Mass extinction
A mass extinction , and faunal or faunal called, is one in geologically take-find short periods of a few thousand to several hundred thousand years great extinctions that from the normal so-called background extinction as clearly stands out as by mass extinctions : extraordinary, dramatic losses of individuals of one or of many populations of Kind within a short time.
In the course of recent geological history , more or less pronounced faunal changes have taken place every ten million years. These events, which can be traced on the basis of the fossil record , historically served as the basis for drawing the boundaries between the Phanerozoic epochs in the geological time scale (see also evolutionary history ). From the 1970s onwards, geological, paleontological and paleoclimatological findings increasingly showed that these faunal changes are due to drastic increases in extinction rates, which occurred in a relatively narrow geological time window.
The advances in radiometric dating and detection methods made in recent decades have led to a significant increase in measurement accuracy. This made it possible to narrow down various mass extinctions more precisely, to describe them relatively comprehensively and to document previously unknown biological and ecological crises in the course of the earth's history. There is no clear consensus in science on the definition of mass extinction . Some publications only use the term in the case of species loss of 75 percent or more, on the other hand, every major cut in biological diversity is often referred to as mass extinction .
Various panels of experts assume that the sixth mass extinction in the history of life has already begun. According to the Global Report of the World Biodiversity Council of the UN organization IPBES published in May 2019 , one million animal and plant species could be threatened with extinction within the next few decades. A direct comparison of this mass extinction with earlier geological crisis times is problematic insofar as anthropogenic factors currently dominate. The IPBES report names the reasons for the current extinction - in order of magnitude - the loss of habitat , changes in land use , hunting and poaching , climate change , environmental toxins and the occurrence of neobiota .
Research history background
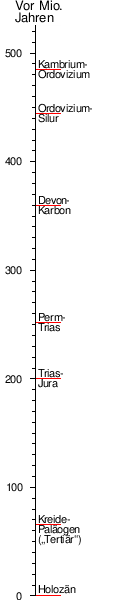
From a geoscientific perspective, June 6, 1980 is a historic date. The issue of the journal Science published on that day contained an article with the title Extraterrestrial Cause for the Cretaceous-Tertiary Extinction as its main contribution (German: Extraterrestrial cause for the Cretaceous Tertiary Extinction ). The authors were the physicist and Nobel Prize winner Luis Walter Alvarez , his son, the geologist Walter Alvarez , and team members Frank Asaro and Helen W. Michel, who are active in the field of nuclear chemistry . In the words of the American paleontologist David M. Raup , the Alvarez study hit “like a bombshell” in science. The hypothesis of an asteroid impact on the Cretaceous-Tertiary boundary (today Cretaceous-Paleogene boundary ), which is based on the detection of an Iridium anomaly and which, among other things , should have caused the extinction of the dinosaurs , sparked protracted controversial discussions at conferences and in the Specialist literature. Part of the rejection resulted from the refusal of many researchers to accept a scenario that had all the characteristics of a catastrophism long believed to have been overcome .
It had been known since the early 19th century that there was almost always a comprehensive change of fauna at the borders or transitions of the geochronological periods. These biological crises were explained - in accordance with the principle of gradual change ( actualism ) - until well into the 20th century, mostly with the assumption of geological processes such as sea level fluctuations and continental shifts and the associated climate and environmental changes over periods of millions of years. Although well-known scientists as the American Nobel laureate expressed Harold Urey or German paleontologist Otto Schindewolf already in the 1950s the assumption that some of extinction events with the radiation bursts near-Earth with a comet or supernova might be related, but their ideas found no response in the professional world.
In contrast, the Alvarez et al. Postulated catastrophe on the Cretaceous-Paleogene border to a gradual paradigm shift with regard to geological times of upheaval as well as to an increasingly intensive analysis of potential mass extinctions, including research areas such as geochemistry , mineralogy , paleontology , paleoclimatology , sedimentology , (event) stratigraphy , volcanology , Geophysics and paleomagnetism . As a result of the interdisciplinary cooperation, multi-causal models for mass extinctions were developed, which, in addition to the conventional explanatory models, also take into account additional factors such as anoxia (the lack of oxygen), ocean acidification , rapid fluctuations in the concentration of greenhouse gases or the influence of magmatic large provinces . The specialist literature on this topic increased almost tenfold between 1984 and 2004, with the result that mass extinctions do not necessarily have to be linked to long-term geological processes, but often took a catastrophic and temporally limited course. Since the beginning of the 21st century, there has also been a growing body of evidence to support the assumption that many biodiversity crises were linked to serious climatic changes and their consequences. On this basis, a “ranking” of the most devastating mass extinctions during the last 541 million years was created.
The so-called "Big Five" (also Big Five ) include in detail:
- the Ordovician mass extinction 444 million years ago
- the Kellwasser event in the Upper Devonian 372 million years ago
- the event on the Permian Triassic border 252 million years ago
- the time of crisis at the Triassic-Jura border 201 million years ago
- the mass extinction at the Cretaceous-Paleogene border 66 million years ago
In the case of the big five , the respective species decline was probably 70 to 75 percent or sometimes more. Although a revision of this structure or a reassessment in favor of other mass extinctions is occasionally suggested (particularly with regard to the Kellwasser event ), the Big Five are still the unofficial guide in depicting the most serious biological crises during the Phanerozoic . This is not only expressed in the relevant specialist literature, but is also frequently discussed in popular science books and articles.
Probable causes of mass extinction
The causes of mass extinctions are a much discussed topic in the various disciplines of geosciences. The actual triggers, the ultimate causes , are now primarily the volcanism of magmatic large provinces and impacts of small cosmic bodies. From these triggering events, attempts are made to find explanations for extinction patterns via sometimes complex causal chains to immediate, proximate causes. Rapid climate changes, ocean acidification and marine anoxia - among others - play an important role as immediate factors that could have extinguished en masse species.
The environmental situation in which the triggering event occurs also seems to be of great importance. The vegetation can influence the composition of the oceans and the atmosphere through its influence on weathering and erosion processes as well as organisms in general through their metabolism. The position and arrangement of land masses is also mentioned as an important boundary condition for the effectiveness of ultimate causes of extinction (their change sometimes also as the actual trigger). According to a recently developed hypothesis, the pronounced continentality of the 138 million km² supercontinent Pangea was a main reason why the biosphere was particularly vulnerable to the discharge of volcanic gases and ash at that time - there was no negative feedback that would have stabilized the earth system. After the dissolution of Pangea, the higher rate of weathering on the fragmented continents and the higher productivity of carbon-binding organisms would have withdrawn more carbon dioxide from the atmosphere and thus counteracted the consequences of volcanism and thus ultimately a mass extinction.
Volcanism
Volcanic eruptions of the category VEI-6 or VEI-7 on the volcanic explosion index cause a more or less pronounced cooling effect over several years due to their emission of volcanic ash . With the help of high-altitude currents (strong wind bands), the released aerosols are distributed in the stratosphere - the second lowest layer of the atmosphere - where they dampen the transmitted solar radiation to a considerable extent through absorption , scattering and reflection .
The super volcanoes , which belong to the highest category VEI-8, can cause decades of changes in global temperature due to their ejection quantity of over 1,000 km³ of lava , ash and aerosols ( tephra ) and trigger a volcanic winter due to the particles distributed in the atmosphere . All known super volcanoes did not leave behind an eruption, due to the size of their magma chamber , not volcanic cones, but huge calderas . The discharge of pyroclastic material destroyed all life within a radius of at least 100 km, and the area covered with volcanic ash was probably millions of square kilometers. Events of this magnitude resulted in mass extinctions in the affected regions with a decrease in biodiversity. More than 40 such catastrophes have so far been clearly proven in recent geological history, including the La Garita eruption in the early Oligocene around 38 to 36 million years ago (= 38 to 36 mya), the last VEI-8 eruption of the Yellowstone Hotspots 640,000 years ago and the Toba event on Sumatra 74,000 years ago. However, permanent climatic and ecological consequences from super volcanoes have not been proven.
On the other hand, so-called Magmatic Large Provinces (English Large Igneous Provinces ) were several reasons for serious and relatively rapid global warming and the associated mass extinction. This involved the large-volume escape of igneous rocks from the earth's mantle , mainly in the form of flood basalts , which over the course of several hundred thousand years sometimes spilled over an area of millions of km². Depending on the extent and duration of the volcanic activity, considerable amounts of carbon dioxide that have an impact on the climate were released, including hydrogen chloride , fluorine and sulfur dioxide to a significant extent . Since the carbon dioxide emissions in these cases were significantly higher than the aerosol emissions, igneous large provinces were not only responsible for a global rise in temperature, but in extreme cases set an additional warming spiral in motion with the help of the strong greenhouse gas methane or methane hydrate from oceanic deposits. It is very likely that many mass extinctions in the history of the earth are directly related to the large-scale outflow of flood basalts and the subsequent destabilization of marine biotopes. The Magmatic Large Provinces , which influenced the environment and biodiversity in different ways , include the Siberian Trapp ( Perm-Triassic border , 252 mya ), the Dekkan-Trapp in today's West India ( Chalk-Paleogene border , 66 mya) as well the North American Columbia plateau basalt ( Middle Miocene , main activity 16.7 to 15.9 mya).
Climate change
A climate change on a global level is based on a radiative forcing that transforms the earth's climate system from a stable thermal-radiative equilibrium into a new equilibrium. The radiative forcing results from changes in the atmospheric concentrations of greenhouse gases such as carbon dioxide (CO 2 ), methane (CH 4 ) and water vapor (H 2 O), from the varying solar radiation due to the Earth's orbit parameters ( Milanković cycles ) changing over longer periods of time and from the Reflectivity ( albedo ) of the earth's surface including the oceans. Since the components of the earth system form a complex unit, climate change on a monocausal basis is practically impossible, since every partial change affects the overall structure. This takes place predominantly in the form of positive feedback, that is, the system moves further and further away from the original equilibrium due to a large number of cumulative interactions (feedback effects).
In science, a distinction is usually made between the two basic conditions warm climate and cold climate . In order to completely cover the entire climatic spectrum, there is sometimes a differentiation into ice house , cool greenhouse , warm greenhouse and heat house ( icehouse , cool greenhouse, warm greenhouse, hothouse) . According to this, every climatic condition has its own characteristics that differ significantly from the others. In addition, several tipping points are postulated at the transition from ice house to cool greenhouse and from warm greenhouse to hot house , which transform the earth's climate system into a new and partially irreversible state ( tipping elements in the earth system ).
With regard to the mass extinctions in the history of the earth, abrupt climate changes were almost always one of the main causes. These could occur within a few days (as at the Cretaceous-Paleogene border ) and geologically cover very short periods of a few thousand or ten thousand years (as in most magmatic large provinces ). Overall, the respective environmental changes took place so quickly that many ecosystems reached their adaptation limits when transitioning from one climatic condition to the next and collapsed. Serious biological crises correlated several times in the last 540 million years with a cooling phase (with a worldwide temperature drop of 4 to 5 ° C), but more often with strong warming in the range of 5 to 10 ° C. In the latter case, a bundle of side effects (decline in vegetation, outgassing of toxins and pollutants, oxygen deficits, acidification of the oceans, etc.) contributed to further destabilizing the terrestrial biosphere.
Oceanic anoxic events
Oceanic anoxic events (English Oceanic anoxic events , abbreviated OAE ) based on an oxygen deficit (less than 2 mg / l) especially in tropical shallow water seas. Only the top mixed water layer was excluded from this. An indicator of the occurrence of anoxic events are the marine black shale horizons that formed in an oxygen-free environment , which formed from digested sludge at the bottom of the ocean and which were frequently detected in both Paleozoic and Mesozoic sedimentary layers .
A significant hypoxic or anoxic event is usually based on the following conditions:
- an atmospheric carbon dioxide concentration in the range of about 1,000 ppm
- a worldwide subtropical to tropical climate with simultaneous warming of the oceans with correspondingly reduced oxygen binding (from the Ordovician , however, an OAE during a global cooling is documented)
- a greatly reduced or stalled deep water circulation between the polar regions and the equator
- the overfertilization of the seas with continental weathering products due to the rapid erosion processes in a warm climate
A large part of the anoxic events was probably partly caused or at least intensified by the long-lasting CO 2 and sulfur dioxide emissions from magmatic large provinces . OAEs usually extended over a period of 300,000 to 800,000 years, but in exceptional cases could last for over 2 million years or, as during the crisis in the Upper Devonian, occur several times in a row within less than 100,000 years. Regular side effects were an algal bloom that turned the sea water green and the mass multiplication of sulfur bacteria (chlorobia). The bacterial reduction of sulphate resulted in highly toxic hydrogen sulphide (H 2 S), which not only accumulated in the oceans but also in the atmosphere, probably also permanently damaging the ozone layer . According to more recent findings, there have been several hydrogen sulfide-induced mass extinctions in the history of the earth, with particularly serious consequences during the biological crisis on the Permian-Triassic border . The odor of rotten eggs typical of this gas was almost omnipresent at the time, and the lethal effect of hydrogen sulfide killed thousands of marine species as well as many representatives of the land fauna.
Impact events
Impact -EVENTS as the impact of larger asteroids or comets may destabilize not only the biosphere to a considerable extent and mass extinction like the one at the Cretaceous-Paleogene boundary trigger, but also change the climate in the short term (abrupt onset Impaktwinter , in some cases, followed by excessive heat through released greenhouse gases ). There are around 180 impact craters on Earth, more than 5 to 10 km in size, of which only around two dozen are in oceanic sediments. Since sea floors are constantly "diving" into the depths of the earth's mantle due to the plate-tectonic process of subduction , and on the other hand are constantly being formed at the spreading zones , the average age of the oceanic crust is around 80 million years. There is therefore reason to believe that many impact events will remain unknown and can no longer be detected.
The thesis of an asteroid impact on the Cretaceous-Paleogene boundary, published by father and son Alvarez in 1980, found rapid acceptance after initial resistance and was able to establish itself as mainstream scientific within a decade. When a crater of the appropriate size and age was discovered on the Mexican Yucatan peninsula in 1991 , that was not only a confirmation of the Alvarez thesis, but also caused the number of impact supporters to skyrocket. According to the geoscientist and biologist Peter Ward , many researchers by the turn of the millennium “had a fool of the idea” that almost all mass extinctions were caused by celestial bodies that collided with the earth. The main focus was on the Permian Triassic Crisis , which was supposed to be related to structures such as the hypothetical Wilkesland crater or the Bedout formation off Australia. In the meantime, these assumptions have not been confirmed and have been partially refuted. Apart from the impact at the Cretaceous-Palaeogene transition, no other “ global killer ” could be clearly identified in the course of the Phanerozoic . However, this does not rule out that future geological discoveries may revise the previous picture.
Hypothetical extraterrestrial causes
Gamma-ray bursts

Since the first secure evidence of a gamma-ray burst ( gamma-ray burst , abbreviated GRB ) in 1973 gamma-ray burst scenarios are discussed as triggers of previous environmental crises, and especially the Ordovician mass extinction is the focus. There are several explanations for the origin of gamma-ray bursts, mostly in connection with the formation of a neutron star or a black hole . The duration of a gamma-ray burst is often in the order of seconds, with considerable and strongly concentrated amounts of energy being released during this period. Therefore, at least theoretically, there is the possibility that a radiation outbreak that hits the earth directly has the potential to cause lasting damage to earthly life. However, immediate biological effects would be limited as the atmosphere would absorb most of the radiation. A hazard that should not be underestimated, on the other hand, would be the formation of nitrogen oxides with the resulting destruction of the ozone layer . The probability of such an event, however, is extremely low.
Supernovae
Occasionally, a near-Earth supernova is assumed to be the cause of various mass extinctions . Several supernovae signatures in the form of the iron isotope 60 Fe are known from the past . This isotope that can not occur under terrestrial conditions, has a half-life of 2.62 million years ago and was among others in sediments of the early Quaternary ( Gelasian stage) before 2.2 million years and the Lower Pleistocene (Calabrium) 1, Discovered 5 million years ago. However, this evidence comes from recent geological history. For gamma-ray bursts and supernovae, it is equally true that a detection of cosmic radiation influences in Palaeozoic and Mesozoic rock formations is not possible with the instruments currently used.
