, D. Wahl, P. Sheets: Did the TBJ Ilopango eruption cause the AD 536 event? In: AGU Fall Meeting Abstracts, December 2010. bibcode : 2010AGUFM.V13C2370D
- ↑ WS McKerrow (ed.): Ecology of fossils: communities, habitats, ways of life . 2nd edition, Franckh-Kosmos, Stuttgart 1992, ISBN 3-440-06565-0 .
- ↑ A. Brauer: Lake sediments of the Holzmaares from the Vistula Period - varven chronology of the high glacial and evidence of climatic fluctuations . In documenta naturae , Munich 1994, ISSN 0723-8428 , p. 85.
- ↑ F. Wilhelms, H. Miller, MD Gerasimoff, C. Druecker, A. Frenzel, D. Fritzsche, H. Grobe, SB Hansen, SAE Hilmarsson, G. Hoffmann, K. Hörnby, A. Jaeschke, SS Jakobsdottir, P Juckschat, A. Karsten, L. Karsten, PR Kaufmann, T. Karlin, E. Kohlberg, G. Kleffel, A. Lambrecht, A. Lambrecht, G. Lawer, I. Schaermeli, J. Schmitt, SG Sheldon, M Takata, M. Trenke, B. Twarloh, F. Valero-Delgado, D. Wilhelms-Dick: The EPICA Dronning Maud Land deep drilling operation . (PDF) In: Annals of Glaciology . 55, No. 68, 2014, pp. 355-366. doi : 10.3189 / 2014AoG68A189 .
- ^ S. Ross Taylor, Scott McLennan, Planetary Crusts: Their Composition, Origin and Evolution . Cambridge Planetary Science, 2009, ISBN 978-0-521-84186-3 , p. 22 f., P. 208.
- ↑ Roi Granot: Palaeozoic oceanic crust preserved beneath the eastern Mediterranean . In: Nature Geoscience . August 2016. doi : 10.1038 / ngeo2784 .
- ^ A b Matthew M. Wielicki, T. Mark Harrison, Daniel Stockl: Dating terrestrial impact structures: U-Pb depth profiles and (U-Th) / He ages of zircon . (PDF) In: Geophysical Research Letters . 41, No. 12, June 2014, pp. 4168-4175. doi : 10.1002 / 2014GL060757 .
- ↑ Christo Buizerta, Daniel Baggenstos, Wei Jiang, Roland Purtschert, Vasilii V. Petrenko, Zheng-Tian Luc, Peter Müller, Tanner Kuhl, James Lee, Jeffrey P. Severinghaus, Edward J. Brook: adiometric 81 Kr dating identifies 120,000-year -old ice at Taylor Glacier, Antarctica . In: pnas . 111, No. 19, May 2014, pp. 6876-6881. doi : 10.1073 / pnas.1320329111 .
- ↑ K. Hughen, S. Lehman, J. Southon, J. Overpeck, O. Marchal, C. Herring, J. Turnbull: 14 C Activity and Global Carbon Cycle Changes over the Past 50,000 Years . (PDF) In: Science . 303, January 2004, pp. 202-207.
- ↑ Heather D. Graven: Impact of fossil fuel emissions on atmospheric radiocarbon and various applications of radiocarbon over this century . In: pnas . 112, No. 31, July 2015, pp. 9542-9545. doi : 10.1073 / pnas.1504467112 . (accessed on August 30, 2015)
- ↑ K. Panchuk, A. Ridgwell, LR Kump: Sedimentary response to Paleocene-Eocene Thermal Maximum carbon release: A model-data comparison . In: Geology . tape 36 , no. 4 , 2008, p. 315-318 , doi : 10.1130 / G24474A.1 .
- ↑ Melanie J. Leng, Jim D. Marshall: Palaeoclimate interpretation of stable isotope data from lake sediment archives . In: Quaternary Science Reviews . tape 23 , no. 7–8 , April 2004, pp. 811-831 , doi : 10.1016 / j.quascirev.2003.06.012 .
- ↑ Michael Zech, Carolin Bimüller, Andreas Hemp, Cyrus Samimi, Christina Broesike, Claudia Hörold, Wolfgang Zech: Human and climate impact on 15 N natural abundance of plants and soils in high-mountain ecosystems: a short review and two examples from the Eastern Pamirs and Mt. Kilimanjaro . In: Isotopes in Environmental and Health Studies . tape 47 , no. 3 , July 2011, p. 286-296 , doi : 10.1080 / 10256016.2011.596277 .
- ↑ Jung-Hyun Kim, Stefan Schouten, Ellen C. Hopmans, Barbara Donner, Jaap S. Sinninghe Damsté: Global sediment core-top calibration of the TEX 86 paleothermometer in the ocean . In: Geochimica et Cosmochimica Acta . tape 72 , no. 4 , February 15, 2008, p. 1154–1173 , doi : 10.1016 / j.gca.2007.12.010 .
- ↑ F. Ritterbusch, S. Ebser, J. Welte, T. Reichel, A. Kersting, R. Purtschert, W. Aeschbach-Hertig, MK Oberthaler: Groundwater dating with Atom Trap Trace Analysis of 39 Ar . In: Geophysical Research Letters . 41, No. 19, October 2014, pp. 6758-6764. doi : 10.1002 / 2014GL061120 .
- ↑ Daniel Siegel: Global climate change caused by the sun? Fluctuations in radiation intensity (PDF). Kiepenheuer Institute for Solar Physics, Freiburg 2010.
- ^ Adam R. Sarafian, Horst R. Marschall, Francis M. McCubbin, Brian D. Monteleone: Early accretion of water in the inner solar system from a carbonaceous chondrite-like source . (PDF) In: Science . 346, October 2014, pp. 623–626. doi : 10.1126 / science.1256717 .
- ^ Henry H. Hsieh, David Jewitt: A Population of Comets in the Main Asteroid Belt. In Science, Volume 312, 2006, pp. 561-563, doi: 10.1126 / science.1125150 , (PDF) ( Memento from September 6, 2008 in the Internet Archive ).
- ↑ David Beerling, Robert A. Berner, Fred T. Mackenzie, Michael B. Harfoot, John A. Pyle: Methane and the CH 4 -related greenhouse effect over the past 400 million years . (PDF) In: American Journal of Science . 309, February 2009, pp. 97-113. doi : 10.2475 / 02.2009.01 .
- ^ Stefan Rahmstorf: Climate change - some facts . In: From Politics and Contemporary History (APuZ 47/2007)
- ↑ Animation by CIRES / NOAAː Representation of the carbon dioxide concentration in the atmosphere using different time scales .
- ↑ James Hansen , Makiko Sato, Pushker Kharecha, David Beerling, Robert Berner, Valerie Masson-Delmotte, Mark Pagani, Maureen Raymo, Dana L. Royer, James C. Zachos : Target Atmospheric CO 2 : Where Should Humanity Aim? In: The Open Atmospheric Science Journal. Vol. 2, 2008, pp. 217–231, doi: 10.2174 / 1874282300802010217 columbia.edu (PDF; accessed October 24, 2014)
- ↑ Eric Monnin, Andreas Indermühle, André Dällenbach, Jacqueline Flückiger, Bernhard Stauffer, Thomas F. Stocker, Dominique Raynaud, Jean-Marc Barnola: Atmospheric CO 2 Concentrations over the Last Glacial Termination. In: Science. Vol. 291, no. 5501, January 5, 2001, pp. 112-114, doi: 10.1126 / science.291.5501.112
- ^ Thomas Wagner, Christoph Hörmann, Marloes Penning de Vries, Holger Sihler: Global monitoring of volcanic emissions with satellite instruments . Research report 2011, Max Planck Institute for Chemistry
- ↑ Volcanic Gases and Climate Change Overview . Volcano Hazards Program, USGS (US Geological Survey).