Nemesis hypothesis
The nemesis hypothesis (based on the Greek goddess of "just anger") was the assumption, which was popular and much discussed mainly in the 1980s, that the sun is orbited by a brown dwarf or a faint dwarf star at a distance of 1 to 3 light years. In its original form, the hypothesis was based on the scenario that Nemesis during its about 26 to 27 million years lasting orbital period on the sun next point ( Perihel ) when crossing a interspersed with rock dust and ice cosmic region ( Oort cloud a) due to their gravitational effect Series of comets into the inner solar system and thus also towards Earth. This leads to an accumulation of impact events for a very limited time and thus to mass extinctions that take place regularly. The (apparent) periodicity of biological crises in the history of the earth was first postulated by the paleontologists David M. Raup and Jack Sepkoski on the basis of statistical analyzes and formed one of the bases for the elaboration of the hypothesis. Well-founded objections to the nemesis hypothesis were made both from the astronomical and astrophysical as well as from the geoscientific side. There is currently no evidence of a companion star for the sun, and on the other hand the 26 million year cycle of mass extinctions is generally doubted. According to a study published in 2017, the increase in asteroid or comet impacts on Earth in some epochs happened purely by chance and without any apparent periodicity.
Dating and analytical methods
The progress made in the study of extinction events in the last few decades is based in large part on the application and further development of radiometric dating . This age determination method measures the decay rates of naturally occurring isotopes and the isotope ratio . When using a modern mass spectrometer , sample weights of one billionth of a gram are currently often sufficient to achieve precise results. There are a number of different dating methods in the geosciences, the three following methods being the most commonly used:
- Due to their heat resistance and their stable lattice structure due to the radioactive nuclides they contain (such as 235 U, 238 U or 232 Th), zircon crystals are suitable for geochronological dating over the entire history of the earth. The error tolerances in this form of absolute age determination of mass extinctions and geological events are only a few 10,000 years when using this method from the Silurian to the Cretaceous .
- The 40 Ar / 39 Ar-dating is a modified and more accurate method of the conventional K-Ar dating and is widely used for some time to determine the age of minerals and rocks.
- The radiocarbon method , also called 14 C dating , is a method for determining the age of organic substances. Due to its limited range of application from 300 to a maximum of 57,000 years, 14 C dating is only suitable for the most recent geological history. In addition, the increasing anthropogenic CO 2 emissions are currently leading to a significant reduction in the 14 C content in the atmosphere. This effect will probably make future dating on this basis considerably more difficult or falsify it.
Several "palaeothermometers" are used to reconstruct the climate of past periods:
- δ 18 O (Delta-O-18) describes the ratio of the stable oxygen isotopes 18 O / 16 O. This versatile measuring method is suitable for the determination of precipitation temperatures and also serves as an indicator of processes of isotope fractionation such as methanogenesis . Research uses 18 O / 16 O data as a temperature proxy for fossil corals and foraminifera, as well as ice cores , dripstones and freshwater sediments.
- TEX86 (tetraether index of 86 carbon atoms) describes a biogeochemical method for determining the sea surface temperature of earlier epochs. For this purpose, the cell membrane of certain marine protozoa is analyzed. Temporal scope: Jurassic, Cretaceous and entire Cenozoic Era (around the last 200 million years).
One of the most important detection methods in geochemistry and paleoclimatology is based on the properties of the carbon isotope 13 C:
- δ 13 C (Delta-C-13) is the measure of the ratio of the stable carbon isotopes 13 C / 12 C between a sample and a defined standard. The δ 13 C analysis allows the determination of the atmospheric carbon dioxide concentration in the various geological epochs and allows conclusions to be drawn about disturbances or anomalies of the carbon cycle . The release of large amounts of methane, such as during the Paleocene / Eocene temperature maximum , also has significant effects on the global δ 13 C signature.
Chronology of the mass extinctions
Precambrian
Proterozoic (2,400 and 717/640 million years ago)
In the early Proterozoic , in the course of the Great Oxygen Catastrophe, what was probably the most extensive mass extinction of the entire Precambrian took place, during which almost all anaerobic life forms were extinguished. At the beginning of this era, the terrestrial atmosphere had a relatively high concentration of methane, but only very low levels of free oxygen. It is very likely that cyanobacteria already existed more than 3 billion years ago , using oxygen-phototrophic photosynthesis and releasing a significant amount of oxygen as a "waste product" of their metabolism, which, however, occurs during the oxidation of organic compounds, hydrogen sulfide and bivalent iron ions Fe 2+ in trivalent iron ions Fe 3+ has been completely consumed. This process was directly related to the formation of bands of iron ore linked (English Banded Iron formation ), an iron-containing, deposited in Precambrian sedimentary rock which could not be formed under the present conditions geochemical more. At the end of this intensive oxidation phase, the excess of free oxygen accumulated in both the atmosphere and the oceans. The latter led to the mass extinction of obligately anaerobic organisms, i.e. organisms that were dependent on a low-oxygen environment and almost all of which fell victim to the toxicity of oxygen. Due to its oxidative properties, oxygen in the atmosphere caused the methane concentration to collapse with the help of UV radiation .
This turning point resulted in serious climate change. It is very likely that the 300 million year long Paleoproterozoic Glaciation (also known as the Huronic Ice Age ) was the direct consequence of methane reduction and oxygen increase. Geological evidence of this glacial phase can be found in North America, Scandinavia, India and southern Africa, among others, and point to a global cold snap. In addition, some studies postulate at least one snowball earth event that resulted in complete glaciation of the earth, including the equatorial zone and oceans. Several global glaciation cycles are also assumed for the Sturtic Ice Age (717 to 660 mya) and for the Marino Ice Age (640 to 635 mya) in the Neoproterozoic . It is mainly assumed that the natural carbon cycle almost came to a standstill and that biomass production in the oceans remained at a very low level. However, like most of the details of the snowball scenario, these assumptions are controversial in science.
Ediacarian-Cambrian boundary (541 million years ago)

The Ediacara fauna , which existed around 580/570 to 540 million years ago , formed relatively poor, but distinctly heterogeneous biotopes of multicellular animals (Metazoa), which, apart from some deep-sea forms, form the continental shelves or the bottom of the benthic ( bottom -dwelling) way of life Populated shallow seas. Since the Ediacaran fauna has no direct successor in its phylogenetic history, its taxonomic classification is still unclear or controversial to this day. It can be considered certain that the typical representatives of this fauna had little or no mobility (for example, as fixed, fern-like organisms), fed on dissolved organic substances or by breaking down microbial mats, apparently had no skeletal parts and at the transition from Ediacarian disappeared completely by the Cambrian (with a significant reduction in biodiversity already taking place before).
There are two possible main causes for this change of fauna being discussed in science: on the one hand the serious influence of abiotic environmental-changing factors (which would mean a "real" mass extinction), on the other hand the displacement of the Ediacara fauna by the superior competition of "modern" Cambrian species. The fact that the late Neoproterozoic was an era of intense plate tectonic processes speaks in favor of the first assumption . Almost simultaneously with the breakup of the hypothetically postulated supercontinent of Pannotia , several cratons merged to form the major continent Gondwana, combined with extensive folds such as the 8,000 km long mountain range of the Transgondwanan Supermountain . This resulted in strong volcanism , “over-fertilization” of the oceans with continental weathering products due to an extremely high rate of erosion and probably significant fluctuations in climate and sea level. The existence of black slate sediments and the formation of hydrogen sulfide in the oceans of that time have been proven . Both points to a shortage of oxygen and the formation of anoxic zones. Furthermore it was based on the isotopic analysis δ 13 C a global disturbance of the organic carbon cycle are detected. This scenario is countered by the fact that comparable geochemical and geotectonic processes as in the Ediacarian-Cambrian border area had already occurred and that the extinction of the Ediacarian fauna was essentially due to the rapid spread of Cambrian organisms, either in direct form by free-swimming predators or indirectly by burrowing ones and burrowing animals ( bioturbation ), which in this way - in the course of a more gradual transition - destroyed the livelihoods of the Ediacara species.
Overview of Paleozoic and Mesozoic biodiversity crises
With the beginning of the Phanerozoic about 541 million years ago, there was a significant increase in biodiversity, and fossil remains other than microscopic remains have been detected since then. The greatest extinction events occur in the first two eras , the Paleozoic (Earth Age) and the Mesozoic (Earth Middle Ages).
Name / epoch | Dating | Approximate species loss | Probable megavolcanism | Temperature anomalies 1) | Anoxic events |
---|---|---|---|---|---|
Middle Cambrian | 510 mya | 50%? | Kalkarindji Volcanic Province | Not quantified | Yes |
Upper Ordovician | 444 mya | Up to 85% | - | Cooling down to ice age climate (approx. -5 ° C), glaciations in the southern hemisphere | Yes |
Kellwasser event | 372 mya | 65 to 75% | Viluy-Trapp? | Brief heating peaks up to +9 ° C | Yes |
Hangenberg event | 359 mya | Up to 75% | - | Rapid change from warm and glacial phases, serious lowering of the sea level | Yes |
Capitanium | 260 mya | By 60% | Emeishan Trapp | Probably no pronounced climatic fluctuations | Yes (regional?) |
Permian Triassic border | 252 mya | 75% on land, 95% in the ocean | Siberian trap | Warming of +10 ° C, in the Lower Triassic (Smithium / Spathium) +6 ° C | Yes |
Triassic-Jura border | 201 mya | By 70% | Central Atlantic Magmatic Province | Warming by +4 to +6 ° C | Yes |
Chalk-Paleogene border | 66 mya | Up to 75% | Dekkan Trapp | Brief warming after impact winter (+4 ° C in the seas, +6 to +8 ° C on the mainland) | ? |
Paleozoic (ancient times)
Cambrian (about 510, 502 and 485 million years ago)
The Cambrian was an epoch with in some cases greatly increased volcanism, with average temperatures of 20 ° C or above and an atmospheric CO 2 concentration of around 5000 ppm. These factors had a lasting effect on the chemical composition of the seawater, so that the oceanic communities often reached their limits due to sulfur dioxide input, oxygen shortage and acidification and the associated drop in pH .
The rapid increase in biodiversity in the wake of the Cambrian Explosion led to a rapid increase in the background extinction , which reached a very high level as a permanent feature of biological evolution in the first half of the Paleozoic, and especially during the Cambrian . It is therefore difficult to differentiate between the natural exchange of species and mass extinction, especially since some Cambrian strata show considerable gaps with regard to fossil records. A possible trigger for the ecological crisis 510 million years ago was recently identified. According to this, the mass extinction is related to the Kalkarindji Volcanic Province in what is now Western Australia. The flood basalts released over a longer period of time covered an area of 2 million km² and caused (with simultaneous destabilization of the climate) through their outgassing a strong increase in anoxic zones in the oceans. It is estimated that half of all marine life fell victim to the disaster, including many trilobites (three-lobed crabs), conodonts and brachiopods ( arm pods ).
Upper Ordovician (about 444 million years ago)
In the Ordovician mass extinction , about 85 percent of the species and 60 percent of the genera died out, including most of the brachiopods . The trilobites survived, but their immense diversity also decreased significantly. Recent studies support the assumption that the colonization of the mainland by moss-like carpets of plants and early mushroom forms began as early as the Middle Cambrian and intensified in the Ordovician . The increasing vegetation cover deprived the soil of a number of elements such as calcium, magnesium, phosphorus and iron. This resulted in accelerated chemical weathering of the earth's surface with increased binding of atmospheric carbon dioxide and, in combination with other factors, a decrease in temperature of around 5 degrees. Due to the decreasing "buffer effect" of the greenhouse effect due to the gradual reduction of CO 2 , the sun irradiation, which is 4.5 percent lower than at present, must be taken into account ( solar constant in the Ordovician 1306 W / m², currently 1367 W / m²). The global cooling trend culminated in the so-called Andean-Sahara Ice Age around 460 million years ago . It reached its most concise expression at the same time as the height of mass extinction during the last Ordovician stage of the brain antium (from 445.2 mya) and covered large parts of the southern hemisphere. A contributing factor in this change in climate was the location of the greater continent of Gondwana in the immediate vicinity of the South Pole with extensive glaciations in the areas of what is now North Africa and Brazil.
In some current analyzes, the view is that it is not the glacial climate and the associated lowering of the sea level (with a decline in marine shallow water biotopes) that are the primary extinction factors, but that geochemical changes in connection with an oceanic anoxic event are toxic heavy metals such as arsenic, lead or Release manganese on the seabed. Using microfossils, a high incidence of malformed organisms can be proven at the time in question, which were clearly contaminated by toxic elements. In addition, the extensive reduction in trace elements could have played a serious role. The concentration of the vital trace element selenium at the transition from Ordovician to Silurian apparently only reached a fraction of the current level and was also at a minimum in some later mass extinctions such as those in the Upper Devonian. With regard to the possible destabilization of the oceans, this constellation implies a complex cause-and-effect structure, consisting, among other things, of the components increase or decrease in global oxygen and carbon dioxide values, oxidative erosion, anoxic conditions, sea level fluctuations as well as strongly varying nutrient inputs.
A study published in 2004 postulates an extraterrestrial cause for the mass extinction in the form of a gamma-ray flash . Although the rapid decimation of the organisms that inhabit the upper sea zones agrees with the radiation hypothesis, there is no further factual evidence.
Upper Devonian (approx. 372 and 359 million years ago)
system | series | step | ≈ age ( mya ) |
---|---|---|---|
higher | higher | higher | younger |
Devon | Upper Devonian | Family | 358.9 ⬍ 372.2 |
Frasnium | 372.2 ⬍ 382.7 |
||
Middle Devon | Givetium | 382.7 ⬍ 387.7 |
|
Eifelium | 387.7 ⬍ 393.3 |
||
Lower Devon | Emsium | 393.3 ⬍ 407.6 |
|
Pragium | 407.6 ⬍ 410.8 |
||
Lochkovium | 410.8 ⬍ 419.2 |
||
deeper | deeper | deeper | older |
The two paleontologists David M. Raup and Jack Sepkoski, among other things known for their hypothesis of periodic extinction , attempted to statistically quantify the “Big Five” for the first time in 1982. In the Upper Devonian they described three rather weak, but "sticking out from the background noise" extinction events (end of Givetium , end of Frasnium and end of Famennium ), of which the crisis on the Frasnium-Famennium border was the most striking, but not originally one of the Big Five counted. In the following decades, a large number of other crisis periods from the Emsium onwards were identified, such as that at the end of the Eifelium 387.7 million years ago, which were sometimes summarized under the name Central to Upper Devonian Biocrisis . On the other hand, the current research concentrates on two focal points with increasing knowledge: on the one hand on the Kellwasser event 372 million years ago, named after the Upper Devonian limestone layers of the Kellwassertal in the Upper Harz , and on the at least equally strong Hangenberg event 358.9 million years ago, whose name goes back to the so-called Hangenberg outcrops in the northeastern part of the Rhenish Slate Mountains .
- The Kellwasser event (internationally also Frasnian-Famennian Mass Extinction ) recorded species loss of up to 75 percent. The fauna groups of shallow tropical seas such as fish, corals , trilobites and a number of “ reef builders ” were particularly affected , which is why the number of coral reefs decreased significantly. In addition, the biodiversity of phytoplankton was so drastically reduced that the original biodiversity was only achieved again in the Jura .

- In geological tradition, this event corresponds to a worldwide spread of black shale horizons, which can be traced back to several Oceanic Anoxic Events (OAEs) . In the specialist literature there are various scenarios for the causes of the Upper Devonian crisis, such as a significantly increased effect of the Milanković cycles due to the falling carbon dioxide concentration , combined with a sudden overturning of the entire climate system. What is certain is that towards the end of the Devonian Sea level fluctuations occurred several times, which indicate an extremely short-term cycle of different cold and warm phases. The recently discovered mercury anomalies in the Kellwasser strata are considered to be an indicator of the involvement of megavolcanism in the mass extinction of that time and can possibly be assigned to the Viluy Trap in eastern Siberia.
- With the Hangenberg event on the threshold of the Carboniferous , a global warm climate phase that had ruled for more than 80 million years ended . While the Kellwasser event recorded several warming impulses, a clear and relatively abrupt cooling with extensive glaciations began in the southern and western regions of the greater continent Gondwana. The sea level fell by about 100 meters and the atmospheric CO 2 concentration was reduced by about 50 percent due to the massive deposition of organic carbon in black shale sediments. Due to the oxygen-poor conditions in the oceans, several ecosystems collapsed and ammonites , brachiopods (armpods), trilobites , conodonts , stromatopores , ostracods (mussel crabs), placodermi (armored fish) and early terrestrial vertebrates ( tetrapods ) collapsed . The crisis period, which was subdivided into several short-term cycles ( Lower, Middle and Upper Hangenberg Event ), lasted approximately 100,000 to 300,000 years. An accumulation of impact disasters such as the Australian Woodleigh impact , the Alamo impact in today's Nevada or the Swedish Siljan impact structure may also have been directly involved in the extinction waves and climatic changes in the Upper Devonian.
Permian (about 260 million years ago)
After the end of the Permocarbon Ice Age , which began in the Lower Carboniferous and ended in the course of the Permian , the trend towards a stable warm climate emerged, but this was interrupted by a significant environmental change 260 million years ago. The decline in biodiversity in tropical waters, which has long been known in research and assessed differently in terms of extent, was, according to recent studies, a global event that culminated in the so-called Capitanium mass extinction (named after the highest chronostratigraphic level of the Middle Permian or the Guadalupium series). A massive carbon dioxide and sulfur dioxide input into the oceans with the formation of anoxic zones and strong acidification of the sea water is assumed to be the cause. A connection with the simultaneously occurring flood basalts of the Emeishan Trapps in today's southern China (with the center in the province of Sichuan ) is considered very likely in science. The differently pronounced activity cycles of the trap probably lasted almost two million years and during this time covered an area of at least 250,000 km² with basaltic deposits.
Although the extinction event mainly affected the marine fauna of all climatic zones, the number of species also decreased significantly in terrestrial vertebrates, as in various groups of the Dicynodontia , Parareptiles and Pareiasauridae . In addition, the land vegetation in the vicinity of the Emeishan trap was significantly decimated due to atmospheric pollutants and an impairment of photosynthesis . According to current analyzes, over 60 percent of the species and 33 to 35 percent of the genera died out during the Capitanium crisis. It is not conclusively certain whether the event in the Middle Permian reached the extent of the Big Five , but there is increasing evidence that this may well be the case.
Permian Triassic boundary (approx. 252 million years ago)
The largest mass extinction of the Phanerozoic occurred on the Permian-Triassic border . In relation to the number of species, around 95 percent of all marine life forms and around 75 percent of land fauna died out in this period, including - a novelty in the history of the earth - many insect species. The vegetation was so badly decimated by fires, periods of heat and drought, air pollution and soil acidification that the atmospheric oxygen content fell from around 30 percent at the beginning of the Permian to 10 to 15 percent towards the end of the period and then for millions of years (over the entire Triassic and up to the Jura) persisted at a low level. The trigger and main cause of the collapse of the ecosystems is considered to be the large-scale flood basalt emissions of the Siberian trap , which covered an area of 7 million square kilometers with igneous rocks during its activity cycles for several hundred thousand years. Recent studies assume that extensive amounts of fly ash from coal fires and vegetation residues reached the atmosphere and were deposited over a wide area. In addition, gases (carbon dioxide, sulfur dioxide, methane, chloromethane and bromomethane ) that arose from the contact metamorphosis along the extensive underground pathways of the magma - especially along corridors ( sills ) parallel to layers in the Tunguska sedimentary basin - could additionally intensify the outgassing of the flood basalts penetrating the surface to have.

According to recent findings, the mass extinction at that time can be divided into several sections. During phase 1, the global temperature rose by 5 ° C within a geologically very short period of time due to the carbon dioxide emitted by the Siberian Trapp. At the same time, the near-surface water layers of the oceans warmed up by at least 8 ° C by the end of the event, which marked the transition to phase 2: With the formation of oxygen-free (anoxic) sea zones, the rapid drop in the pH value and the release of Methane hydrate began mass extinction in the oceans. Due to the methane escaping into the atmosphere, the global temperature increased by a further 5 ° C in phase 3, and the greenhouse gas concentration reached a CO 2 equivalent value of at least 3,000 ppm. In addition, a short-term galloping greenhouse effect is postulated (English runaway greenhouse effect ) on the basis of a carbon dioxide level of over 7,000 ppm. Another cause of the destabilization of the ecosystem was probably the mass reproduction of marine protozoa in oxygen-free environments, which emitted their metabolic products in the form of methane, halogenated hydrocarbons and large amounts of hydrogen sulfide (H 2 S) into the atmosphere. In addition, it is assumed that the pollutant load in the air also depleted the ozone layer to a considerable extent, with the result of increased UV-B radiation , which may have contributed to pollen malformations and a large-scale decline in vegetation.
Until recently, the duration of the Permian Triassic Crisis was estimated at more than 200,000 years. According to an analysis from 2014, this time window was reduced to two core areas, each spanning 60,000 years (± 48,000 years). In contrast, a study published in 2018 postulates a single event with a maximum duration of around 30,000 years, possibly limited to a few millennia. The global collapse of ecosystems could be dated to the lowest Triassic 251.94 million years ago with the help of newly developed precision measurements. The biological, geophysical and climatic consequences of the mass extinction extended in part to the Middle Triassic . While the ammonites , conodonts and foraminifera recovered within 1 to 3 million years, coral reefs took 8 to 10 million years to fully regenerate. It took even longer to create new forest habitats, which only spread over larger areas after around 15 million years. The gradual renewal of the biotopes damaged by extreme warming, major fires, acid rain and oxygen shortage ("recovery phase") was interrupted several times in the Triassic with a focus on the chronostratigraphic lower levels Smithium and Spathium by further extinction events.
Mesozoic Era (Mesozoic Era)
Triassic-Jura border (approx. 201 million years ago)
Towards the end of the Triassic , a major geological event was heralded with the beginning of the disintegration of the supercontinent Pangea, which had existed since the late Carboniferous . Along the plate edges of today's North America and Europe, extensive rift fractures (rift systems) with the first marine ingresses arose . This development, towards the gradual opening of the later Central Atlantic, resulted in the -Jurassic boundary triad the emergence of 11 million km² Central Atlantic magmatic province (English Central Atlantic Magmatic Province , abbreviated CAMP ), including their Magmaausflüsse of the richest of the known geological history . The main phase of flood basalt volcanism began 201.5 million years ago, lasted approximately 600,000 years, and probably had four brief bursts of greatly increased activity. This plate tectonic process had serious consequences for the atmosphere, climate and biosphere and is generally considered to be the primary cause of mass extinction with a species loss of approximately 70 to 75 percent. The conodonts were completely wiped out in the seas , and on land the archosauria, which are not counted among the dinosaurs , were largely decimated . Other reptiles from the Diapsida group , many amphibians and some precursors of mammals were also affected to a considerable extent .
Several current studies have come to the result that the focus of the mass extinction should be set around 100,000 years before the eruptive flood basalt phase of the CAMP event. According to these analyzes, activity in the Central Atlantic Magmatic Province began at an intrusive stage . In the course of this process, large amounts of magma flowed into evaporite and carbonate deposits (with additional proportions of hydrocarbons ) and over periods of several thousand or ten thousand years caused the outgassing of carbon dioxide in the five-digit gigatons range through contact metamorphosis .
The factors involved in the mass extinction were thus essentially identical to those at the Permian-Triassic boundary: a strong and rapid increase in the atmospheric carbon dioxide concentration and, associated with it, rapid warming, an acidification caused by the input of CO 2 and sulfur dioxide of the ocean, a bio-calcification crisis at the expense of marine organisms that form calcareous shells and possibly the release of bacterially generated methane and hydrogen sulfide . In the upper, euphotic zone of the Tethys Sea - a large marginal sea of the supercontinent Pangea and biodiversity hotspots - the high hydrogen sulfide and low oxygen concentrations may have contributed to the fact that marine ecosystems there only recovered very slowly.
Cretaceous-Paleogene boundary (approx. 66 million years ago)
The Cretaceous-Paleogene boundary (formerly Cretaceous-Tertiary boundary ) also marks the change from the Middle Ages to the Modern Era . At this geological seam 70 of all animal extinctions to 75 percent, including with the exception of birds and the dinosaurs . The cause in the literature two event areas will be discussed: on the one hand the impact ( impact ) of an asteroid near the peninsula Yucatan in Mexico today, which of the 180 km through measured Chicxulub crater was created, and on the other the alternative flood basalt scenario of the Deccan Traps in western India. Some studies postulated at the beginning of the 2000s that the Chicxulub crater formed 300,000 years before the actual Cretaceous-Paleogene boundary . In view of the current data, this assumption is now classified as unlikely. The application of dating methods with very low tolerance ranges led to the result that the impact event and the Cretaceous-Paleogene boundary layer coincide precisely in time. The impact winter following the impact is now considered factually secured. Thus, according to the current state of knowledge, the Chicxulub impact is the primary cause of the mass extinction at that time.

The most common scenario in science assumes that 66.040 million years ago (± 0.032 million years) an approximately 14 km large asteroid detonated at a speed of 20 km / s in a tropical shallow sea and evaporated within a second. Due to the force of the explosion, several thousand cubic kilometers of carbonate and evaporite rock were hurled as glowing ejecta into the stratosphere and sometimes beyond. In addition to the immediate consequences of the impact, such as megatsunamis , a supersonic pressure wave and earthquakes with a magnitude of 11 or 12, forest and wildfires occurred around the world, with brief but severe heating of the atmosphere. Within a few days, a large amount of soot and dust particles spread throughout the atmosphere, absorbing sunlight for months, causing a global drop in temperature and bringing photosynthesis to a standstill in most plants. An additional cooling factor was possibly an atmospheric layer of sulfuric acid - aerosols , which, according to a recent study, could have caused a temperature drop of 26 ° C and contributed significantly to the global average temperature falling below freezing point for a few years , with dramatic consequences for the whole Biosphere. The oceanic and mainland areas were equally affected by this crisis. 75 percent of the species fell victim to mass extinction within a probably very short, but not exactly determinable period of time, including not only the dinosaurs, but also the ammonites , the large marine reptiles such as plesio or mosasaurs , almost all calciferous foraminifera and various plankton groups . Of the Cretaceous bird species only a very small proportion survived the turning point of mass extinction.
A hypothesis presented by several well-known geoscientists in April 2015 assumes that due to the impact energy of 3 × 10 23 joules (according to another calculation 1 × 10 24 joules) and the tectonic shock waves triggered by this, the long "smoldering" Deccan Trapp recorded a significant increase in its activity. According to this hypothesis, the short-term discharge of 70 percent of all Dekkan-Trapp flood basalts is due to the Chicxulub impact. The previously neglected possibility of a causal connection between asteroid impact and flood basalt volcanism is currently being discussed on a broad basis in the geosciences . In science, however, there is disagreement as to whether the main activity of this Magmatic Greater Province took place immediately at or shortly after the Cretaceous-Paleogene border or already before.
Cenozoic (Earth Modern Age)
Eocene-Oligocene boundary (33.9 million years ago)
As part of the Grande Coupure ("Great Gorge") , a major species extinction occurred at the Eocene - Oligocene transition 33.9 to 33.7 million years ago, which was linked to a marked drop in temperature on land and in the oceans (English Eocene- Oligocene extinction event ). About 60 percent of the Eocene mammal genera in Europe were affected by the rapid onset of climate change and its consequences, including a large part of the Palaeotheria (early horse relatives ), primates , Creodonta and other animal groups. While subtropical vegetation still existed in Europe during the Eocene, extensive steppe areas have now emerged due to the cooler and drier climate. On a global scale, the temperature of the oceans fell by 4 to 5 ° C and the sea level fell by about 30 meters.
The climatic changes at the Eocene-Oligocene border can at least partially be explained by plate tectonic processes . Until the later Eocene, Antarctica and South America were connected by a land bridge before the Drake Strait gradually opened. This created in the Southern Ocean the strongest ocean current of the earth, the Antarctic Circumpolar Current , the Antarctica circled from now on in a clockwise direction, the continent from the supply warmer sea water section and the basis for the formation of the Antarctic ice sheet created. Thus, Antarctica was not only geographically isolated, but also thermally, and the inland glaciation that began on the continent 33.7 million years ago was synonymous with the beginning of the Cenozoic Ice Age . In the north polar region, too, a significant cooling was observed. Finds of dropstones show the temporary existence of Greenland continental ice 38 to 30 million years ago. In addition, it is assumed that the Arctic Ocean , which was isolated for a long time, found a connection to the global ocean circulation after a transition phase as a brackish sea in the early Oligocene (≈ 32 mya) with the influx of salty North Atlantic water .
What is striking in this context is the steep drop in the atmospheric CO 2 concentration. While this was still in a range of 700 to 1,000 ppm towards the end of the Eocene, this level dropped abruptly by around 40 percent 33.7 million years ago (and was possibly even lower for a very short geological period). An additional cooling factor, for example in the form of a large meteorite impact, is therefore discussed on various occasions. The most suitable candidate would be the Popigai impact in northern Siberia, with an “official” age of 35.7 million years. According to a recent re-dating, however, the impact event lies directly on the Eocene-Oligocene boundary and, if the information is confirmed, could have been causally linked to the mass extinction at that time.
Pliocene-Pleistocene transition (3.8 to 2.4 million years ago)
The global cooling that intensified at the end of the Pliocene changed into the Quaternary Ice Age with the beginning of the Pleistocene around 2.6 million years ago (as the most recent period of the Cenozoic Ice Age ). Associated with this was a relatively rapid onset of glaciation of large parts of the northern hemisphere and a gradual lowering of the sea level by more than 100 meters. This led to a reduction or desiccation of shallow shelf seas by around 30 percent and thus to a loss of oceanic habitats. The marine megafauna at home near the coast , at its tip the 15 to 20 meter large basking shark megalodon , were particularly affected by this , while marine mammals and sea turtles recorded a loss of species of around 50 percent. Worldwide, the genetic diversity of megafauna decreased by 15 percent during the transition period from the Pliocene to the Pleistocene.
Young Pleistocene (about 50,000 to 12,000 years ago)
Towards the end of the Pleistocene and partially in the early Holocene , a large part of the megafauna of America, Eurasia and Australia was wiped out in the course of the Quaternary extinction . Although only relatively few animal species were affected, the event has a certain significance in the public reception, as it included very large and "popular" animal species such as the mammoth , the woolly rhinoceros and the saber-toothed cat . The reasons for the delayed extinction on different continents are largely unexplained and the subject of scientific controversy. It is often assumed that humans have exterminated various large mammal species through intensive hunting ( overkill hypothesis ) in the course of their immigration to new habitats . On the other hand, the drastic climate change at the end of the last icing phase could have been a decisive impetus for extinction. Newer publications combine climatic and anthropogenic factors into an explanatory model, whereby the human influence is ascribed a clear preponderance.
In some regions, the Pleistocene and today's mass extinctions merge with one another without a clear time limit. Some species, such as the woolly mammoth and the giant deer , disappeared at different times in the course of the Late Pleistocene and Holocene, depending on the region. While the woolly mammoths of the Eurasian and North American mainland no longer lived to see the end of the Pleistocene, some populations on remote islands survived into the Holocene. As the last representative of the Ice Age megafauna, a dwarf form of the mammoth on the 7608 km² Wrangel Island in the Arctic Ocean only died out around 3,700 years ago after humans first reached the island.