- ^ A b F. Jourdan, K. Hodges, B. Sell, U. Schaltegger, MTD Wingate, LZ Evins, U. Söderlund, PW Haines, D. Phillips, T. Blenkinsop: High-precision dating of the Kalkarindji large igneous province , Australia, and synchrony with the Early-Middle Cambrian (Stage 4-5) extinction . (PDF) In: Geology . 42, No. 6, June 2014, pp. 543-546. doi : 10.1130 / G35434.1 .
- ↑ a b Stephan V. Sobolev, Alexander V. Sobolev, Dmitry V. Kuzmin, Nadezhda A. Krivolutskaya, Alexey G. Petrunin, Nicholas T. Arndt, Viktor A. Radko, Yuri R. Vasiliev: Linking mantle plumes, large igneous provinces and environmental catastrophes . (PDF) In: Nature . 477, No. 7364, September 2011, pp. 312-316. doi : 10.1038 / nature10385 .
- ↑ Hetu C. Sheth: “Large Igneous Provinces (LIPs)”: Definition, recommended terminology, and a hierarchical classification. Revised version of the article of the same name in Earth Science Reviews. Volume 85, pp. 117–124, 2007. mantleplumes.org (PDF; accessed November 17, 2014)
- ^ Robert B. Smith, Lawrence W. Braile: Crustal Structure and Evolution of an Explosive Silicic Volcanic System at Yellowstone National Park . In Geology of Yellowstone Park Area; 33rd Annual Field Conference Guidebook , 1982, pp. 233-250.
- ↑ Lesson 1 starter activity: Ranking the seven continents (PDF). Publication by the Royal Geographical Society. (accessed on November 17, 2014)
- ↑ Spencer G. Lucas, Joerg W. Schneider, Giuseppe Cassinis: Non-marine Permian biostratigraphy and biochronology: an introduction. In: Spencer G. Lucas, Giuseppe Cassinis, Joerg W. Schneider (Eds.): Non-Marine Permian Biostratigraphy and Biochronology. Geological Society, London, Special Publications, 265, London 2006, pp. 1-14. sp.lyellcollection.org (PDF)
- ^ Neil J. Tabor: Wastelands of tropical Pangea: High heat in the Permian . In: Geology . tape 41 , no. 5 , 2013, p. 623–624 , doi : 10.1130 / focus052013.1 .
- ↑ Frank Körnerː Climate and sedimentation patterns of the peri-tethyalen, continental Permian - interdisciplinary studies on red beds of the Lodève basin (S-France). Faculty of Geosciences, Geotechnics and Mining of the Technical University Bergakademie Freiberg, 2005. [1] (PDF; accessed on February 24, 2020)
- ↑ Isabel P. Montañez, Neil J. Tabor, Deb Niemeier, William A. DiMichele, Tracy D. Frank, Christopher R. Fielding, John L. Isbell, Lauren P. Birgenheier, Michael C. Rygel: CO 2 -Forced Climate and Vegetation Instability During Late Paleozoic Deglaciation . (PDF) In: Science . 315, No. 5808, January 2007, pp. 87-91. doi : 10.1126 / science.1134207 . (accessed on January 20, 2016)
- ^ Definition of Antarctica. (In common parlance, the land mass at the South Pole is often referred to as Antarctica . The correct geographical names are Antarctica for the continent and Antarctica for the south polar region.)
- ↑ Franz v. Cernyː The changeability of the climate and its causes (PDF), A. Hartleben's Verlag, Vienna - Pest - Leipzig 1881.
- ^ Edwin Kemper: The climate of the Cretaceous period . (= Geological Yearbook. Series A, Issue 96). Published by the Federal Institute for Geosciences and Raw Materials and the State Geological Offices in the Federal Republic of Germany, E. Schweizerbart'sche Verlagsbuchhandlung, Stuttgart 1987, ISBN 3-510-96400-4 , p. 105, p. 111 ff.
- ↑ Dennis V. Kent, Paul E. Olsen, Cornelia Rasmussen, Christopher Lepre, Roland Mundil, Randall B. Irmis, George E. Gehrels, Dominique Giesler, John W. Geissman, William G. Parker: Empirical evidence for stability of the 405 -kiloyear Jupiter – Venus eccentricity cycle over hundreds of millions of years . In: PNAS . 115, No. 24, June 2018. doi : 10.1073 / pnas.1800891115 .
- ↑ Ilja J. Kocken, Margot J. Cramwinckel, Richard E. Zeebe, Jack J. Middelburg, Appy Sluijs: The 405 kyr and 2.4 Myr eccentricity components in Cenozoic carbon isotope records . (PDF) In: Climate of the Past . January 15, 2019, pp. 91-104. doi : 10.5194 / cp-15-91-2019 .
- ^ A. Ganopolski, R. Winkelmann, HJ Schellnhuber: Critical insolation - CO 2 relation for diagnosing past and future glacial inception . In: Nature . 529, No. 7585, January 2016, pp. 200-203. doi : 10.1038 / nature16494 .
- ↑ József Pálfy: Disasters in the history of the earth. Global extinction? Schweizerbart, Stuttgart 2005, p. 102.
- ↑ G. Rugel, T. Faestermann, K. Knie, G. Korschinek, M. Poutivtsev, D. Schumann, N. Kivel, I. Günther-Leopold, R. Weinreich, M. Wohlmuther: New Measurement of the 60 Fe Half -Life. In: Physical Review Letters. Vol. 103, Issue 7, 2009, doi: 10.1103 / PhysRevLett.103.072502 .
- ↑ K. Knie, G. Korschinek, T. Faestermann, EA Dorfi, G. Rugel, A. Wallner: 60 Fe Anomaly in a Deep-Sea Manganese Crust and Implications for a Nearby Supernova Source . (PDF) In: Physical Review Letters . 93, No. 17, October 2004, pp. 171103-1-171103-4. doi : 10.1103 / PhysRevLett.93.171103 .
- ↑ Alexandra Witze: Supernova left its mark in ancient bacteria. In: Nature, April 2013. doi: 10.1038 / nature.2013.12797
- ^ A. Wallner, J. Feige, N. Kinoshita, M. Paul, LK Fifield, R. Golser, M. Honda, U. Linnemann, H. Matsuzaki, S. Merchel, G. Rugel, SG Tims, P. Steier , T. Yamagata, SR Winkler: Recent near-Earth supernovae probed by global deposition of interstellar radioactive 60 Fe . In: Nature . 532, No. 7597, April 2016, pp. 69-72. doi : 10.1038 / nature17196 .
- ↑ AL Melott, BS Lieberman, CM Laird, LD Martin, MV Medvedev, BC Thomas, JK Cannizzo, N. Gehrels, CH Jackman: Did a gamma-ray burst initiate the late Ordovician mass extinction? In: International Journal of Astrobiology . tape 3 , no. 2 , 2004, p. 55–61 , doi : 10.1017 / S1473550404001910 , arxiv : astro-ph / 0309415 .
- ^ NJ Shaviv: Toward a solution to the early faint Sun paradox: A lower cosmic ray flux from a stronger solar wind. In: Journal of Geophysical Research. 108 (A12), 2003, p. 1437. doi: 10.1029 / 2003JA009997
- ^ Andrew C. Overholt, Adrian L. Melott, Martin Pohl: Testing the link between terrestrial climate change and galactic spiral arm transit . In: The Astrophysical Journal . No. 705, November 2009, pp. L101-L103. doi : 10.1088 / 0004-637X / 705/2 / L101 .
- ↑ M. Scheffer, V. Brovkin, P. Cox: Positive feedback between global warming and atmospheric CO 2 concentration inferred from past climate change. In: Geophysical Research Letters. 33 (2006), p. L10702, doi: 10.1029 / 2005GL025044
- ↑ Kristina Pistone, Ian Eisenman, Veerabhadran Ramanathan : Observational determination of albedo decrease caused by vanishing Arctic sea ice . In: Proceedings of the National Academy of Sciences . 111, No. 9, March 2014, pp. 3322-3326. doi : 10.1073 / pnas.1318201111 .
- ↑ AY Glikson, AJ Meixner, B. Radke, IT Uysal, E. Saygin, J. Vickers, TP Mernagh: Geophysical anomalies and quartz deformation of the structure Warburton West, central Australia . (PDF) In: Tectonophysics . 643, March 2015, pp. 55-72. doi : 10.1016 / j.tecto.2014.12.010 .
- ^ William K. Hartmann, Donald R. Davis: Satellite-sized planetesimals and lunar origin . In Icarus , Volume 24, Number 4, 1975, pp. 504-515, doi: 10.1016 / 0019-1035 (75) 90070-6
- ↑ Robin M. Canup: Simulations of a late lunar-forming impact (PDF), Icarus, Vol. 168, 2004, pp. 433-456. (accessed October 29, 2014)
- ^ G. Pannella: Paleontological Evidence on the Earth's Rotational History since Early Precambrian . Astrophysics and Space Science 16 (1972), pp. 212-237. bibcode : 1972Ap & SS..16..212P
- ↑ WK Hartmann: Megaregolith evolution and cratering cataclysm models. Lunar cataclysm as a misconception (28 years later) . In: Meteoritics & Planetary Science . 38, No. 4, April 2003, pp. 579-593. doi : 10.1111 / j.1945-5100.2003.tb00028.x .
- ^ William F. Bottke, David Vokrouhlický, David Minton, David Nesvorný, Alessandro Morbidelli, Ramon Brasser, Bruce Simonson, Harold F. Levison: An Archaean heavy bombardment from a destabilized extension of the asteroid belt . In: Nature . 485, No. 7396, June 2006, pp. 78-81. doi : 10.1038 / nature10967 .
- ↑ A. Mund, RJ Walker, JR Reimink, RL Rudnick, RM Gaschnig: Tungsten-182 in the upper continental crust: Evidence from glacial diamictites . (PDF) In: Chemical Geology . 494, September 2018, pp. 144–152. doi : 10.1016 / j.chemgeo.2018.07.036 .
- ^ A b James F. Kasting, Shuhei Ono: Palaeoclimates: the first two billion years . (PDF): The Royal Society Publishing, Philosophical Transactions B . June 2006. doi : 10.1098 / rstb.2006.1839 .
- ↑ Robert E. Kopp, Joseph L. Kirschvink, Isaac A. Hilburn, Cody Z. Nash: The Paleoproterozoic snowball Earth: A climate disaster triggered by the evolution of oxygenic photosynthesis . In: Proc. Natl. Acad. Sci. USA . 102, No. 32, 2005, pp. 11131-11136. doi : 10.1073 / pnas.0504878102 .
- ↑ Phillip W. Schmidt, George E. Williams: Paleomagnetism of the Lorrain Formation, Quebec, and Implications for The Latitude of Huronian Glaciation (PDF), Geophysical Research Abstracts, Vol. 5, 08262, 2003. (accessed November 5, 2014 )
- ^ Heinrich D. Holland: The oxygenation of the atmosphere and oceans . In: Philosophical Transactions of the Royal Society B . 361, No. 1470, June 2006, pp. 903-915. doi : 10.1098 / rstb.2006.1838 .
- ^ Alan D. Rooney, Justin V. Strauss, Alan D. Brandon, Francis A. Macdonald: A Cryogenian chronology: Two long-lasting synchronous Neoproterozoic glaciations . (PDF) In: Geology . 43, No. 5, May 2015, pp. 459-462. doi : 10.1130 / G36511.1 .
- Jump up ↑ Judy P. Pu, Samuel A. Bowring, Jahandar Ramezani, Paul Myrow, Timothy D. Raub, Ed Landing, Andrea Mills, Eben Hodgin, Francis A. Macdonald: Dodging snowballs: Geochronology of the Gaskiers glaciation and the first appearance of the Ediacaran biota . (PDF) In: Geology . 44, No. 11, November 2016, pp. 955-958. doi : 10.1130 / G38284.1 .
- ↑ A Neoproterozoic Snowball Earth , Science Vol. 281, No. 5381, pp. 1342-1346, August 28, 1998.
- ↑ Nick Eyles: Glacio-epochs and the supercontinent cycle after ~ 3.0 Ga: Tectonic boundary conditions for glaciation . In: Palaeogeography, Palaeoclimatology, Palaeoecology . 258, No. 1-2, February 2008, pp. 89-129. doi : 10.1016 / j.palaeo.2007.09.021 .
- ↑ Irina V. Gorodetskaya, Mark A. Cane, L.-Bruno Tremblay, Alexey Kaplan: The effects of sea-ice and land-snow concentrations on planetary albedo from the earth radiation budget experiment . In: Atmosphere-Ocean . 44, No. 2, 2006, pp. 195-205. doi : 10.3137 / ao.440206 .
- ^ Philip Allen and James Etienne: Sedimentary challenge to Snowball Earth. Nature Geoscience, 1, pp. 817-825, 2008.
- ^ Dorian S. Abbot, Raymond T. Pierrehumbert: Mudball: Surface dust and Snowball Earth deglaciation . In: Journal of Geophysical Research . 115, No. D3, February 2010. doi : 10.1029 / 2009JD012007 .
- ^ Frank A. Corsetti, Stanley M. Awramik, David Pierce: A complex microbiota from snowball Earth times: Microfossils from the Neoproterozoic Kingston Peak Formation, Death Valley, USA . In: pnas . 100, No. 8, April 2003, pp. 4399-4404. doi : 10.1073 / pnas.0730560100 .
- ↑ DE Canfield , SW Poulton, GM Narbonne: Late-Neoproterozoic deep-ocean oxygenation and the rise of animal life . In: Science . 315, No. 5808, January 2007, pp. 92-5. doi : 10.1126 / science.1135013 . PMID 17158290 .
- ↑ JEN Veron: Mass extinctions and ocean acidification: biological constraints on geological dilemmas . (PDF) In: Coral Reefs . 27, No. 3, September 2008, pp. 459-472. doi : 10.1007 / s00338-008-0381-8 .
- ^ Richard J. Twitchett: The palaeoclimatology, palaeoecology and palaeoenvironmental analysis of mass extinction events . (PDF) In: Palaeogeography, Palaeoclimatology, Palaeoecology . 232, No. 2-4, March 2006, pp. 190-213. doi : 10.1016 / j.palaeo.2005.05.019 .
- ^ Peter Ward: Under a Green Sky: Global Warming, the Mass Extinctions of the Past, and What They Can Tell Us About Our Future. 2007, ISBN 978-0-06-113791-4 .
- ^ Benjamin C. Gill, Timothy W. Lyons, Seth A. Young, Lee R. Kump, Andrew H. Knoll, Matthew R. Saltzman: Geochemical evidence for widespread euxinia in the Later Cambrian ocean . In: Nature . 469, January 2011, pp. 80-83. doi : 10.1038 / nature09700 .
- ↑ Pascale F. Poussart, Andrew J. Weaver, Christopher R. Barne: Late Ordovician glaciation under high atmospheric CO 2 : A coupled model analysis . (PDF) In: Paleoceanography . 14, No. 4, August 1999, pp. 542-558. doi : 10.1029 / 1999PA900021 .
- ↑ David AT Hapera, Emma U. Hammarlund, Christian M. Ø. Rasmussen: End Ordovician extinctions: A coincidence of causes . (PDF) In: Gondwana Research (Elsevier) . 25, No. 4, May 2014, pp. 1294–1307. doi : 10.1016 / j.gr.2012.12.021 .