In 2007 a startling study postulated that an asteroid or a comet fragment collided with the earth about 12,800 years ago. The Clovis comet theory assumes that the impact (or the detonation of the cosmic body in the atmosphere) had serious consequences, especially in the North Atlantic region. According to this hypothesis, the impact was not only responsible for the disappearance of the Pleistocene megafauna, but also destroyed the early Clovis culture on the American continent. In addition, as a result of the impact, considerable amounts of ash and dust are said to have spread into the atmosphere, which significantly dampened the solar radiation and contributed significantly to the sudden cold snap of the Younger Dryas . Although the hypothesis was an often discussed and widely received topic in the specialist literature, it was largely rejected by scientists until recently due to a lack of convincing evidence. However, several recent studies have listed a number of indications in favor of an impact.
More ecological and biological crises
The following sections describe those events in the Phanerozoic that clearly stand out from the natural background extinction , but do not fall under the term mass extinction : either because only a relatively small number of species or genera were affected or because the extent of environmental changes due to the further development of analytical methods is only in the has been more precisely determined in recent years and is still being discussed at the moment.
Middle Silurian and Silurian-Devonian borders

Until the beginning of the 1990s, the Silurian was regarded as a low-event epoch with a stable warm climate. In the meantime, a number of biological crises and mass extinctions have also been identified during this period, with a focus on the Wenlock series (433.4 to 427.4 mya) and near the Silurian-Devonian border (419.2 mya). During the Wenlock, in the last phase of the Caledonian mountain formation, the continental plates of Laurentia , Baltica and Avalonia finally merged to form the greater continent Laurussia . This resulted in intense volcanism, which caused chemical and climatic anomalies in the atmosphere and oceans and had a lasting effect on the short and long-term carbon cycle. The marine life forms of the conodonts and various plankton groups such as the graptolites were particularly affected . In the latter, the extinction rate rose to 95 percent before biodiversity increased again over longer periods of time. Towards the end of the Silurian, a clear cooling of the oceans became noticeable, coupled with a reshaping of the deep water currents and a change in the organic carbon cycle. The resulting disturbance of the ecological balance including several waves of extinction is documented in detail in the fossil tradition of the cephalopods ( cephalopods ).
Carbon-Permian boundary (298.9 million years ago)
The over millions of years hardly changed position of the United continent Gondwana within the Antarctic contributed by the action of the ice-albedo feedback essential to the creation of Permokarbonen glacial age at which a duration of approximately 80 million years ago by the Lower Carboniferous up in the Middle Permian enough. An additional cooling factor was the increasing spread of deep-rooted and soil-splitting plants during the Carboniferous. The combination of increased soil erosion with extensive coalification processes removed large amounts of carbon from the atmosphere. In the course of this development, the global temperature gradually sank to an ice age level, and the atmospheric CO 2 concentration fell towards the end of the epoch to the lowest level in the Phanerozoic , with a fluctuation range of 150 to 700 coupled with the various cold and warm phases ppm. According to a 2017 study, carbon dioxide levels decreased to the minimum of likely 100 ppm in the early Permian. If this assumption is confirmed, the earth's climate system would have moved into the immediate vicinity of the tipping point around 298 million years ago that would have brought the planet into the climatic state of global glaciation, comparable to the snowball earth events in the Neoproterozoic .
In the late Carboniferous, the rainforests located near the equator collapsed (in the specialist literature Carboniferous Rainforest Collapse ) and with it the first mass extinction of plants. The tropical forests were decimated to a few islands of vegetation within a geologically very short period of time, and the majority of the wetlands and swamps also disappeared. Various arthropods , most of the amphibians and early reptiles with a semi-aquatic way of life were particularly affected by the loss of these biotopes . During the transition phase from the Carboniferous to the Permian, new forest biotopes emerged which were adapted to a cooler and dry climate with seasonal temperature fluctuations. An example of this change is the cold-resistant and deciduous Glossopteris flora in the southern part of Gondwana, which developed into the predominant plant type there.
Jurassic-Cretaceous boundary (145.0 million years ago)
Just like the Silurian, the Jura has long been described in geoscientific treatises as a comparatively quiet epoch. This assessment has changed fundamentally in the last few decades. Accordingly, the middle section of the Mesozoic Era was a time of extensive tectonic processes, strong climatic and sea level fluctuations and an atmospheric oxygen concentration that was well below the current level. In addition, the cooling trend beginning towards the end of the Jurassic and extending into the early Lower Cretaceous is an indicator of a possible mass extinction. However, experts are divided on this issue. While on the one hand a significant loss of species is postulated, especially in the case of marine teleosaurids and ichthyosaurs , other studies contradict this assumption and point to significant gaps in the fossil record at the time in question. Overall, the Jurassic-Cretaceous border seems to be the most inconspicuous of all the epoch transitions of the Phanerozoic from a paleobiological point of view.
Cenomanian-Turonian boundary (93.9 million years ago)
The Cretaceous Period , which spanned a period of 79 million years, exhibited almost continuously tropical to subtropical conditions up to higher latitudes. After a relatively cool beginning, one of the strongest warming phases in the Phanerozoic occurred in the optimum climate of the Middle and Upper Cretaceous , before the temperatures gradually fell again towards the end of the Cretaceous, in the late Maastrichtian due to the Dekkan-Trapp volcanism with abrupt climate changes and presumably seasonal sea ice cover as well the formation of continental ice in higher Antarctic regions. This long-term temperature trend was interrupted several times by pronounced climatic fluctuations, which were associated with varying CO 2 concentrations, extensive tectonic activities and strong volcanism. A special feature of the Cretaceous is the accumulation of oceanic anoxic events , with the one at the Cenomanium - Turonium border ( OAE-2 for short , also called Bonarelli event ) reaching global proportions and probably the most striking disruption of the carbon cycle in the last 100 million years. with significant climatic and biological effects. In the older literature, the event is assigned a duration of 300,000 years, more recent studies estimate around 700,000 years. During the anoxic environmental crisis, combined with a cooling of around 4 ° C, various types of plankton and reef builders as well as the class of cephalopods (including ammonites and belemnites ) were severely decimated, and with the genus Platypterygius , the last representatives of the ichthyosaurs died out. The staggered but ultimately abrupt disappearance of the ichthyosaurs is the expression of a series of biotic events during the OAE-2, which subsequently led to the spread of new species in modified marine ecosystems.
Paleocene / Eocene temperature maximum (55.8 million years ago)
The Paleocene / Eocene Temperature Maximum (PETM) was a very strong warming phase lasting 170,000 to 200,000 years. The global temperature rise took place on the basis of an already existing warm climate and ran parallel to a significant increase in the concentration of greenhouse gases in the atmosphere and the world's oceans. During the PETM, the global temperature rose from around 18 to 24 ° C within a few millennia. Due to the extensive input of carbon dioxide, this caused an acidification of the oceans down to the deeper layers and the creation of anoxic milieus. While on the mainland the expansion of the tropical climatic zone led to extensive migration of flora and fauna and in some species of mammals to short stature due to lack of food in connection with phenotypic reactions , in the oceans there was a mass extinction of the benthic foraminifera with a species loss between 30 and 50 Percent. The significant decrease in the pH value played a decisive role in the destabilization of the marine biotopes . Affected by this, but only partially threatened with extinction, were organisms settled in the deep sea (sea urchins, mussels, snails) as well as almost all plankton groups . A tendency to "dwarf" also recorded many marine species, including the ostracods (shellfish). This development most likely resulted from the warming and acidification of the deep sea regions and a related disruption of the remineralization processes of organic carbon.
The current mass extinction

The wave of extinction , which was mainly based on human influences, began at the transition from the Pleistocene to the Holocene and continues, with increasing amplification, to the present. The International Union for Conservation of Nature (IUCN) assumes that the currently registered loss of species exceeds the rate of normal background extinction by 1,000 to 10,000 times. Further investigation also suggests that the sixth mass extinction has already begun. Since humans settled down in the Neolithic Age, the animal world, especially the megafauna, has been greatly suppressed. When humans settled on other islands from around 800 AD, they exterminated the megafauna there, which had a low reproductive rate and had no escape reflexes, through excessive hunting (Madagascar, the moas in New Zealand) or made the resident bird life disappear ( Hawaii, Polynesia). Extinction accelerated with the Age of Discovery from around 1500, when Europeans colonized other continents, exterminated alleged pests and brought in neozoa (rats, foxes, pigs) to which the native fauna was often defenseless.
The 2014 annual report of the World Wide Fund For Nature (WWF) speaks of a sometimes dramatic deterioration in the situation of many species such as rhinos (of one subspecies, the northern white rhinoceros , there are only a few specimens left, according to WWF), elephants ( poachers in Africa kills more elephants than offspring are born), lions (in West Africa they are on the verge of extinction, in India there are only remnants) or walruses (they are victims of climate change, their resting places on ice floes disappear as the arctic pack ice recedes ) . Many other animals are losing their habitat according to the WWF: apes like the bonobos lose their last reserves, for example, caused by a proposed in a national park in Congo oil production. The primate 94 percent currently found on the Red List in one of the three highest risk category (as of 2014). According to WWF, biodiversity has suffered greatly since the 1970s; the number of mammals, birds , reptiles and fish has since halved on average, the world is losing 380 animal and plant species every day.
"Humans are causing the greatest global extinction of species since the disappearance of the dinosaurs."
The Living Planet Report published by the WWF at the end of October 2016 in cooperation with the London Zoological Society (ZSL) and the Global Footprint Network (GFN) reports a decline in populations in over 14,000 animal populations examined worldwide nearly 60 percent during the past 40 years; the stocks of animals in rivers and lakes have declined by an average of 81 percent worldwide.
"If we do not expand organic farming significantly, we cannot say for sure whether there will be anything left of the current bird population in ten or 20 years."
Currently (May 2019) are, according to the Weltbiodiversitätsrat (IPBES species conservation conference in Paris) threatened up to a million species with extinction, 500,000 of which are considered "dead species walking" (in a sense living dead called). The cycads are particularly threatened with plants, some are already extinct. The (risk) factors listed below contribute in their totality and on time scales of different lengths to the possibility of anthropogenic mass extinction:
- Intensive land use, especially deforestation or clearing of forests as well as the transformation of natural ecosystems into agriculturally usable areas ( monocultures ) with the application of pesticides with the risk of e.g. B. numerous species of insects
- Commercial hunting of protected animal species
- Global warming on a regional and global level through anthropogenic emissions, with consequences such as shifting climate zones, increasing extreme weather, warming and acidification of the oceans
- Endangerment of marine biodiversity through overfishing , by-catches and violations of species protection
- Pollutant inputs into marine systems: wastewater from industry and agriculture, dumping of heavy fuel oil , various oil spill events, halogenated and chlorinated hydrocarbons , heavy metals such as lead , cadmium or mercury , nuclear waste or radioactively contaminated wastewater, solid waste (mainly in the form of plastics , including significant amounts of nanoparticles )
- Immigration of neophytes , with possible displacement of autochthonous (native) species in flora and fauna
- Viral infections
- The road , the effect of light pollution on living organisms and the Verunruhigung the landscape as by its use as recreation areas are also important factors.
Mass extinction and evolution
The emergence of new species
A mass extinction was usually followed by an accelerated phase of adaptive radiation by the surviving species, which, as they reproduced, colonized habitats that were previously occupied by competing life forms. The speed of this spread depended to a large extent on the geophysical framework conditions and thus on the usability of the available biotopes. While, for example, mammals benefited from the relatively rapid regeneration of natural systems after the upheaval at the Cretaceous-Paleogene frontier , and in the Paleocene, an initial increase in biodiversity in the period 0.4 to 1.0 million years after the extinction event and thus recorded the formation of new species, normalization was slow following the mass extinction at the Permian-Triassic transition. Due to the ongoing environmental crisis with numerous consequences, it sometimes took 10 to 15 million years before a stable ecological equilibrium was established. During this transitional period, some more robust species sometimes occupied many orphaned habitats (“ catastrophe taxa ”) with massive reproduction before they were pushed back by the competitive pressure of immigrating populations. A similarly delayed regeneration could also have occurred in the (albeit controversial) fossil gap of the early Carboniferous ("Romers's gap") , which was possibly a late consequence of the previous Hangenberg event .
Extinction and survival patterns using the example of the Chicxulub impact
The late Maastrichtian , the last stage of the Cretaceous , showed, in addition to a clear cooling tendency, several short-term and strongly pronounced climatic fluctuations, which probably go back to the long-lasting flood basalt volcanism of the Dekkan-Trapp in today's West India. As a result, scientists have held the view for decades - seemingly supported by the fossil record - that many species of the Mesozoic fauna were "on the decline" long before their ultimate disappearance. More recent studies, on the other hand, paint a differentiated picture of the events of that time. Overall, there is no clear evidence that dinosaurs and other animal groups were affected by long-term decline even before the drastic cut at the Cretaceous-Paleogene border . This finding also applies to the pterosauria ( pterosaurs ), the biodiversity of which apparently remained stable during the Upper Cretaceous and which could possibly record an increase in diversity until their abrupt extinction. There is thus much to suggest that the biological crisis at the end of the Mesozoic era was caused solely by the Chicxulub impact.
The impact event 66 million years ago caused a number of serious environmental changes that occurred in staggered chronological order and triggered a worldwide catastrophe that killed all non-avian dinosaurs and a large part of the other fauna. Already in the first hour after the impact, extremely strong earthquakes and a supersonic pressure and heat wave in connection with a shower-like tektite rain led to a mass extinction of the land and water fauna in a wider area around the epicenter . Events immediately following this were a megatsunami and the emergence of global wildfires due to the widely distributed ejection of glowing rock debris (ejecta) . In the next stage, a cloud of dust and soot enveloping the earth formed within a few days, which absorbed the sunlight, largely suppressed plant photosynthesis and marked the beginning of a rapidly onset of impact winter. This resulted in a strong decimation of the land vegetation with a corresponding effect on large herbivores.
The complete extinction of the relatively species-poor dinosaur population was probably due to a combination of various factors. Due to the size of the individual individuals, there was no way to escape the primary consequences of the asteroid impact and - unlike mammals, for example - to flee into natural protected areas such as burrows. As predominantly endothermic (warm-blooded) animals, dinosaurs needed a sufficient supply of food to maintain their body temperature and vital functions, which, however, decreased significantly in the course of the impact catastrophe. Essentially, the group of dinosaurs was divided into herbivores and carnivores , whether or not they included omnivores (omnivores), such as the ornithomimosauria , is currently not known. Of the mammals, no purely herbivorous or carnivorous forms appear to have survived the event. In contrast, omnivores and insectivores were able to hold their own to some extent, as they used insects, worms and snails as a source of food. The lower end of the food chain was formed by so-called detritus , an as yet non- humified organic substance in or on the ground, consisting of dead plant tissue, pollen, carrion, microorganisms and other organic material. It is assumed that the detritus deposits on the Cretaceous-Paleogene border did not suffer any significant losses and thus ensured the existence of many smaller living beings.
The flora and fauna in present-day North America were among the most severely damaged. According to a statistical study, almost 60 percent of all plant species died out in this region. The reduction in flora was less noticeable in the southern hemisphere. Nevertheless, here, as everywhere, there was a spread of fungi, mosses and lichens and, after some time, a growth spike in ferns. The fact that the vegetation crisis was only of a temporary nature is probably due to the polyploidy that occurs in many higher plants . Polyploidy describes the genetic characteristic when an organism has several sets of chromosomes in a cell. This property often leads to increased vitality and better adaptation to deteriorating climatic conditions in plants and could have contributed to survival in the drastically changed environment of the post-impact era.

In addition to the Cretaceous birds, of which only a few species found shelter in the paleogene, lizards and snakes also recorded considerable losses. Since no land animal with a body weight of more than 15 to 20 kg survived the period of crisis, short stature was generally an advantage. Crocodiles were a certain exception in this regard. Although their largest representatives in the form of marine reptiles also died out, as ectothermic (cold-blooded) creatures they were able to forego food for a very long time, and because of their semi-aquatic way of life in rivers and lakes, they could avoid the worst disturbances and thus limit species loss to around 50 percent. This leads to the assumption that freshwater biotopes are among the relatively safe areas of life among the ecological niches.