- ^ Seth A. Young, Matthew R. Saltzman, Kenneth A. Foland, Jeff S. Linder, Lee R. Kump: A major drop in seawater 87 Sr / 86 Sr during the Middle Ordovician (Darriwilian): Links to volcanism and climate? . In: Geology . 37, No. 10, 2009, pp. 951-954. doi : 10.1130 / G30152A.1 .
- ↑ Thijs RA Vandenbroucke, Poul Emsbo, Axel Munnecke, Nicolas Nuns, Ludovic Duponchel, Kevin Lepot, Melesio Quijada, Florentin Paris, Thomas Servais, Wolfgang Kiessling: Metal-induced malformations in early Palaeozoic plankton are harbingers of mass extinctions . In: Nature Communications . 6, August 2015. doi : 10.1038 / ncomms8966 .
- ↑ John A. Long, Ross R. Large, Michael SY Lee, Michael J. Benton, Leonid V. Danyushevsky, Luis M. Chiappe, Jacqueline A. Halpin, David Cantrill, Bernd Lottermoser: Severe selenium depletion in the Phanerozoic oceans as a factor in three global mass extinction events . (PDF) In: Gondwana Research . 36, August 2016, pp. 209-218. doi : 10.1016 / j.gr.2015.10.001 .
- ↑ A. Melott, B. Lieberman, C. Laird, Martin L., M. Medvedev, B. Thomas, J. Cannizzo, N. Gehrels, C. Jackman: Did a gamma-ray burst initiate the late Ordovician mass extinction? In: International Journal of Astrobiology . tape 3 , no. 2 , 2004, p. 55–61 , doi : 10.1017 / S1473550404001910 , arxiv : astro-ph / 0309415 .
- ↑ David PG Bond, Stephen E. Grasby: On the causes of mass extinctions . In: Palaeogeography, Palaeoclimatology, Palaeoecology . 478, No. 15, July 2017, pp. 3–29. doi : 10.1016 / j.palaeo.2016.11.005 .
- ↑ Leszek Marynowski, Michał Zatoń, Michał Rakociński, Paweł Filipiak, Slawomir Kurkiewicz, Tim J. Pearce: Deciphering the upper Famennian Hangenberg Black Shale depositional environments based on multi-proxy record . (PDF) In: Palaeogeography, Palaeoclimatology, Palaeoecology . 346-347, August 2012, pp. 66-86. doi : 10.1016 / j.palaeo.2012.05.020 .
- ↑ Marina Kloppischː Organic-geochemical comparison of selected rocks of the Frasnium / Famennium border (Oberdevon) in the Bergisches Land and the Eifel (PDF). Reports from Forschungszentrum Jülich, Institute for Chemistry and Dynamics of the Geosphere, 2002. (accessed on October 24, 2014)
- ^ David De Vleeschouwer, Micha Rakociński, Grzegorz Racki, David PG Bond, Katarzyna Sobień, Philippe Claeys: The astronomical rhythm of Late-Devonian climate change (Kowala section, Holy Cross Mountains, Poland) . (PDF) In: Earth and Planetary Science Letters . No. 365, 2013, pp. 25-37. doi : 10.1016 / j.epsl.2013.01.016 .
- Jump up ↑ Andrew J. Retzler, Leif Tapanila, Julia R. Steenberg, Carrie J. Johnson, Reed A. Myers: Post-impact depositional environments as a proxy for crater morphology, Late Devonian Alamo impact, Nevada . (PDF) In: Geosphere (Geological Society of America) . January 2015. doi : 10.1130 / GES00964.1 .
- ^ Plant Fossils of West Virginia. Climate and the Carboniferous Period . geocraft.com , 2009.
- ↑ Peter J. Franks, Dana L. Royer, David J. Beerling, Peter K. Van de Water, David J. Cantrill, Margaret M. Barbour and Joseph A. Berry: New constraints on atmospheric CO 2 concentration for the Phanerozoic . (PDF) In: Geophysical Research Letters . 31, No. 13, July 2014. doi : 10.1002 / 2014GL060457 .
- ^ DJ Beerling: Low atmospheric CO 2 levels during the Permo-Carboniferous glaciation inferred from fossil lycopsids . In: pnas . 99, No. 20, August 2002. doi : 10.1073 / pnas.202304999 .
- ^ Arthropleura armata fossil of the year 2015 . Paleontological Society
- ^ Andrew C. Scott, Ian J. Glasspool: The diversification of Paleozoic fire systems and fluctuations in atmospheric oxygen concentration . In: pnas . 103, No. 29, July 2006. doi : 10.1073 / pnas.0604090103 .
- ↑ Isabel P. Montañez, Jennifer C. McElwain, Christopher J. Poulsen, Joseph D. White, William A. DiMichele, Jonathan P. Wilson, Galen Griggs, Michael T. Hren: Climate, pCO 2 and terrestrial carbon cycle linkages during late Palaeozoic glacial – interglacial cycles . (PDF) In: Nature Geoscience . 9, No. 11, November 2016, pp. 824–828. doi : 10.1038 / ngeo2822 .
- ↑ Gerilyn S. Soreghan, Dustin E. Sweet, Nicholas G. Heaven: Upland Glaciation in Tropical Pangea: Geologic Evidence and Implications for Late Paleozoic Climate Modeling . (PDF) In: The Journal of Geology . 122, No. 2, March 2014, pp. 137–163. doi : 10.1086 / 675255 .
- ^ A b Georg Feulner: Formation of most of our coal brought Earth close to global glaciation . In: PNAS . 114, No. 43, October 2017, pp. 11333–11337. doi : 10.1073 / pnas.1712062114 .
- ^ A b Borja Cascales-Miñana and Christopher J. Cleal: The plant fossil record reflects just two great extinction events . In: Terra Nova . 26, No. 3, 2013, pp. 195-200. doi : 10.1111 / ter.12086 .
- ^ William A. DiMichele, Neil J. Tabor, Dan S. Chaney, W. John Nelson: From wetlands to wet spots: Environmental tracking and the fate of Carboniferous elements in Early Permian tropical floras . (PDF) In: GSA (Geological Society of America) . Special Paper 399, 2006, pp. 223-248. doi : 10.1130 / 2006.2399 (11) .
- ↑ Sarda Sahney, Michael Benton, Howard J. Falcon-Lang: Rainforest collapse triggered Pennsylvanian tetrapod diversification in Euramerica . (PDF) In: Geology . 38, No. 12, November 2010, pp. 1079-1082. doi : 10.1130 / G31182.1 .
- ↑ Erik L. Gulbranson, Isabel P. Montañez, Neil J. Tabor, C. Oscar Limarino: Late Pennsylvanian aridification on the southwestern margin of Gondwana (Paganzo Basin, NW Argentina): A regional expression of a global climate perturbation . (PDF) In: Palaeogeography, Palaeoclimatology, Palaeoecology . 417, January 2015, pp. 220-235. doi : 10.1016 / j.palaeo.2014.10.029 .
- ↑ David PG Bond, Paul B. Wignall, Michael M. Joachimski, Yadong Sun, Ivan Savov, Stephen E. Grasby, Benoit Beauchamp, Dierk PG Blomeier: An abrupt extinction in the Middle Permian (Capitanian) of the Boreal Realm (Spitsbergen) and its link to anoxia and acidification . (PDF) In: Geological Society of America Bulletin . (online), April 2015. doi : 10.1130 / B31216.1 .
- ↑ MO Clarkson, SA Kasemann, RA Wood, TM Lenton, SJ Daines, S. Richoz, F. Without Mueller, A. Meixner, SW Poulton, ET Tipper: Ocean acidification and the Permo-Triassic mass extinction . In: Science . 348, No. 6231, April 2015, pp. 229-232. doi : 10.1126 / science.aaa0193 .