In contrast, the mass extinction in the oceans began with a slight time lag, but then with serious consequences. Rapidly occurring geochemical changes, caused by the massive input of pollutants such as nitrogen oxides and sulfur dioxide or the resulting sulfuric acid, were responsible for this . These processes were mainly at the expense of calcareous organisms, whose extinction rate for ammonites was 100 percent and for foraminifera and various plankton species exceeded 90 percent. This indicates strong acidification of the oceanic areas over tens of thousands of years, and it presumably took over a million years before the sustainably disturbed oceanic equilibrium was fully restored. What is certain is that with the extensive reduction in nannoplankton, many of the life forms that are dependent on it have lost their nutritional basis, which has had a chain reaction on the entire marine ecosystem and ultimately also on the megafauna indigenous to it. Thus, the mass extinction in the seas reached similar dimensions as that on the mainland.
The impact winter, which lasted for several decades, is one of the main causes of the mass extinction at that time. There are different assumptions in science about the extent of the cold snap. While on the one hand a temperature drop of 26 ° C with global permafrost caused by an atmospheric layer of sulfuric acid aerosols is postulated, according to another study a comparatively moderate and temporary cooling took place. On the other hand, there is agreement that the recovery of the southern hemisphere biotopes evidently proceeded significantly faster than that of the corresponding regions in the northern hemisphere. After the end of the cold phase, the earth entered the stage of global warming, partly due to the billions of tons of carbon dioxide that the Chicxulub impact had released as a result of the evaporation of oceanic soils, to a greater extent due to the increased CO 2 emissions of the Deccan -Trapps, possibly initiated by the tectonic tremors of the asteroid impact. The extent to which the rapid onset of the greenhouse climate, which lasted at least 50,000 years, played a role in the mass extinction has not yet been adequately clarified in detail.
The authors of a study published at the end of 2017 came to the conclusion that the asteroid hit rock layers that contained high concentrations of hydrocarbons and sulfates . Due to the extensive heating and evaporation of the oceanic sediments in this region, extensive amounts of soot and sulfur aerosols were distributed in the stratosphere , which considerably increased the climatic effects of the following impact winter in terms of its duration and extent. If the asteroid had hit an area with lower levels of hydrocarbons (around 87 percent of the earth's surface), the biological crisis would probably have been far more mild, with a significantly higher survival rate for the Mesozoic fauna. A study published in 2020 postulated on the basis of computer simulations that the likely angle of impact of the asteroid, which is 45 to 60 degrees, produced the most devastating effects of all impact scenarios.
See also
literature
English language works
- Ashraf Elewa (Ed.): Mass Extinction. Springer, Berlin a. a. 2008, ISBN 978-3-540-75915-7 .
- George R. McGhee Jr: When the Invasion of Land Failed. The Legacy of the Devonian Extinctions. Columbia University Press, New York 2013, ISBN 978-0-231-16057-5 (illustration of various biological crises in connection with the Devonian mass extinctions).
- George R. McGhee Jr .: Carboniferous Giants and Mass Extinction. The Late Paleozoic Ice Age World. Columbia University Press, New York 2018, ISBN 978-0-231-18097-9 (Description of flora and fauna during the Permocarbon Ice Age with an emphasis on the Carboniferous).
German language works
- Matthias Glaubrecht : The end of evolution: man and the destruction of species . C. Bertelsmann, Munich 2019. ISBN 978-3-570-10241-1 .
- Peter Ward , Joe Kirschvink : A New Story of Life. How catastrophes determined the course of evolution. Deutsche Verlags Anstalt, Munich 2016. ISBN 978-3-421-04661-1 .
- Norman MacLeod: Species Die - Turning Points in Evolution. Theiss Verlag - Wissenschaftliche Buchgesellschaft, Darmstadt 2016, ISBN 978-3-8062-3284-4 (popular scientific presentation by a paleontologist on the entire topic).
- Jens Boenigk, Sabina Wodniok: Biodiversity and Earth History . Springer Verlag, Berlin - Heidelberg 2014 (Springer Spectrum), DOIː 10.1007 / 978-3-642-55389-9 , ISBN 978-3-642-55388-2 (textbook on the emergence of diversity in the geological context).
- Elizabeth Kolbert : The sixth dying. How man writes natural history. Translated from the English by Ulrike Bischoff. Suhrkamp, Berlin 2015, ISBN 3-515-08518-1 .
- Ted Nield: Super Continent . The Secret Life of Our Planet: An Adventurous Journey Through Earth's History. Verlag Antje Kunstmann, Munich 2008, ISBN 978-3-88897-526-4 .
- József Pálfy: Disasters in the history of the earth. Global extinction? Schweizerbart, Stuttgart 2005, ISBN 3-510-65211-8 .
- David M. Raup : The Nemesis Affair. A Story of the Death of Dinosaurs and the Ways of Science , WW Norton 1986, German edition: The black star. How the dinosaurs died - the dispute over the nemesis hypothesis. Rowohlt, Reinbek 1990, ISBN 3-498-05720-0 (on the nemesis hypothesis of a periodic extraterrestrial cause, which was popular in the 1980s).
Web links
- astris.de: The history of the earth - mass extinction
- scinexx.de: mass extinction
- oekosystem-erde.de: mass extinction
- Peter D. Ward: Death from Below. In: Spektrum der Wissenschaft , 3/2007, pp. 26–33.
Individual evidence
- ^ A b Richard J. Twitchett: The palaeoclimatology, palaeoecology and palaeoenvironmental analysis of mass extinction events . (PDF) In: Palaeogeography, Palaeoclimatology, Palaeoecology . 232, No. 2-4, March 2006, pp. 190-213. doi : 10.1016 / j.palaeo.2005.05.019 .
- ↑ The types of loss can not only by an increased extinction, but also a low Artbildungsrate be contributed, see Bond and Grasby (2017), page 8, and Anthony D. Barnosky , Nicholas Matzke, Susumu Tomiya, Guinevere OU Wogan, Brian Swartz, Tiago B. Quental, Charles Marshall, Jenny L. McGuire, Emily L. Lindsey, Kaitlin C. Maguire, Ben Mersey, Elizabeth A. Ferrer: Has the Earth's sixth mass extinction already arrived ?. In: Nature. 471, No. 7336, March 2011, pp. 51-52.
- ↑ Anthony D. Barnosky, Nicholas Matzke, Susumu Tomiya, Guinevere OU Wogan, Brian Swartz, Tiago B. Quental, Charles Marshall, Jenny L. McGuire, Emily L. Lindsey, Kaitlin C. Maguire, Ben Mersey, Elizabeth A. Ferrer: Has the Earth's sixth mass extinction already arrived? . In: Nature . 471, No. 7336, March 2011, pp. 51-57. doi : 10.1038 / nature09678 .
- ^ A b Richard K. Bambach: Phanerozoic biodiversity mass extinctions . In: Annual Review of Earth and Planetary Sciences . 34, May 2006, pp. 127-155. doi : 10.1146 / annurev.earth.33.092203.122654 .
- ^ LW Alvarez, W. Alvarez, F. Asaro, HW Michel: Extraterrestrial Cause for the Cretaceous-Tertiary Extinction . (PDF) In: Science . 208, No. 4448, June 1980, pp. 1095-1108.
- ↑ David M. Raup: The black star. How the dinosaurs died - the dispute over the nemesis hypothesis. Rowohlt, Reinbek 1990, ISBN 3-498-05720-0 , p. 73.
- ↑ a b c d e f g David PG Bond, Stephen E. Grasby: On the causes of mass extinctions . (PDF) In: Palaeogeography, Palaeoclimatology, Palaeoecology . 478, No. 15, July 2017, pp. 3–29. doi : 10.1016 / j.palaeo.2016.11.005 .
- ↑ Alycia L. Stigall: Speciation collapse and invasive species dynamics during the Late Devonian “Mass Extinction” . In: GSA Today (Geological Society of America) . 22, No. 1, January 2012, pp. 4-9. doi : 10.1130 / G128A.1 .
- ↑ Ulf von Rauchhaupt: The world has already ended five times . In: FAZ.NET
- ^ Paul B. Wignall: The Worst of Times . Princeton University Press, 2016, ISBN 978-0-691-17602-4 .
- ↑ Ben G. Mason, David M. Pyle, Clive Oppenheimer: The size and frequency of the largest explosive eruptions on Earth . (PDF) In: Bulletin of Volcanology . 66, No. 8, December 2004, pp. 735-748. doi : 10.1007 / s00445-004-0355-9 .
- ^ Robert B. Smith, Lawrence W. Braile: Crustal Structure and Evolution of an Explosive Silicic Volcanic System at Yellowstone National Park In Geology of Yellowstone Park Area; 33rd Annual Field Conference Guidebook , 1982, pp. 233-250.
- ^ David PG Bond, Paul B. Wignall: Large igneous provinces and mass extinctions: An update . (PDF) In: The Geological Society of America (GSA) Special Paper . 505, September 2014, pp. 29-55. doi : 10.1130 / 2014.2505 (02) .
- ^ A b Daniel H. Rothman, Gregory P. Fournier, Katherine L. French, Eric J. Alm, Edward A. Boyle, Changqun Cao, Roger E. Summons: Methanogenic burst in the end-Permian carbon cycle . In: PNAS . 111, No. 15, April 2014, pp. 5462-5467. doi : 10.1073 / pnas.1318106111 .
- ↑ a b Mark A. Richards, Walter Alvarez, Stephen Self, Leif Karlstrom, Paul R. Renne, Michael Manga, Courtney J. Sprain, Jan Smit, Loÿc Vanderkluysen, Sally A. Gibson: Triggering of the largest Deccan eruptions by the Chicxulub impact . (PDF) In: Geological Society of America Bulletin . April 2015. doi : 10.1130 / B31167.1 .
- ↑ Jennifer Kasbohm, Blair Schoene: Rapid eruption of the Columbia River flood basalt and correlation with the mid-Miocene climate optimum . (PDF) In: Science Advances . 4, No. 9, September 2018. doi : 10.1126 / sciadv.aat8223 .
- ↑ Marten Scheffer, Victor Brovkin, Peter M. Cox: Positive feedback between global warming and atmospheric CO 2 concentration inferred from past climate change . In: Geophysical Research Letters . 33, No. 10, May 2006, pp. L10702 1-4. doi : 10.1029 / 2005GL025044 .
- ↑ David L. Kidder, Thomas R. Worsley: A human-induced hothouse climate? . (PDF) In: GSA Today (The Geological Society of America) . 22, No. 2, February 2012, pp. 4-11. doi : 10.1130 / G131A.1 .
- ↑ Timothy M. Lenton, Hermann Held, Elmar Kriegler, Jim W. Hall, Wolfgang Lucht, Stefan Rahmstorf, Hans Joachim Schellnhuber: Tipping elements in the Earth's climate system . In: PNAS . 105, No. 6, 2008, pp. 1786-1793. doi : 10.1073 / pnas.0705414105 .
- ↑ Vincent E. Courtillot, Paul R. Renne: Time-correlation of mass-extinctions and flood-basalt-Events . (PDF) In: Comptes Rendus Geoscience . 335, No. 1, January 2003, pp. 113-140. doi : 10.1016 / S1631-0713 (03) 00006-3 .
- ↑ Vincent E. Courtillot, Paul R.Renne: On the ages of flood basalt events Comptes Rendus Geoscience 335, 2003
- ↑ Katja M. Meyer, Lee R. Kump: Oceanic Euxinia in Earth History: Causes and Consequences . (PDF) In: Annual Review of Earth and Planetary Sciences . 36, May 2008, pp. 251-288. doi : 10.1146 / annurev.earth.36.031207.124256 .
- ^ Gregory A. Brennecka, Achim D. Herrmann, Thomas J. Algeo, Ariel D. Anbar: Rapid expansion of oceanic anoxia immediately before the end-Permian mass extinction . In: PNAS . 108, No. 43, October 2011, pp. 17631-17634. doi : 10.1073 / pnas.1106039108 .
- ^ Alan R. Hildebrand, Glen T. Penfield, David A. Kring, Mark Pilkington, Antonio Camargo, Stein B. Jacobsen, William V. Boynton: Chicxulub Crater: A possible Cretaceous / Tertiary boundary impact crater on the Yucatán Peninsula, Mexico . (PDF) In: Geology . 19, No. 9, September 1991, pp. 867-871.
- ↑ Peter Ward, Joe Kirschvink: A New Story of Life. How catastrophes determined the course of evolution. Deutsche Verlags-Anstalt, Munich 2016, ISBN 978-3-421-04661-1 , p. 36.
- ↑ a b Christian Koeberl: Mass Extinctions and Impact Events in Earth History: A Brief Overview. Originally in: Yearbook of the Federal Geological Institute (Austria), Volume 147 / Issue 1 + 2, commemorative publication for the 65th birthday of HR Univ.-Prof. Dr. Hans Peter Schönlaub, Director of the Federal Geological Institute.
- ↑ AY Glikson, AJ Meixner, B. Radke, IT Uysal, E. Saygin, J. Vickers, TP Mernagh: Geophysical anomalies and quartz deformation of the structure Warburton West, central Australia . (PDF) In: Tectonophysics . 643, March 2015, pp. 55-72. doi : 10.1016 / j.tecto.2014.12.010 .
- ↑ a b A. L. Melott, BS Lieberman, CM Laird, LD Martin, MV Medvedev, BC Thomas, JK Cannizzo, N. Gehrels, CH Jackman: Did a gamma-ray burst initiate the late Ordovician mass extinction? . (PDF) In: International Journal of Astrobiology . 3, No. 1, April 2004, pp. 55-61. doi : 10.1017 / S1473550404001910 .
- ↑ KZ Stanek, OY Gnedin, JF Beacom, AP Gould, JA Johnson, JA Kollmeier, M. Modjaz, MH Pinsonneault, R. Pogge, DH Weinberg: Protecting Life in the Milky Way: Metals Keep the GRBs Away . (PDF) In: Acta Astronomica . 56, December 2006, pp. 333-345. arxiv : astro-ph / 0604113 .
- ↑ K. Knie, G. Korschinek, T. Faestermann, EA Dorfi, G. Rugel, A. Wallner: 60 Fe Anomaly in a Deep-Sea Manganese Crust and Implications for a Nearby Supernova Source . (PDF) In: Physical Review Letters . 93, No. 17, October 2004, pp. 171103-1-171103-4. doi : 10.1103 / PhysRevLett.93.171103 .
- ^ A. Wallner, J. Feige, N. Kinoshita, M. Paul, LK Fifield, R. Golser, M. Honda, U. Linnemann, H. Matsuzaki, S. Merchel, G. Rugel, SG Tims, P. Steier , T. Yamagata, SR Winkler: Recent near-Earth supernovae probed by global deposition of interstellar radioactive 60 Fe . In: Nature . 532, No. 7597, April 2016, pp. 69-72. doi : 10.1038 / nature17196 .
- ^ A b David M. Raup, J. John Sepkoski: Periodicity of extinctions in the geologic past . (PDF) In: Proceedings of the National Academy of Sciences (PNAS) . 81, No. 4539, 1984, pp. 801-805.
- ^ Marc Davis, Piet Hut, Richard A. Muller: Extinction of species by periodic comet showers . (PDF) In: Nature . 308, April 1984, pp. 715-717. doi : 10.1038 / 308715a0 .
- ^ Matthias MM Meier, Sanna Holm-Alwmark: A tale of clusters: no resolvable periodicity in the terrestrial impact cratering record . In: Monthly Notices of the Royal Astronomical Society . 467, No. 3, June 2017, pp. 2545-2551. doi : 10.1093 / mnras / stx211 .
- ↑ Heather D. Graven: Impact of fossil fuel emissions on atmospheric radiocarbon and various applications of radiocarbon over this century . In: pnas . 112, No. 31, July 2015, pp. 9542-9545. doi : 10.1073 / pnas.1504467112 .