- ^ Yadong Sun, Michael M. Joachimski, Paul B. Wignall, Chunbo Yan, Yanlong Chen, Haishui Jiang, Lina Wang, Xulong Lai: Lethally Hot Temperatures During the Early Triassic Greenhouse . (PDF) In: Science . 338, No. 6105, October 2012, pp. 366-370. doi : 10.1126 / science.1224126 .
- ^ Mass extinctions: Peter Ward : The microbes strike back, New Scientist Feb. 9, 2008; Spiegel, 2009
- ^ Daniel H. Rothman, Gregory P. Fournier, Katherine L. French, Eric J. Alm, Edward A. Boyle, Changqun Cao, Roger E. Summons: Methanogenic burst in the end-Permian carbon cycle . In: PNAS . 111, No. 15, April 2014, pp. 5462-5467. doi : 10.1073 / pnas.1318106111 .
- ^ Seth D. Burgess, Samuel Bowring, Shu-zong Shen: High-precision timeline for Earth's most severe extinction . In: PNAS . 111, No. 9, 2014. doi : 10.1073 / pnas.1317692111 .
- ↑ Shu-Zhong Shen, Jahandar Ramezani, Jun Chen, Chang-Qun Cao, Douglas H. Erwin, Hua Zhang, Lei Xiang, Shane D. Schoepfer, Charles M. Henderson, Quan-Feng Zheng, Samuel A. Bowring, Yue Wang , Xian-Hua Li, Xiang-Dong Wang, Dong-Xun Yuan, Yi-Chun Zhang, Lin Mu, Jun Wang, Ya-Sheng Wu: A sudden end-Permian mass extinction in South China . In: GSA Bulletin (The Geological Society of America) . September 2018. doi : 10.1130 / B31909.1 .
- ↑ Adrian P. Jones; David G. Price; Paul S. DeCarli; Richard Clegg: Impact Decompression Melting: A Possible Trigger for Impact Induced Volcanism and Mantle Hotspots? In: C. Koeberl and F. Martinez-Ruiz (eds.): Impact markers in the Stratigraphic Record . Springer Verlag, Berlin 2003, ISBN 3-540-00630-3 , pp. 91-120 ( mantleplumes.org [PDF]).
- ↑ Terrence J. Blackburn, Paul E. Olsen, Samuel A. Bowring, Noah M. McLean, Dennis V. Kent, John Puffer, Greg McHone, E. Troy Rasbury, Mohammed Et-Touhami: Zircon U-Pb Geochronology Links the End -Triassic Extinction with the Central Atlantic Magmatic Province . (PDF) In: Science . 349, No. 366, May 2013, pp. 941-945. doi : 10.1126 / science.1234204 .
- ↑ Katja M. Meyer, Lee R. Kump: Oceanic Euxinia in Earth History: Causes and Consequences . In: Annual Review of Earth and Planetary Sciences . 36, 2008, p. 251. doi : 10.1146 / annurev.earth.36.031207.124256 .
- ↑ Jochen Kuss, Christian Scheibner, Jens Wendler: Oceanic crises in the last 125 million years. What shallow water deposits report (PDF) University of Bremen, Department of Geosciences.
- ↑ Max Planck Institute for Marine Microbiology: Toxic ocean conditions 93.9 million years ago
- ↑ G. Keller, T. Adatte, W. Stinnesbeck, M. Rebolledo-Vieyra, JU Fucugauchi, U. Kramar, Doris Stüben: Chicxulub impact predates the KT boundary mass extinction . In: pnas . 101, No. 11, 2004, pp. 3753-3758. doi : 10.1073 / pnas.0400396101 .
- ↑ Peter Schulte: Comment on the paper "Chicxulub impact predates KT boundary: New evidence from Brazos, Texas" by Keller et al. (2007) . (PDF) In: Earth and Planetary Science Letters . No. 269, 2008, pp. 614-620.
- ^ Paul R. Renne, Alan L. Deino, Frederik J. Hilgen, Klaudia F. Kuiper, Darren F. Mark, William S. Mitchell III, Leah E. Morgan, Roland Mundil, Jan Smit: Time Scales of Critical Events Around the Cretaceous-Paleogene Boundary . (PDF) In: Science . 339, No. 6120, February 2013, pp. 684-687. doi : 10.1126 / science.1230492 .
- ^ Robert Sanders: New evidence comet or asteroid impact was last straw for dinosaurs . In: UC Berkeley News Center, Feb. 7, 2013.
- ↑ Johan Vellekoop, Appy Sluijs, Jan Smit, Stefan Schouten, Johan WH Weijers, Jaap S. Sinninghe Damsté, Henk Brinkhuis: Rapid short-term cooling Following the Chicxulub impact at the Cretaceous-Paleogene boundary . In: pnas . 111, No. 21, May 2014. doi : 10.1073 / pnas.1319253111 .
- ^ Tyler R. Lyson, Antoine Bercovici, Stephen GB Chester, Eric J. Sargis, Dean Pearson, Walter G. Joyce: Dinosaur extinction: closing the '3 m gap' . In: The Royal Society, Biology Letters . July 2011. doi : 10.1098 / rsbl.2011.0470 .
- ↑ Zoltan Siki-Sava, Eric Buffetaut, Attila Ősi, Xabier Pereda-Suberbiola, Stephen L. Brusatte: Island life in the Cretaceous - faunal composition, biogeography, evolution, and extinction of land-living vertebrates on the Late Cretaceous European archipelago . In: ZooKeys . 469, January 2015, pp. 1–161. doi : 10.3897 / zookeys.469.8439 .
- ↑ Michael J. Henehan, Andy Ridgwell, Ellen Thomas, Shuang Zhang, Laia Alegret, Daniela N. Schmidt, James WB Rae, James D. Witts, Neil H. Landman, Sarah E. Greene, Brian T. Huber, James R. Super, Noah J. Planavsky, Pincelli M. Hull: Rapid ocean acidification and protracted Earth system recovery followed the end-Cretaceous Chicxulub impact . In: PNAS . 116, No. 43, October 2019. doi : 10.1073 / pnas.1905989116 .
- ↑ Stephen L. Brusatte, Richard J. Butler, Paul M. Barrett, Matthew T. Carrano, David C. Evans, Graeme T. Lloyd, Philip D. Mannion, Mark A. Norell, Daniel J. Peppe, Paul Upchurch, Thomas E. Williamson: The extinction of the dinosaurs . In: Biological Reviews, Cambridge Philosophical Society (Wiley Online Library) . 90, No. 2, May 2015, pp. 628–642. doi : 10.1111 / brv.12128 .
- ↑ Pincelli M. Hull, André Bornemann, Donald E. Penman, Michael J. Henehan, Richard D. Norris, Paul A. Wilson, Peter Blum, Laia Alegret, Sietske J. Batenburg, Paul R. Bown, Timothy J. Bralower, Cecile Cournede, Alexander Deutsch, Barbara Donner, Oliver Friedrich, Sofie Jehle, Hojung Kim, Dick Kroon, Peter C. Lippert, Dominik Loroch, Iris Moebius, Kazuyoshi Moriya, Daniel J. Peppe, Gregory E. Ravizza, Ursula Röhl, Jonathan D Schueth, Julio Sepúlveda, Philip F. Sexton, Elizabeth C. Sibert, Kasia K. Śliwińska, Roger E. Summons, Ellen Thomas, Thomas Westerhold, Jessica H. Whiteside, Tatsuhiko Yamaguchi, James C. Zachos: On impact and volcanism across the Cretaceous-Paleogene boundary . (PDF) In: Science . 367, No. 6475, January 2020, pp. 266-272. doi : 10.1126 / science.aay5055 .
- ↑ a b Christian Koeberl: Mass Extinctions and Impact Events in Earth History: A Brief Overview. Originally in: Yearbook of the Federal Geological Institute (Austria), Volume 147 / Issue 1 + 2, commemorative publication for the 65th birthday of HR Univ.-Prof. Dr. Hans Peter Schönlaub, Director of the Federal Geological Institute.
- ^ Douglas S. Robertson, Malcolm C. McKenna, Owen B. Toon, Sylvia Hope, Jason A. Lillegraven: Survival in the first hours of the Cenozoic . (PDF) In: Geological Society of America Bulletin . 116, No. 5/6, pp. 760-768. doi : 10.1130 / B25402.1 .
- ↑ Claire M. Belcher: reigniting the Cretaceous-Palaeogene debate firestorm . In: geology . 37, No. 12, 2009, pp. 1147-1148. doi : 10.1130 / focus122009.1 .
- ^ Douglas S. Robertson, William M. Lewis, Peter M. Sheehan, Owen B. Toon: Reevaluation of the heat-fire hypothesis . In: Journal of Geophysical Research: Biogeoscience . 110, No. 1, March 2013, pp. 329–336. doi : 10.1002 / jgrg.20018 .
- ↑ Julia Brugger, Georg Feulner, Stefan Petri: Baby, it's cold outside: Climate model simulations of the effects of the asteroid impact at the end of the Cretaceous . In: Geophysical Research Letters . 44, No. 1, January 2017, pp. 419-427. doi : 10.1002 / 2016GL072241 .
- ↑ Nicholas R. Longrich, Tim Tokaryk, Daniel J. Field: Mass extinction of birds at the Cretaceous-Paleogene (K-Pg) boundary . In: pnas . 108, No. 37, September 2011, pp. 15253-15257. doi : 10.1073 / pnas.1110395108 .
- ↑ Mark A. Richards, Walter Alvarez, Stephen Self, Leif Karlstrom, Paul R. Renne, Michael Manga, Courtney J. Sprain, Jan Smit, Loÿc Vanderkluysen, Sally A. Gibson: Triggering of the largest Deccan eruptions by the Chicxulub impact . (PDF) In: Geological Society of America Bulletin . April 2015. doi : 10.1130 / B31167.1 .
- ^ Joseph S. Byrnes, Leif Karlstrom: Anomalous K-Pg - aged seafloor attributed to impact-induced mid-ocean ridge magmatism . In: Science Advances . 4, No. 2, February 2018. doi : 10.1126 / sciadv.aao2994 .
- ^ Paul R. Renne, Courtney J. Sprain, Mark A. Richards, Stephen Self, Loÿc Vanderkluysen, Kanchan Pande: State shift in Deccan volcanism at the Cretaceous-Paleogene boundary, possibly induced by impact . (PDF) In: Science . 350, No. 6256, October 2015, pp. 76-78. doi : 10.1126 / science.aac7549 .
- ↑ GS Collin, N. Patel, TM Davison, ASP Rae, JV Morgan, SPS Gulick, IODP-ICDP Expedition 364 Science Party: GL Christeson, E. Chenot, P. Claeys, CS Cockell, MJL Coolen, L. Ferrière, C. Gebhardt, K. Goto, H. Jones, DA Kring, J. Lofi, CM Lowery, R. Ocampo-Torres, L. Perez-Cruz, AE Pickersgill, MH Poelchau, C. Rasmussen, M. Rebolledo-Vieyra, U . Riller, H. Sato, J. Smit, SM Tikoo, N. Tomioka, J. Urrutia-Fucugauchi, MT Whalen, A. Wittmann, L. Xiao, KE Yamaguchi, Third-Party Scientists: N. Artemieva, TJ Bralower: A steeply-inclined trajectory for the Chicxulub impact . In: Nature Communications . May 11, 2020. doi : 10.1038 / s41467-020-15269-x .
- ↑ Camilla M. Wilkinson, Morgan Ganerød, Bart WH Hendriks, Elizabeth A. Eide: Compilation and appraisal of geochronological data from the North Atlantic Igneous Province (NAIP) . In: Geological Society, London, Special Publications (Lyell Collection) . 447, November 2016, pp. 69-103. doi : 10.1144 / SP447.10 .
- ↑ Michael Storey, Robert A. Duncan, Carl C. Swisher: Paleocene-Eocene Thermal Maximum and the Opening of the Northeast Atlantic . (PDF) In: Science . 316, No. 5824, April 2007, pp. 587-589. doi : 10.1126 / science.1135274 .
- ^ Richard E. Zeebe, Andy Ridgwell, James C. Zachos: Anthropogenic carbon release rate unprecedented during the past 66 million years . (PDF) In: Nature Geoscience . 9, No. 4, April 2016, pp. 325–329. doi : 10.1038 / ngeo2681 .
- ^ Joost Frieling, Alina I. Iakovleva, Gert-Jan Reichart, Galina N. Aleksandrova, Zinaida N. Gnibidenko, Stefan Schouten, Appy Sluijs: Paleocene-Eocene warming and biotic response in the epicontinental West Siberian Sea . (PDF) In: geology . 42, No. 9, September 2014, pp. 767-770. doi : 10.1130 / G35724.1 .
- ↑ T. Aze, PN Pearson, AJ Dickson, MPS Badger, PR Bown, RD Pancost, SJ Gibbs, BT Huber, MJ Leng, AL Coe, AS Cohen, GL Foster: Extreme warming of tropical waters during the Paleocene-Eocene Thermal Maximum . (PDF) In: geology . 42, No. 9, July 2014, pp. 739-742. doi : 10.1130 / G35637.1 .
- ↑ Donald E. Penman, Bärbel Hönisch , Richard E. Zeebe, Ellen Thomas, James C. Zachos: Rapid and sustained surface ocean acidification during the Paleocene-Eocene Thermal Maximum . (PDF) In: Oceanography . 29, No. 5, May 2014, pp. 357-369. doi : 10.1002 / 2014PA002621 .
- ^ Francesca A. McInerney, Scott L. Wing: The Paleocene-Eocene Thermal Maximum: A Perturbation of Carbon Cycle, Climate, and Biosphere with Implications for the Future . (PDF) In: Annual Review of Earth and Planetary Sciences . 39, May 2011, pp. 489-516. doi : 10.1146 / annurev-earth-040610-133431 . (accessed on August 29, 2016)
- ^ Gary Shaffer, Matthew Huber, Roberto Rondanelli, Jens Olaf Pepke Pedersen: Deep time evidence for climate sensitivity increase with warming . In: Geophysical Research Letters . 43, No. 12, June 2016, pp. 6538-6545. doi : 10.1002 / 2016GL069243 .
- ↑ Eleni Anagnostou, Eleanor H. John, Kirsty M. Edgar, Gavin L. Foster, Andy Ridgwell, Gordon N. Inglis, Richard D. Pancost, Daniel J. Lunt, Paul N. Pearson: Changing atmospheric CO 2 concentration was the primary driver of early Cenozoic climate . In: Nature . 533, May 2016, pp. 380-384. doi : 10.1038 / nature17423 . (accessed on January 11, 2018)
- ↑ EN Speelman, MML van Kempen, J. Barke, H. Brinkhuis, GJ Reichart, AJP Smolders, JGM Roelofs, F. Sangiorgi, JW de Leeuw, AF Lotter, JS Sinninghe Damsté: The Eocene Arctic Azolla bloom: environmental conditions, productivity and carbon drawdown . (PDF) In: Geobiology . 7, No. 2, March 2009, pp. 155-170. doi : 10.1111 / j.1472-4669.2009.00195.x .