- ↑ Melanie J. Leng, Jim D. Marshall: Palaeoclimate interpretation of stable isotope data from lake sediment archives . In: Quaternary Science Reviews . tape 23 , no. 7–8 , April 2004, pp. 811-831 , doi : 10.1016 / j.quascirev.2003.06.012 .
- ↑ Jung-Hyun Kim, Stefan Schouten, Ellen C. Hopmans, Barbara Donner, Jaap S. Sinninghe Damsté: Global sediment core-top calibration of the TEX 86 paleothermometer in the ocean . (PDF) In: Geochimica et Cosmochimica Acta . 72, No. 4, February 2008, pp. 1154-1173. doi : 10.1016 / j.g approx.2007.12.010 .
- ↑ K. Panchuk, A. Ridgwell, LR Kump: Sedimentary response to Paleocene-Eocene Thermal Maximum carbon release: A model-data comparison . In: Geology . 36, No. 4, April 2008, pp. 315-318. doi : 10.1130 / G24474A.1 .
- ^ Heinrich D. Holland: The oxygenation of the atmosphere and oceans . (PDF): Philosophical Transactions of the Royal Society B . 361, No. 1470, June 2006, pp. 903-915. doi : 10.1098 / rstb.2006.1838 .
- ↑ Robert E. Kopp, Joseph L. Kirschvink, Isaac A. Hilburn, Cody Z. Nash: The Paleoproterozoic snowball Earth: A climate disaster triggered by the evolution of oxygenic photosynthesis . In: PNAS . 102, No. 32, 2005, pp. 11131-11136. doi : 10.1073 / pnas.0504878102 .
- ^ Alan D. Rooney, Justin V. Strauss, Alan D. Brandon, Francis A. Macdonald: A Cryogenian chronology: Two long-lasting synchronous Neoproterozoic glaciations . (PDF) In: Geology . 43, No. 5, May 2015, pp. 459-462. doi : 10.1130 / G36511.1 .
- ^ Frank A. Corsetti, Stanley M. Awramik, David Pierce: A complex microbiota from snowball Earth times: Microfossils from the Neoproterozoic Kingston Peak Formation, Death Valley, USA . In: PNAS . 100, No. 8, April 2003, pp. 4399-4404. doi : 10.1073 / pnas.0730560100 .
- ↑ Marc Laflamme, Simon AF Darroch, Sarah M. Tweedt, Kevin J. Peterson, Douglas H. Erwin: The end of the Ediacara biota: Extinction, biotic replacement, or Cheshire Cat? . (PDF) In: Gondwana Research . 23, No. 2, March 2013, pp. 558-573. doi : 10.1016 / j.gr.2012.11.004 .
- ^ Richard J. Squire, Ian H. Campbell, Charlotte M. Allen, Christopher JL Wilson: Did the Transgondwanan Supermountain trigger the explosive radiation of animals on Earth? . (PDF) In: Earth and Planetary Science Letters . 250, No. 1-2, October 2006, pp. 116-133. doi : 10.1016 / j.epsl.2006.07 .
- ↑ Lawrence M. Och, Graham A. Shields-Zhou, Simon W. Poulton, Christina Manning, Matthew F. Thirlwall, Da Li, Xi Chen, Hongfei Ling, Tony Osborn, Lorenzo Cremonese: Redox changes in Early Cambrian black shales at Xiaotan section, Yunnan Province, South China . (PDF) In: Precambrian Research . 225, February 2013, pp. 166-189. doi : 10.1016 / j.precamres.2011.10.005 .
- ↑ Tomoko Ishikawa, Yuichiro Ueno, Degan Shu, Yong Li, Jian Han, Junfeng Guo, Naohiro Yoshida, Tsuyoshi Komiya: Irreversible change of the oceanic carbon cycle in the earliest Cambrian: High-resolution organic and inorganic carbon chemostratigraphy in the Three Gorges area , South China . (PDF) In: Precambrian Research . 225, February 2013, pp. 190-208. doi : 10.1016 / j.precamres.2011.10.004 .
- ^ Benjamin C. Gill, Timothy W. Lyons, Seth A. Young, Lee R. Kump, Andrew H. Knoll, Matthew R. Saltzman: Geochemical evidence for widespread euxinia in the Later Cambrian ocean . (PDF) In: Nature . 469, No. 7328, January 2011, pp. 80-83. doi : 10.1038 / nature09700 .
- ↑ F. Jourdan, K. Hodges, B. Sell, U. Schaltegger, MTD Wingate, LZ Evins, U. Söderlund, PW Haines, D. Phillips, T. Blenkinsop: High-precision dating of the Kalkarindji large igneous province, Australia , and synchrony with the Early-Middle Cambrian (Stage 4-5) extinction . (PDF) In: Geology . 42, No. 6, June 2014, pp. 543-546. doi : 10.1130 / G35434.1 .
- ↑ Jennifer L. Morris, Mark N. Puttick, James W. Clark, Dianne Edwards, Paul Kenrick, Silvia Pressel, Charles H. Wellman, Ziheng Yang, Harald Schneider, Philip CJ Donoghue: The timescale of early land plant evolution . In: PNAS . February 2018. doi : 10.1073 / pnas.1719588115 .
- ↑ Timothy M. Lenton, Michael Crouch, Martin Johnson, Nuno Pires, Liam Dolan: First plants cooled the Ordovician . (PDF) In: Nature Geoscience . 5, February 2012, pp. 86-89. doi : 10.1038 / ngeo1390 .
- ↑ Pascale F. Poussart, Andrew J. Weaver, Christopher R. Barne: Late Ordovician glaciation under high atmospheric CO 2 : A coupled model analysis . (PDF) In: Paleoceanography . 14, No. 4, August 1999, pp. 542-558.
- ↑ Thijs RA Vandenbroucke, Poul Emsbo, Axel Munnecke, Nicolas Nuns, Ludovic Duponchel, Kevin Lepot, Melesio Quijada, Florentin Paris, Thomas Servais, Wolfgang Kiessling: Metal-induced malformations in early Palaeozoic plankton are harbingers of mass extinctions . In: Nature Communications . 6, August 2015. doi : 10.1038 / ncomms8966 .
- ↑ John A. Long, Ross R. Large, Michael SY Lee, Michael J. Benton, Leonid V. Danyushevsky, Luis M. Chiappe, Jacqueline A. Halpin, David Cantrill, Bernd Lottermoser: Severe selenium depletion in the Phanerozoic oceans as a factor in three global mass extinction events . (PDF) In: Gondwana Research . 36, August 2016, pp. 209-218. doi : 10.1016 / j.gr.2015.10.001 .
- ^ David M. Raup, J. John Sepkoski: Mass Extinctions in the Marine Fossil Record . (PDF) In: Science . 215, No. 4539, March 1982, pp. 1501-1503.
- ↑ RT Becker, P. Königshof, CE Brett: Devonian climate, sea level and evolutionary events: an introduction . (PDF) In: Geological Society, London, Special Publications . 423, August 2016, pp. 1–10. doi : 10.1144 / SP423.15 .
- ^ "Middle to Upper Devonian biotic crisis", see Thomas J. Algeo, Stephen E. Scheckler: Terrestrial-marine teleconnections in the Devonian: links between the evolution of land plants, weathering processes, and marine anoxic events. In: Philosophical Transactions of the Royal Society of London B (Biological Sciences). 353, No. 1365, 1998, pp. 113–130, doi : 10.1098 / rstb.1998.0195 , PMC 1692181 (free full text)
- ↑ Marina Kloppischː Organic-geochemical comparison of selected rocks of the Frasnium / Famennium border (Oberdevon) in the Bergisches Land and the Eifel (PDF). Reports from Forschungszentrum Jülich, Institute for Chemistry and Dynamics of the Geosphere, 2002.
- ^ David De Vleeschouwer, Micha Rakociński, Grzegorz Racki, David PG Bond, Katarzyna Sobień, Philippe Claeys: The astronomical rhythm of Late-Devonian climate change (Kowala section, Holy Cross Mountains, Poland) . (PDF) In: Earth and Planetary Science Letters . 365, March 2013, pp. 25-37. doi : 10.1016 / j.epsl.2013.01.016 .
- ^ Sarah K. Carmichael, Johnny A. Waters, Cameron J. Batchelor, Drew M. Coleman, Thomas J. Suttner, Erika Kido, LM Moore, Leona Chadimová: Climate instability and tipping points in the Late Devonian: Detection of the Hangenberg Event in an open oceanic island arc in the Central Asian Orogenic Belt . (PDF) In: Gondwana Research . 32, April 2016, pp. 213-231. doi : 10.1016 / j.gr.2015.02.009 .
- ↑ Sandra Isabella Kaiser, Ralf Thomas Becker, Thomas Steuber, Sarah Zhor Aboussalam: Climate-controlled mass extinctions, facies, and sea-level changes around the Devonian – Carboniferous boundary in the eastern Anti-Atlas (SE Morocco) . (PDF) In: Palaeogeography, Palaeoclimatology, Palaeoecology . 310, No. 3-4, October 2011, pp. 340-364. doi : 10.1016 / j.palaeo.2011.07.026 .
- ↑ Grzegorz Racki, Michał Rakociński, Leszek Marynowski, Paul B. Wignall: Mercury enrichments and the Frasnian-Famennian biotic crisis: A volcanic trigger proved? . (PDF) In: Geology . 46, No. 6, June 2018, pp. 543-546. doi : 10.1130 / G40233.1 .
- ↑ J. Ricci, X. Quidelleur, V. Pavlov, S. Orlov, A. Shatsillo, V. Courtillot: New 40 Ar / 39 Ar and K-Ar ages of the Viluy traps (Eastern Siberia): Further evidence for a relationship with the Frasnian-Famennian mass extinction . (PDF) In: Palaeogeography, Palaeoclimatology, Palaeoecology . 386, September 2013, pp. 531-540. doi : 10.1016 / j.palaeo.2013.06.020 .
- ↑ Leszek Marynowski, Michał Zatoń, Michał Rakociński, Paweł Filipiak, Slawomir Kurkiewicz, Tim J. Pearce: Deciphering the upper Famennian Hangenberg Black Shale depositional environments based on multi-proxy record . (PDF) In: Palaeogeography, Palaeoclimatology, Palaeoecology . 346-347, August 2012, pp. 66-86. doi : 10.1016 / j.palaeo.2012.05.020 .
- ^ A b Sandra Isabella Kaiser, Markus Aretz, Ralph Thomas Becker: The global Hangenberg Crisis (Devonian-Carboniferous transition): review of a first-order mass extinction . (PDF) In: Geological Society, London, Special Publications . 423, August 2016, pp. 387-437.
- ^ Paul M. Myrow, Jahandar Ramezani, Anne E. Hanson, Samuel A. Bowring, Grzegorz Racki, Michał Rakociński: High-precision U – Pb age and duration of the latest Devonian (Famennian) Hangenberg event, and its implications . (PDF) In: Terra Nova . 26, No. 3, June 2014, pp. 222–229. doi : 10.1111 / ter.12090 .
- Jump up ↑ Andrew J. Retzler, Leif Tapanila, Julia R. Steenberg, Carrie J. Johnson, Reed A. Myers: Post-impact depositional environments as a proxy for crater morphology, Late Devonian Alamo impact, Nevada . (PDF) In: Geosphere (Geological Society of America) . 11, No. 1, January 2015, pp. 123-143. doi : 10.1130 / GES00964.1 .
- ↑ David PG Bond, Paul B. Wignall, Michael M. Joachimski, Yadong Sun, Ivan Savov, Stephen E. Grasby, Benoit Beauchamp, Dierk PG Blomeier: An abrupt extinction in the Middle Permian (Capitanian) of the Boreal Realm (Spitsbergen) and its link to anoxia and acidification . (PDF) In: GSA Bulletin (Geological Society of America) . 127, No. 9-10, September 2015, pp. 1411-1421. doi : 10.1130 / B31216.1 .
- ↑ He Bin, Yi-Gang Xu, Xiao-Long Huang, Zhen-Yu Luo, Yu-Ruo Shi, Qi-Jun Yang, Song-Yue Yu: Age and duration of the Emeishan flood volcanism, SW China: Geochemistry and SHRIMP zircon U-Pb dating of silicic ignimbrites, post-volcanic Xuanwei Formation and clay tuff at the Chaotian section . (PDF) In: Earth and Planetary Science Letters . 255, March 2007, pp. 306-323. doi : 10.1016 / j.epsl.2006.12.021 .
- ↑ J. Gregory Shellnutt: The Emeishan large igneous province: A synthesis . In: Geoscience Frontiers (Elsevier) . 5, No. 3, August 2016, pp. 369-394. doi : 10.1016 / j.gsf.2013.07.003 .
- ↑ Stephen E. Grasby, Hamed Sanei, Benoit Beauchamp: Catastrophic dispersion of coal fly ash into oceans during the latest Permian extinction . (PDF) In: Nature Geoscience . 4, February 2011, pp. 104-107. doi : 10.1038 / ngeo1069 .
- ^ LT Elkins-Tanton, SE Grasby, BA Black, RV Veselovskiy, OH Ardakani, F. Goodarzi: Field evidence for coal combustion links the 252 Ma Siberian Traps with global carbon disruption . (PDF) In: Geology . 48, June 2020. doi : 10.1130 / G47365.1 .
- ↑ Henrik H. Svensen, Sergei Frolov, Grigorii G. Akhmanov, Alexander G. Polozov, Dougal A. Jerram, Olga V. Shiganova, Nikolay V. Melnikov, Karthik Iyer, Sverre Planke: Sills and gas generation in the Siberian Traps . In: Philosophical Transactions of the Royal Society A . 376, No. 2130, October 2018. doi : 10.1098 / rsta.2017.0080 .
- ↑ Michael M. Joachimski, Xulong Lai, Shuzhong Shen, Haishui Jiang, Genming Luo, Bo Chen, Jun Chen, Yadong Sun: Climate warming in the latest Permian and the Permian – Triassic mass extinction . (PDF) In: Geology . 40, No. 3, March 2012, pp. 195-198. doi : 10.1130 / G32707 .
- ↑ MO Clarkson, SA Kasemann, RA Wood, TM Lenton, SJ Daines, S. Richoz, F. Without Mueller, A. Meixner, SW Poulton, ET Tipper: Ocean acidification and the Permo-Triassic mass extinction . (PDF) In: Science . 348, No. 6231, April 2015, pp. 229-232. doi : 10.1126 / science.aaa0193 .
- ^ Yadong Sun, Michael M. Joachimski, Paul B. Wignall, Chunbo Yan, Yanlong Chen, Haishui Jiang, Lina Wang, Xulong Lai: Lethally Hot Temperatures During the Early Triassic Greenhouse . (PDF) In: Science . 338, No. 6105, October 2012, pp. 366-370. doi : 10.1126 / science.1224126 .
- ↑ Michael J. Benton, Richard J. Twitchett: How to kill (almost) all life: the end-Permian extinction event . (PDF) In: Trends in Ecology and Evolution . 18, No. 7, July 2003, pp. 358-365. doi : 10.1016 / S0169-5347 (03) 00093-4 .
- ↑ Jeffrey P. Benca, Ivo AP Duijnstee, Cindy V. Looy: UV-B-induced forest sterility: Implications of ozone shield failure in Earth's largest extinction . In: Science Advances . 4, No. 2, February 2018. doi : 10.1126 / sciadv.1700618 .
- ^ Seth D. Burgess, Samuel Bowring, Shu-Zhong Shen: High-precision timeline for Earth's most severe extinction . In: PNAS . 111, No. 9, March 2014, pp. 3316-3321. doi : 10.1073 / pnas.1317692111 .