- ↑ Henk Brinkhuis, Stefan Schouten, Margaret E. Collinson, Appy Sluijs, Jaap S. Sinninghe Damsté, Gerald R. Dickens, Matthew Huber, Thomas M. Cronin, Jonaotaro Onodera, Kozo Takahashi, Jonathan P. Bujak, Ruediger Stein, Johan van der Burgh, James S. Eldrett, Ian C. Harding, André F. Lotter, Francesca Sangiorgi, Han van Konijnenburg-van Cittert, Jan W. de Leeuw, Jens Matthiessen, Jan Backman, Kathryn Moran: Episodic fresh surface waters in the Eocene Arctic Ocean . (PDF) In: Nature . 441, June 2006, pp. 606-609. doi : 10.1038 / nature04692 .
- ↑ The Chesapeake Bay Impact Crater in the Earth Impact Database ( November 15, 2010 memento in the Internet Archive ). Planetary and Space Science Center, Department of Earth Sciences, University of New Brunswick (Canada).
- ^ Roald Tagle, Lutz Hecht: Geochemical identification of projectiles in impact rocks . (PDF) In: The University of Arizona, Meteoritics & Planetary Science Archives . 41, No. 11, 2006, pp. 1721-1735.
- ^ Galen R. Gisler: Tsunamis from asteroid impacts in deep water. Archived from the original on January 11, 2012. (PDF) In: Physics of Geological Processes, University of Oslo . March 2007. Retrieved May 21, 2015.
- ^ E. Buchner, WH Schwarz, M. Schmieder, M. Trieloff: Establishing a 14.6 ± 0.2 Ma age for the Nördlinger Ries impact (Germany) - A prime example for concordant isotopic ages from various dating materials . In: Geophysical Research Letters . 45, No. 4, April 2010, pp. 662-674. doi : 10.1111 / j.1945-5100.2010.01046.x .
- ↑ How Big was the Chesapeake Bay Crater . Imperial College, London. Department of Earth Science and Engineering.
- ↑ R. Coccioni, D. Basso, H. Brinkhuis, S. Galeotti, S. Gardin, p Monechi, p Spezzaferri: Marine biotic signal across a late Eocene impact layer at Massignano, Italy: evidence for long-term environmental perturbations ? . In: Terra Nova . 12, No. 6, December 2000, pp. 258-263. doi : 10.1046 / j.1365-3121.2000.00305.x .
- ↑ The Popigai Impact Crater in the Earth Impact Database . ( Memento from August 30, 2014 in the Internet Archive ) Planetary and Space Science Center, Department of Earth Sciences, University of New Brunswick (Canada).
- ↑ Becky Oskin: Russia's Popigai Meteor Crash Linked to Mass Extinction . livescience, June 13, 2014.
- ↑ Luigi Jovane, Fabio Florindo, Jaume Dinares-Turell: Environmental magnetic record of paleoclimate change from the Eocene-Oligocene stratotype section, Massignano, Italy . (PDF) In: Geophysical Research Letters . 31, 2004. doi : 10.1029 / 2004GL020554 .
- ↑ James Zachos, Mark Pagani, Lisa Sloan, Ellen Thomas, Katharina Billups: Trends, Rhythms, and Aberrations in Global Climate 65 Ma to Present . (PDF) In: Science . 292, No. 5517, April 2001, pp. 686-693. doi : 10.1126 / science.1059412 .
- ^ Madelaine Böhme : The Miocene Climatic Optimum: evidence from ectothermic vertebrates of Central Europe . (PDF) In: Palaeogeography, Palaeoclimatology, Palaeoecology . 195, No. 3-4, June 2003, pp. 389-401. doi : 10.1016 / S0031-0182 (03) 00367-5 .
- ↑ a b Shiming Wan, Wolfram M. Kürschner, Peter D. Clift, Anchun Li, Tiegang Li: Extreme weathering / erosion during the Miocene Climatic Optimum: Evidence from sediment record in the South China Sea . In: Geophysical Research Letters . 36, No. 19, October 2009. doi : 10.1029 / 2009GL040279 .
- ↑ Wolfram M. Kürschner, Zlatko Kvaček, David L. Dilcher: The impact of Miocene atmospheric carbon dioxide fluctuations on climate and the evolution of terrestrial ecosystems . In: pnas . 105, No. 2, 2007, pp. 449-453. doi : 10.1073 / pnas.0708588105 .
- ↑ Jennifer Kasbohm, Blair Schoene: Rapid eruption of the Columbia River flood basalt and correlation with the mid-Miocene climate optimum . (PDF) In: Science Advances . 4, No. 9, September 2018. doi : 10.1126 / sciadv.aat8223 .
- ↑ Edward Gasson, Robert M. DeConto, David Pollard, Richard H. Levy: Dynamic Antarctic ice sheet during the early to mid-Miocene . In: PNAS . 113, No. 13, March 2016, pp. 3459-3464. doi : 10.1073 / pnas.1516130113 .
- ^ AR Lewis, DR Marchant, AC Ashworth, SR Hemming, ML Machlus: Major middle Miocene global climate change: Evidence from East Antarctica and the Transantarctic Mountains . (PDF) In: Geological Society of America Bulletin . 119, No. 11/12, pp. 1449-1461. doi : 10.1130 / 0016-7606 (2007) 119 [1449: MMMGCC] 2.0.CO; 2 .
- ↑ Madelaine Böhme, Michael Winklhofer, August Ilg: Miocene precipitation in Europe: Temporal trends and spatial gradients . (PDF) In: Palaeogeography, Palaeoclimatology, Palaeoecology . 304, No. 3-4, May 2011, pp. 212-218. doi : 10.1016 / j.palaeo.2010.09.028 .
- ↑ Madelaine Böhme, August Ilg, Michael Winklhofer: Late Miocene “washhouse” climate in Europe . (PDF) In: Earth and Planetary Science Letters . 275, No. 3-4, November 2008, pp. 393-401. doi : 10.1016 / j.epsl.2008.09.011 .
- ^ M. Prevedi, BG Liepert, DT Peteet, J. Hansen, DJ Beerling, AJ Broccoli, S. Frolking, JN Galloway, M. Heimann, C. Le Quéré, S. Levitus, V. Ramaswamy: Climate sensitivity in the Anthropocene . (PDF) In: Earth System Dynamics Discussions . No. 2, September 2011, pp. 531-550. doi : 10.5194 / esdd-2-531-2011 .
- ↑ a b Mark Pagani, Zhonghui Liu, Jonathan LaRiviere, Ana Christina Ravelo: High Earth-system climate sensitivity determined from Pliocene carbon dioxide concentrations . (PDF) In: Nature Geoscience . 3, 2010. doi : 10.1038 / ngeo724 .
- ↑ James Hansen, Makiko Sato, Gary Russell, Pushker Kharecha: Climate Sensitivity, Sea Level, and Atmospheric CO 2 . In: NASA Goddard Institute for Space Studies and Columbia University Earth Institute, New York . 2013. arxiv : 1211.4846 . doi : 10.1098 / rsta.2012.0294 .
- ^ D. Garcia-Castellanos, A. Villaseñor: Messinian salinity crisis regulated by competing tectonics and erosion at the Gibraltar Arc . (PDF) In: Nature . No. 480, 2011, pp. 359-363. doi : 10.1038 / nature10651 .
- ↑ C. Montes, A. Cardona, C. Jaramillo, A. Pardo, JC Silva, V. Valencia, C. Ayala, LC Pérez-Angel, LA Rodriguez-Parra, V. Ramirez, H. Niño: Middle Miocene closure of the Central American Seaway . (PDF) In: Science . 348, No. 6231, April 2015, pp. 226-229. doi : 10.1126 / science.aaa2815 .