- ↑ Shu-Zhong Shen, Jahandar Ramezani, Jun Chen, Chang-Qun Cao, Douglas H. Erwin, Hua Zhang, Lei Xiang, Shane D. Schoepfer, Charles M. Henderson, Quan-Feng Zheng, Samuel A. Bowring, Yue Wang , Xian-Hua Li, Xiang-Dong Wang, Dong-Xun Yuan, Yi-Chun Zhang, Lin Mu, Jun Wang, Ya-Sheng Wu: A sudden end-Permian mass extinction in South China . (PDF) In: GSA Bulletin (The Geological Society of America) . 131, September 2018, pp. 205-223. doi : 10.1130 / B31909.1 .
- ↑ a b Michael Benton, Andrew J. Newell: Impacts of global warming on Permo-Triassic terrestrial ecosystems . (PDF) In: Gondwana Research . 25, No. 4, May 2014, pp. 1308-1337. doi : 10.1016 / j.gr.2012.12.010 .
- ↑ Zhong-Qiang Chen, Michael J. Benton: The timing and pattern of biotic recovery following the end-Permian mass extinction . (PDF) In: Nature Geoscience . 5, No. 6, June 2012, pp. 375-383. doi : 10.1038 / ngeo1475 .
- ↑ Terrence J. Blackburn, Paul E. Olsen, Samuel A. Bowring, Noah M. McLean, Dennis V. Kent, John Puffer, Greg McHone, E. Troy Rasbury, Mohammed Et-Touhami: Zircon U-Pb Geochronology Links the End -Triassic Extinction with the Central Atlantic Magmatic Province . (PDF) In: Science . 340, No. 6135, May 2013, pp. 941-945. doi : 10.1126 / science.1234204 .
- ^ Tran T. Huynh, Christopher J. Poulsen: Rising atmospheric CO 2 as a possible trigger for the end-Triassic mass extinction . (PDF) In: Palaeogeography, Palaeoclimatology, Palaeoecology . 217, No. 3-4, February 2005, pp. 223-242. doi : 10.1016 / j.palaeo.2004.12.004 .
- ↑ Jessica H. Whiteside, Paul E. Olsen, Timothy Eglinton, Michael E. Brookfield, Raymond N. Sambrotto: Compound-specific carbon isotopes from Earth's largest flood basalt eruptions directly linked to the end-Triassic mass extinction . (PDF) In: PNAS . 107, No. 15, April 2010, pp. 6721-6725. doi : 10.1073 / pnas.1001706107 .
- ↑ JHFL Davies, H. Bertrand, N. Youbi, M. Ernesto, U. Schaltegger: End-Triassic mass extinction started by intrusive CAMP activity . (htlm) In: Nature Communications . May 8, 2017. doi : 10.1038 / ncomms15596 .
- ↑ Thea H. Heimdal, Henrik. H. Svensen, Jahandar Ramezani, Karthik Iyer, Egberto Pereira, René Rodrigues, Morgan T. Jones, Sara Callegaro: Large-scale sill emplacement in Brazil as a trigger for the end-Triassic crisis . (htlm) In: Nature Scientific Reports . January 8, 2018. doi : 10.1038 / s41598-017-18629-8 .
- ↑ Sylvain Richoz, Bas van de Schootbrugge, Jörg Pross, Wilhelm Püttmann, Tracy M. Quan, Sofie Lindström, Carmen Heunisch, Jens Fiebig, Robert Maquil, Stefan Schouten, Christoph A. Hauzenberger, Paul B. Wignall: Hydrogen sulphide poisoning of shallow seas following the end-Triassic extinction . (PDF) In: Nature Geoscience . 5, August 2012, pp. 662-667. doi : 10.1038 / NGEO1539 .
- ↑ G. Keller, T. Adatte, W. Stinnesbeck, M. Rebolledo-Vieyra, JU Fucugauchi, U. Kramar, Doris Stüben: Chicxulub impact predates the KT boundary mass extinction . In: PNAS . 101, No. 11, March 2004, pp. 3753-3758. doi : 10.1073 / pnas.0400396101 .
- ↑ Peter Schulte, Robert P. Speijer, Henk Brinkhuis, Agnes Kontny, Philippe Claeys, Simone Galeotti, Jan Smit: Comment on the paper "Chicxulub impact predates KT boundary: New evidence from Brazos, Texas" by Keller et al. (2007) . (PDF) In: Earth and Planetary Science Letters . 269, No. 3-4, May 2008, pp. 614-620. doi : 10.1016 / j.epsl.2007.11.06 .
- ^ Paul A. Renne, Alan L. Deino, Frederik J. Hilgen, Klaudia F. Kuiper, Darren F. Mark, William S. Mitchell III, Leah E. Morgan, Roland Mundil, Jan Smit: Time Scales of Critical Events Around the Cretaceous-Paleogene Boundary . (PDF) In: Science . 339, No. 6120, February 2013, pp. 684-687. doi : 10.1126 / science.1230492 .
- ↑ Johan Vellekoop, Appy Sluijs, Jan Smit, Stefan Schouten, Johan WH Weijers, Jaap S. Sinninghe Damsté, Henk Brinkhuis: Rapid short-term cooling Following the Chicxulub impact at the Cretaceous-Paleogene boundary . In: PNAS . 111, No. 21, May 2014, pp. 7537-7541. doi : 10.1073 / pnas.1319253111 .
- ↑ Stephen L. Brusatte, Richard J. Butler, Paul M. Barrett, Matthew T. Carrano, David C. Evans, Graeme T. Lloyd, Philip D. Mannion, Mark A. Norell, Daniel J. Peppe, Paul Upchurch, Thomas E. Williamson: The extinction of the dinosaurs . In: Biological Reviews, Cambridge Philosophical Society (Wiley Online Library) . 90, No. 2, May 2015, pp. 628–642. doi : 10.1111 / brv.12128 .
- ↑ a b c Michael J. Henehan, Andy Ridgwell, Ellen Thomas, Shuang Zhang, Laia Alegret, Daniela N. Schmidt, James WB Rae, James D. Witts, Neil H. Landman, Sarah E. Greene, Brian T. Huber, James R. Super, Noah J. Planavsky, Pincelli M. Hull: Rapid ocean acidification and protracted Earth system recovery followed the end-Cretaceous Chicxulub impact . In: PNAS . 116, No. 43, October 2019. doi : 10.1073 / pnas.1905989116 .
- ^ A b Douglas S. Robertson, Malcolm C. McKenna, Owen B. Toon, Sylvia Hope, Jason A. Lillegraven: Survival in the first hours of the Cenozoic . (PDF) In: Geological Society of America Bulletin . 116, No. 5/6, pp. 760-768. doi : 10.1130 / B25402 .
- ^ Douglas S. Robertson, William M. Lewis, Peter M. Sheehan, Owen B. Toon: Reevaluation of the heat-fire hypothesis . In: Journal of Geophysical Research: Biogeoscience . 110, No. 1, March 2013, pp. 329–336. doi : 10.1002 / jgrg.20018 .
- ↑ a b Julia Brugger, Georg Feulner, Stefan Petri: Baby, it's cold outside: Climate model simulations of the effects of the asteroid impact at the end of the Cretaceous . In: Geophysical Research Letters . 44, No. 1, January 2017, pp. 419-427. doi : 10.1002 / 2016GL072241 .
- ^ A b Nicholas R. Longrich, Tim Tokaryk, Daniel J. Field: Mass extinction of birds at the Cretaceous-Paleogene (K-Pg) boundary . In: PNAS . 108, No. 37, September 2011, pp. 15253-15257. doi : 10.1073 / pnas.1110395108 .
- ^ Paul R. Renne, Courtney J. Sprain, Mark A. Richards, Stephen Self, Loÿc Vanderkluysen, Kanchan Pande: State shift in Deccan volcanism at the Cretaceous-Paleogene boundary, possibly induced by impact . (PDF) In: Science . 350, No. 6256, October 2015, pp. 76-78. doi : 10.1126 / science.aac7549 .
- ↑ a b Pincelli M. Hull, André Bornemann, Donald E. Penman, Michael J. Henehan, Richard D. Norris, Paul A. Wilson, Peter Blum, Laia Alegret, Sietske J. Batenburg, Paul R. Bown, Timothy J. Bralower, Cecile Cournede, Alexander Deutsch, Barbara Donner, Oliver Friedrich, Sofie Jehle, Hojung Kim, Dick Kroon, Peter C. Lippert, Dominik Loroch, Iris Moebius, Kazuyoshi Moriya, Daniel J. Peppe, Gregory E. Ravizza, Ursula Röhl, Jonathan D. Schueth, Julio Sepúlveda, Philip F. Sexton, Elizabeth C. Sibert, Kasia K. Śliwińska, Roger E. Summons, Ellen Thomas, Thomas Westerhold, Jessica H. Whiteside, Tatsuhiko Yamaguchi, James C. Zachos: On impact and volcanism across the Cretaceous-Paleogene boundary . (PDF) In: Science . 367, No. 6475, January 2020, pp. 266-272. doi : 10.1126 / science.aay5055 .
- ↑ James S. Eldrett, Ian C. Harding, Paul A. Wilson, Emily Butler, Andrew P. Roberts: Continental ice in Greenland during the Eocene and Oligocene . (PDF) In: Nature . 446, March 2007, pp. 176-179. doi : 10.1038 / nature05591 .
- ↑ Michael Starkz, Wilfried Jokat, Gregor Knorr, Gerrit Lohmann: Threshold in North Atlantic-Arctic Ocean circulation controlled by the subsidence of the Greenland-Scotland Ridge . In: Nature Communications (online) . June 8, 2017. doi : 10.1038 / ncomms15681 .
- ↑ Mark Pagani, Matthew Huber, Zhonghui Liu, Steven M. Bohaty, Jorijntje Henderiks, Willem Sijp, Srinath Krishnan, Robert M. DeConton: The Role of Carbon Dioxide During the Onset of Antarctic Glaciation . (PDF) In: Science . 334, No. 6060, December 2011, pp. 1261-1264. doi : 10.1126 / science.1203909 .
- ↑ Becky Oskin: Russia's Popigai Meteor Crash Linked to Mass Extinction . livescience, June 13, 2014.
- ↑ Catalina Pimiento, John N. Griffin, Christopher F. Clements, Daniele Silvestro, Sara Varela; Mark D. Uhen, Carlos Jaramillo: The Pliocene marine megafauna extinction and its impact on functional diversity . (PDF) In: Nature ecology & evolution . 2017. doi : 10.1038 / s41559-017-0223-6 .
- ↑ Sander van der Kaars, Gifford H. Miller, Chris SM Turney, Ellyn J. Cook, Dirk Nürnberg, Joachim Schönfeld, A. Peter Kershaw, Scott J. Lehman: Humans rather than climate the primary cause of Pleistocene megafaunal extinction in Australia . In: Nature Communications . January 8, 2017. doi : 10.1038 / ncomms14142 .
- ↑ David L. Fox, Daniel C. Fisher, Sergey Vartanyan, Alexei N. Tikhonov, Dick Mol, Bernard Buigues: Paleoclimatic implications of oxygen isotopic variation in late Pleistocene and Holocene tusks of Mammuthus primigenius from northern Eurasia . (PDF) In: Quaternary International . 169-170, July 2007, pp. 154-165. doi : 10.1016 / j.quaint.2006.09.001 .
- ↑ RB Firestone, A. West, JP Kennett, L. Becker, TE Bunch, ZS Revay, PH Schultz, T. Belgya, DJ Kennett, JM Erlandson, OJ Dickenson, AC Goodyear, RS Harris, GA Howard, JB Kloosterman, P. Lechler, PA Mayewski, J. Montgomery, R. Poreda, T. Darrah, SS Que Hee, AR Smith, A. Stich, W. Topping, JH Wittke, WS Wolbach: Evidence for an extraterrestrial impact 12,900 years ago that contributed to the megafaunal extinctions and Younger Dryas cooling . In: Proceedings of the National Academy of Sciences (PNAS) . 103, No. 41, October 2007, pp. 16016-16021. doi : 10.1073 / pnas.0706977104 .
- ↑ Nicholas Pinter, Andrew C. Scott, Tyrone L. Daulton, Andrew Podoll, Christian Koeberl, R. Scott Anderson, Scott E. Ishman: The Younger Dryas impact hypothesis: A requiem . (PDF) In: Earth-Science Reviews (Elsevier) . 106, No. 3-4, June 2011, pp. 247-264. doi : 10.1016 / j.earscirev.2011.02.005 .
- ↑ Wendy S. Wolbach, Joanne P. Ballard, Paul A. Mayewski, Andrew C. Parnell, Niamh Cahill, Victor Adedeji, Ted E. Bunch, Gabriela Domínguez-Vázquez, Jon M. Erlandson, Richard B. Firestone, Timothy A. French, George Howard, Isabel Israde-Alcántara, John R. Johnson, David Kimbel, Charles R. Kinzie, Andrei Kurbatov, Gunther Kletetschka, Malcolm A. LeCompte, William C. Mahaney, Adrian L. Melott, Siddhartha Mitra, Abigail Maiorana- Boutilier, Christopher R. Moore, William M. Napier, Jennifer Parlier, Kenneth B. Tankersley, Brian C. Thomas, James H. Wittke, Allen West, James P. Kennett: Extraordinary Biomass-Burning Episode and Impact Winter Triggered by the Younger Dryas Cosmic Impact ∼12,800 Years Ago. 2. Lake, Marine, and Terrestrial Sediments . (PDF) In: The Journal of Geology . February 2018. doi : 10.1086 / 695704 .
- ↑ Andrew MT Moore, James P. Kennett, William M. Napier, Ted E. Bunch, James C. Weaver, Malcolm LeCompte, A. Victor Adedeji, Paul Hackley, Gunther Kletetschka, Robert E. Hermes, James H. Wittke, Joshua J. Razink, Michael W. Gaultois, Allen West: Evidence of Cosmic Impact at Abu Hureyra, Syria at the Younger Dryas onset (~ 12.8 ka): high-temperature melting at> 2200 ° C . In: Nature Scientific Reports . March 10, 2020. doi : 10.1038 / s41598-020-60867-w .
- ↑ Bradley D. Cramer, Daniel J. Condon, Ulf Söderlund, Carly Marshall, Graham J. Worton, Alan T. Thomas, Mikael Calner, David C. Ray, Vincent Perrier, Ian Boomer, P. Jonathan Patchett, Lennart Jeppsson: U -Pb (zircon) age constraints on the timing and duration of Wenlock (Silurian) paleocommunity collapse and recovery during the “Big Crisis” . (PDF) In: Geological Society of America (Bulletin) . 124, No. 11-12, October 2012, pp. 1841-1857. doi : 10.1130 / B30642.1 .
- ↑ Štěpán Manda, Jiří Frýda: Silurian-Devonian boundary events and their influence on cephalopod evolution: evolutionary significance of cephalopod egg size during mass extinctions . (PDF) In: Bulletin of Geosciences . 85, No. 3, 2010, pp. 513-540. doi : 10.3140 / bull.geosci.1174 .
- ↑ Alexander J. Hetherington, Joseph G. Dubrovsky, Liam Dolan: Unique Cellular Organization in the Oldest Root Meristem . In: Current Biology . 26, No. 12, June 2016, pp. 1629–1633. doi : 10.1016 / j.cub.2016.04.072 .
- ^ Peter Franks: New constraints on atmospheric CO 2 concentration for the Phanerozoic . (PDF) In: Geophysical Research Letters . 31, No. 13, July 2014. doi : 10.1002 / 2014GL060457 .
- ↑ Isabel P. Montañez, Jennifer C. McElwain, Christopher J. Poulsen, Joseph D. White, William A. DiMichele, Jonathan P. Wilson, Galen Griggs, Michael T. Hren: Climate, pCO 2 and terrestrial carbon cycle linkages during late Palaeozoic glacial – interglacial cycles . (PDF) In: Nature Geoscience . 9, No. 11, November 2016, pp. 824–828. doi : 10.1038 / ngeo2822 .
- ^ Georg Feulner: Formation of most of our coal brought Earth close to global glaciation . In: PNAS . 114, No. 43, October 2017, pp. 11333–11337. doi : 10.1073 / pnas.1712062114 .