- ↑ Aaron O'Dea, Harilaos A. Lessios, Anthony G. Coates, Ron I. Eytan, Sergio A. Restrepo-Moreno, Alberto L. Cione, Laurel S. Collins, Alan de Queiroz, David W. Farris, Richard D. Norris, Robert F. Stallard, Michael O. Woodburne, Orangel Aguilera, Marie-Pierre Aubry, William A. Berggren, Ann F. Budd, Mario A. Cozzuol, Simon E. Coppard, Herman Duque-Caro, Seth Finnegan, Germán M . Gasparini, Ethan L. Grossman, Kenneth G. Johnson, Lloyd D. Keigwin, Nancy Knowlton, Egbert G. Leigh, Jill S. Leonard-Pingel, Peter B. Marko, Nicholas D. Pyenson, Paola G. Rachello-Dolmen, Esteban Soibelzon, Leopoldo Soibelzon, Jonathan A. Todd, Geerat J. Vermeij, Jeremy BC Jackson: Formation of the Isthmus of Panama . In: Science Advances . 2, No. 8, August 2016. doi : 10.1126 / sciadv.1600883 .
- ↑ Gerald H. Haug, Lloyd D. Keigwin: How the Isthmus of Panama Put Ice in the Arctic. Drifting continents open and close gateways between oceans and shift Earth's climate . In: oceanus magazine, Woods Hole Oceanographic Institution . 42, No. 2, April 2004.
- ↑ Gerald Haug , Ralf Tiedemann, Rainer Zahn: From the Panama Isthmus to the Greenland Ice Spectrum of Science, November 1998.
- ↑ a b Peter Marcott, Jeremy D. Shakun, Peter U. Clark, Alan C. Mix: A Reconstruction of Regional and Global Temperature for the Past 11,300 Years . (PDF) In: Science . 6124, No. 269, March 2013, pp. 1198-1201. doi : 10.1126 / science.1228026 .
- ↑ Darrell Kaufman, Nicholas McKay, Cody Routson, Michael Erb, Christoph Dätwyler, Philipp S. Sommer, Oliver Heiri, Basil Davis: Holocene global mean surface temperature, a multi-method reconstruction approach . In: Nature Scientific Data . June 7, 2020. doi : 10.1038 / s41597-020-0530-7 .
- ^ Susan L. Hautala, Evan A. Solomon, H. Paul Johnson, Robert N. Harris, Una K. Miller: Dissociation of Cascadia margin gas hydrates in response to contemporary ocean warming . (PDF) In: Geophysical Research Letters . 41, No. 23, December 5, 2014, pp. 8486-8494. doi : 10.1002 / 2014GL061606 .
- ^ IPCC. Climate Change 2013 - The Physical Science Basis . Summary for Policymakers, Technical Summary and Frequently Asked Questions.
- ↑ Hans-Joachim Schellnhuber et al .: Turn Down the Heat - Why a 4 ° C Warmer World Must be Avoided. (German: The 4 ° report. Why a world four degrees warmer must be prevented ). Report for the World Bank , prepared by the Potsdam Institute for Climate Impact Research and Climate Analytics, November 2012.
- ↑ TM Lenton, H. Held, E. Kriegler, JW Hall, W. Lucht, S. Rahmstorf, HJ Schellnhuber: Tipping elements in the Earth's climate system . In: Proceedings of the National Academy of Sciences . 105, No. 6, February 12, 2008, pp. 1786-1793. ISSN 0027-8424 . doi : 10.1073 / pnas.0705414105 .
- ↑ J. Hansen, M. Sato, P. Hearty, R. Ruedy, M. Kelley, V. Masson-Delmotte, G. Russell, G. Tselioudis, J. Cao, Eric Rignot , I. Velicogna, E. Kandiano, K. von Schuckmann, P. Kharecha, AN Legrande, M. Bauer, K.-W. Lo: Ice melt, sea level rise and superstorms: evidence from paleoclimate data, climate modeling, and modern observations that 2 ° C global warming is highly dangerous . (PDF) In: Atmospheric Chemistry and Physics (Discussions) . 15, No. 14, 2015, pp. 20059–20179. doi : 10.5194 / acpd-15-20059-2015 .
- ↑ Quote Stefan Rahmstorf, in: Paläoklima: The whole Holozän . ScienceBlogs (KimaLounge), June 17, 2013.
- ↑ Samuel B. Fey, Adam M. Siepielski, Sébastien Nusslé, Kristina Cervantes-Yoshida, Jason L. Hwan, Eric R. Huber, Maxfield J. Fey, Alessandro Catenazzi, Stephanie M. Carlson: Recent shifts in the occurrence, cause, and magnitude of animal mass mortality events . In: pnas . January 2015. doi : 10.1073 / pnas.1414894112 .
- ↑ January Zalasiewicz, Mark Williams, Will Steffen, Paul Crutzen: The New World of the Anthropocene . (PDF) In: Environmental Science & Technology . 44, No. 7, 2010.
- ↑ Will Steffen, Jacques Grinevald, Paul Crutzen, John McNeill: The Anthropocene: conceptual and historical perspectives . In: The Royal Society, Philosophical Transactions A . 369, No. 1938, January 2011. doi : 10.1098 / rsta.2010.0327 .
- ↑ quaternary.stratigraphy.org: Working Group on the 'Anthropocene'. Subcommission on Quaternary Stratigraphy.
- ↑ Colin Waters, Jan Zalasiewicz: Newsletter of the Anthropocene Working Group . Volume 5: Report of activities 2013–14, September 2014.
- ↑ Meera Subramanian: Anthropocene now: influential panel votes to recognize Earth's new epoch . In: Nature . May 2019. doi : 10.1038 / d41586-019-01641-5 . accessed on May 24, 2019
- ^ Subcommission on Quaternary Stratigraphy: Working Group on the 'Anthropocene' - Results of binding vote by AWG. accessed on May 26, 2019.
- ↑ Susan Solomon, Gian-Kasper Plattner, Reto Knutti , Pierre Friedlingstein: Irreversible climate change due to carbon dioxide emissions . In: pnas . 106, No. 6, February 2009, pp. 1704-1709. doi : 10.1073 / pnas.0812721106 .
- ↑ Victor Brovkin: By time machine into the history of the climate . ( Memento from May 5, 2015 in the Internet Archive ) Max Planck Institute for Meteorology.
- ^ Richard E. Zeebe: Time-dependent climate sensitivity and the legacy of anthropogenic greenhouse gas emissions . In: pnas . 110, No. 34, August 2013, pp. 13739-13744. doi : 10.1073 / pnas.1222843110 .
- ↑ Understanding plate motions . Information from the United States Geological Survey (USGS) on plate tectonics.
- ↑ Masaki Yoshida, M. Santosh: Supercontinents, mantle dynamics and plate tectonics: A perspective based on conceptual vs. numerical models . (PDF) In: Earth-Science Reviews . 105, No. 1-2, March 2011, pp. 1-24. doi : 10.1016 / j.earscirev.2010.12.002 .
- ↑ Holger Kroker: Protocol of an inevitable catastrophe . planet Earth. World of geosciences.
- ↑ Christine Bounama, Werner von Bloh, Siegfried Franck: The end of the spaceship earth (PDF). Spectrum of Science, October 2004 (accessed December 10, 2014)
- ^ I.-Juliana Sackmann, Arnold I. Boothroyd, Cathleen E. Cramer: Our Sun. III. Present and Future . In: The Astrophysical Journal . 418, Nov 1993, pp. 457-468. bibcode : 1993ApJ ... 418..457S .
- ↑ S. Franck, C. Bounama, W. von Bloh: Causes and timing of future biosphere extinctions . (PDF) In: Biogeosciences, European Geosciences Union (EGU) . March 2006, pp. 85-92.