- ↑ Borja Cascales-Miñana, Christopher J. Cleal: The plant fossil record reflects just two great extinction events . In: Terra Nova . 26, No. 3, 2013, pp. 195-200. doi : 10.1111 / ter.12086 .
- ^ William A. DiMichele, Neil J. Tabor, Dan S. Chaney, W. John Nelson: From wetlands to wet spots: Environmental tracking and the fate of Carboniferous elements in Early Permian tropical floras . (PDF) In: GSA (Geological Society of America) . Special Paper 399, 2006, pp. 223-248. doi : 10.1130 / 2006.2399 (11) .
- ↑ Sarda Sahney, Michael Benton, Howard J. Falcon-Lang: Rainforest collapse triggered Pennsylvanian tetrapod diversification in Euramerica . (PDF) In: Geology . 38, No. 12, November 2010, pp. 1079-1082. doi : 10.1130 / G31182.1 .
- ↑ Guillaume Dera, Benjamin Brigaud, Fabrice Monna, Rémi Laffont, Emmanuelle Pucéat, Jean-François Deconinck, Pierre Pellenard, Michael M. Joachimski, Christophe Durlet: Climatic ups and downs in a disturbed Jurassic world . (PDF) In: Geology . 53, No. 3, March 2011, pp. 215-218. doi : 10.1130 / G31579.1 .
- ↑ Federico Fanti, Tetsuto Miyashita, Luigi Cantelli, Fawsi Mnasri, Jihed Dridi, Michela Contessi, Andrea Cau: The largest thalattosuchian (Crocodylomorpha) supports teleosaurid survival across the Jurassic-Cretaceous boundary . (PDF) In: Cretaceous Research . 61, June 2016, pp. 263-274. doi : 10.1016 / j.cretres.2015.11.011 .
- ↑ Yongdong Wang, Huang Chengmin, Bainian Sun, Cheng Quan, Wu Jingyu, Zhicheng Lin: Paleo-CO 2 variation trends and the Cretaceous greenhouse climate . (PDF) In: Earth-Science Reviews . 129, February 2014, pp. 136–147. doi : 10.1016 / j.earscirev.2013.11.001 .
- ↑ Vanessa C. Bowman, Jane E. Francis, James B. Riding: Late Cretaceous winter sea ice in Antarctica? . (PDF) In: Geology . 41, No. 12, December 2013, pp. 1227-1230. doi : 10.1130 / G34891.1 .
- ↑ James S. Eldrett, Ian Jarvis, John S. Lignum, Darren R. Grätze, Hugh C. Jenkyns, Martin A. Pearce: Black shale deposition, atmospheric CO 2 drawdown, and cooling during the Cenomanian-Turonian Oceanic Anoxic Event . In: Paleoceanography and Paleoclimatology . 26, No. 3, September 2011. doi : 10.1029 / 2010PA002081 .
- ↑ James S. Eldrett, Chao Ma, Steven C. Bergman, Brendan Lutz, F. John Gregory, Paul Dodsworth, Mark Phipps, Petros Hardas, Daniel Minisini, Aysen Ozkan, Jahander Ramezani, Samuel A. Bowring, Sandra L. Kamo, Kurt Ferguson, Calum Macaulay, Amy E. Kelly: An astronomically calibrated stratigraphy of the Cenomanian, Turonian and earliest Coniacian from the Cretaceous Western Interior Seaway, USA: Implications for global chronostratigraphy . (PDF) In: Cretaceous Research . 56, pp. 316-344. doi : 10.1016 / j.cretres.2015.04.010 .
- ↑ Valentin Fischer, Nathalie Bardet, Roger BJ Benson, Maxim S. Arkhangelsky, Matt Friedman: Extinction of fish-shaped marine reptiles associated with reduced evolutionary rates and global environmental volatility . In: Nature Communinications . March 7, 2016. doi : 10.1038 / ncomms10825 .
- ^ Richard E. Zeebe, Andy Ridgwell, James C. Zachos : Anthropogenic carbon release rate unprecedented during the past 66 million years . (PDF) In: Nature Geoscience . 9, No. 4, April 2016, pp. 325–329. doi : 10.1038 / ngeo2681 .
- ↑ Donald E. Penman, Bärbel Hönisch , Richard E. Zeebe, Ellen Thomas, James C. Zachos: Rapid and sustained surface ocean acidification during the Paleocene-Eocene Thermal Maximum . (PDF) In: Oceanography . 29, No. 5, May 2014, pp. 357-369. doi : 10.1002 / 2014PA002621 .
- ^ Francesca A. McInerney, Scott L. Wing: The Paleocene-Eocene Thermal Maximum: A Perturbation of Carbon Cycle, Climate, and Biosphere with Implications for the Future . (PDF) In: Annual Review of Earth and Planetary Sciences . 39, May 2011, pp. 489-516. doi : 10.1146 / annurev-earth-040610-133431 .
- ^ Abigail RD Ambrosia, William C. Clyde, Henry C. Fricke, Philip D. Gingerich, Hemmo A. Abels: Repetitive mammalian dwarfing during ancient greenhouse warming events . (PDF) In: Science Advances . 3, No. 3, March 2017. doi : 10.1126 / sciadv.1601430 .
- ↑ Stephen GB Chester, Jonathan I. Bloch, Ross Secord, Doug M. Boyer: A New Small-Bodied Species of Palaeonictis (Creodonta, Oxyaenidae) from the Paleocene-Eocene Thermal Maximum . (PDF) In: Journal of Mammalian Evolution . 17, No. 4, December 2010, pp. 227-243. doi : 10.1007 / s10914-010-9141-y .
- ↑ Tatsuhiko Yamaguchi, Richard D. Norris, André Bornemann: Dwarfing of ostracodes during the Paleocene – Eocene Thermal Maximum at DSDP Site 401 (Bay of Biscay, North Atlantic) and its implication for changes in organic carbon cycle in deep-sea benthic ecosystem . (PDF) In: Palaeogeography, Palaeoclimatology, Palaeoecology . 346-347, No. 6384, August 2012, pp. 130-144. doi : 10.1016 / j.palaeo.2012.06.004 .
- ↑ William J. Ripple, Christopher Wolf, Thomas M. Newsome, Mauro Galetti, Mohammed Alamgir, Eileen Crist, Mahmoud I. Mahmoud, William F. Laurance and 15,364 life scientists from 184 countries: World Scientists' Warning to Humanity: A Second Notice . In: BioScience . tape 67 , no. 12 , 2017, p. 1026-1028 , doi : 10.1093 / biosci / bix125 .
- ↑ Klaus Jacob: The sixth catastrophe. In: Süddeutsche Zeitung , August 31, 2014.
- ↑ Gerardo Ceballos, Paul R. Ehrlich, Anthony D. Barnosky, Andrés García, Robert M. Pringle, Todd M. Palmer: Accelerated modern human-induced species losses: Entering the sixth mass extinction. In: Science Advances. 1, 2015, p. E1400253, doi : 10.1126 / sciadv.1400253 .
- ↑ wwf.de: Winner and Loser 2014
- ↑ European wild bird indicators and Trends of common birds in Europe, 2018 update. In: ebcc.info . January 21, 2019, accessed January 31, 2019 .
- ↑ Christian Mihatsch: The world is losing 380 animal and plant species every day . Badische Zeitung , October 6, 2014
- ↑ WWF Germany Press Office: Winners and Losers 2016 WWF: 2016 is a mixed year for species protection
- ↑ wwf.de: Living Planet Report 2016 (October 27, 2016)
- ↑ badische-zeitung.de , Panorama , October 28, 2016: Animal population has declined in Germany, but also worldwide (October 30, 2016)
- ↑ a b Südkurier : Ornithologist Peter Berthold: "Our birds are shitty"
- ↑ Alarming UN report: Up to a million species are threatened with extinction. In: nzz.ch . April 23, 2019, accessed April 24, 2019 .
- ↑ Humans displace a million animal and plant species , Süddeutsche Zeitung , May 6, 2019.
- ^ Francisco Sánchez-Bayo, Kris AG Wyckhuys: Worldwide decline of the entomofauna: A review of its drivers . In: Biological Conservation . 232, April 2019, pp. 8-27. doi : 10.1016 / j.biocon.2019.01.020 .
- ^ Mark C. Urban: Accelerating extinction risk from climate change . (PDF) In: Science . 348, No. 6234, May 2015, pp. 571-573. doi : 10.1126 / science.aaa4984 .
- ^ The State of World Fisheries and Aquaculture. Food and Agriculture Organization of the United Nations. 2016, ISBN 978-92-5-109185-2 .
- ^ CD Harvell, D. Montecino-Latorre et al. a .: Disease epidemic and a marine heat wave are associated with the continental-scale collapse of a pivotal predator (Pycnopodia helianthoides). In: Science Advances. 5, 2019, p. Eaau7042, doi : 10.1126 / sciadv.aau7042 .
- ^ A b Gregory P. Wilson: Mammals across the K / Pg boundary in northeastern Montana, USA: dental morphology and body-size patterns reveal extinction selectivity and immigrant-fueled ecospace filling . (PDF) In: Paleobiology . 39, No. 3, May 2013, pp. 429-469. doi : 10.1666 / 12041 .
- ↑ Evolution imposes 'speed limit' on recovery after mass extinctions. In: ScienceDaily. Accessed September 7, 2019 .
- ↑ Jens Boenigk, Sabina Wodniok: Biodiversity and Earth History . Berlin 2014, ISBN 978-3-642-55388-2 , p. 69.
- ↑ Jason S. Anderson, Tim Smithson, Chris F. Mansky, Taran Meyer, Jennifer Clack: A Diverse Tetrapod Fauna at the Base of 'Romer's Gap' . In: PLOS ONE . 10, No. 4, April 2015. doi : 10.1371 / journal.pone.0125446 .
- ↑ Manabu Sakamoto, Michael Benton, Chris Venditti: Dinosaurs in decline tens of millions of years before their final extinction . In: PNAS . 113, No. 18, May 2016, pp. 5036-5040. doi : 10.1073 / pnas.1521478113 .
- ↑ Zoltan Siki-Sava, Eric Buffetaut, Attila Ősi, Xabier Pereda-Suberbiola, Stephen L. Brusatte: Island life in the Cretaceous - faunal composition, biogeography, evolution, and extinction of land-living vertebrates on the Late Cretaceous European archipelago . In: ZooKeys . 469, January 2015, pp. 1–161. doi : 10.3897 / zookeys.469.8439 .
- ↑ Stephen L. Brusatte, Carlos RA Candeiro, Felipe M. Simbras: The last dinosaurs of Brazil: The Bauru Group and its implications for the end-Cretaceous mass extinction . In: Anais da Academia Brasileira de Ciências (Rio de Janeiro) . 89, No. 3. doi : 10.1590 / 0001-3765201720160918 .
- ↑ Nicholas R. Longrich, David M. Martill, Brian Andres: Late Maastrichtian pterosaurs from North Africa and mass extinction of Pterosauria at the Cretaceous-Paleogene boundary . In: PLOS Biology . March 2018. doi : 10.1371 / journal.pbio.2001663 .
- Jump up ↑ Robert A. DePalma, Jan Smit, David A. Burnham, Klaudia Kuiper, Phillip L. Manning, Anton Oleinik, Peter Larson, Florentin J. Maurrasse, Johan Vellekoop, Mark A. Richards, Loren Gurche, Walter Alvarez: A seismically induced onshore surge deposit at the KPg boundary, North Dakota . In: PNAS . 116, No. 17, April 2019, pp. 8190-8199. doi : 10.1073 / pnas.1817407116 .
- ↑ Katherine Kornei: Huge global tsunami followed dinosaur-killing asteroid impact . In: Eos . December 20, 2018, doi : 10.1029 / 2018EO112419 .
- ↑ Peter Wilf, Kirk R. Johnson: Land plant extinction at the end of the Cretaceous: a quantitative analysis of the North Dakota megafloral record . (PDF) In: Paleobiology . 30, September 2004, pp. 347-368. doi : 10.1666 / 0094-8373 (2004) 030 <0347: LPEATE> 2.0.CO; 2 .
- ↑ Jeffrey A. Fawcett, Steven Maere, Yves Van de Peer: Plants with double genomes might have had a better chance to survive the Cretaceous-Tertiary extinction event . In: PNAS . 106, No. 14, April 2009, pp. 5737-5742. doi : 10.1073 / pnas.0900906106 .
- ↑ Nicholas R. Longrich, Bhart-Anjan S. Bhullar, Jacques A. Gauthier: Mass extinction of lizards and snakes at the Cretaceous-Paleogene boundary . In: PNAS . 109, No. 52, December 2012, pp. 21396-21401. doi : 10.1073 / pnas.1211526110 .
- ↑ Stéphane Jouve, Nathalie Bardet, Nour-Eddine Jalil, Xabier Pereda Suberbiola, Baâdi Bouya, Mbarek Amaghzaz: The Oldest African Crocodylian: Phylogeny, Paleobiogeography, and Differential Survivorship Of Marine Reptiles Through the Cretaceous-Tertiary Boundary . (PDF) In: Journal of Vertebrate Paleontology . February 28, 2008, pp. 409-421. doi : 10.1671 / 0272-4634 (2008) 28 [409: TOACPP] 2.0.CO; 2 .
- ↑ Michael J. Henehan, Pincelli M. Hull, Donald E. Penman, James WB Rae, Daniela N. Schmidt: Biogeochemical significance of pelagic ecosystem function: an end-Cretaceous case study . In: Philosophical Transactions of the Royal Society B (Biological Sciences) . 171, No. 1694, May 2016. doi : 10.1098 / rstb.2015.0510 .
- ↑ Toby Tyrrell, Agostino Merico, David Ian Armstrong McKay: Severity of ocean acidification following the end-Cretaceous asteroid impact . In: PNAS . 112, No. 21, May 2015, pp. 6556-6561. doi : 10.1073 / pnas.1418604112 .
- ↑ Kunio Kaiho, Naga Oshima, Kouji Adachi, Yukimasa Adachi, Takuya Mizukami, Megumu Fujibayashi, Ryosuke Saito: Global climate change driven by soot at the K-Pg boundary as the cause of the mass extinction . In: Nature Scientific Reports . July 6, 2016. doi : 10.1038 / srep28427 .
- ↑ Michael P. Donovan, Ari Iglesias, Peter Wilf, Conrad C. Labandeira, N. Rubén Cúneo: Rapid recovery of Patagonian plant – insect associations after the end-Cretaceous extinction . In: Nature Ecology & Evolution . December 1, 2016. doi : 10.1038 / s41559-016-0012 .
- ^ Sierra V. Petersen, Andrea Dutton, Kyger C. Lohmann: End-Cretaceous extinction in Antarctica linked to both Deccan volcanism and meteorite impact via climate change . In: Nature Communications . July 7, 2016. doi : 10.1038 / ncomms12079 .
- ↑ Kunio Kaiho, Naga Oshima: Site of asteroid impact changed the history of life on Earth: the low probability of mass extinction . In: Scientific Reports . November 7, 2017. doi : 10.1038 / s41598-017-14199-x .
- ↑ GS Collin, N. Patel, TM Davison, ASP Rae, JV Morgan, SPS Gulick, IODP-ICDP Expedition 364 Science Party: GL Christeson, E. Chenot, P. Claeys, CS Cockell, MJL Coolen, L. Ferrière, C. Gebhardt, K. Goto, H. Jones, DA Kring, J. Lofi, CM Lowery, R. Ocampo-Torres, L. Perez-Cruz, AE Pickersgill, MH Poelchau, C. Rasmussen, M. Rebolledo-Vieyra, U . Riller, H. Sato, J. Smit, SM Tikoo, N. Tomioka, J. Urrutia-Fucugauchi, MT Whalen, A. Wittmann, L. Xiao, KE Yamaguchi, Third-Party Scientists: N. Artemieva, TJ Bralower: A steeply-inclined trajectory for the Chicxulub impact . In: Nature Communications . May 11, 2020. doi : 10.1038 / s41467-020-15269-x .