Luciferins

Luciferins (derived from the Latin lucifer , 'bringing light' ) are different natural substances that are used in various bioluminescent organisms to generate light. By catalytic activity of the corresponding luciferase - enzyme to react with oxygen ( oxidation ). During the change, mostly the splitting off of subgroups on the luciferin, energy is given off in the form of light . Both luciferins and luciferases are species or taxon-specific , that is, characteristic of each group of living beings.
history
As early as the 17th century, Robert Boyle observed that every bioluminescent system needed oxygen.
At the beginning of the 18th century, René Réaumur observed that powders of dried and ground bioluminescent organisms glow when water is added. The first reports of experimental work with luciferin-luciferase systems go back to the French Raphael Dubois . While working on fireflies in 1885, he discovered that a substance was consumed in a light-emitting reaction. In doing so, he extracted substances from various organisms that produce biological light, which are the cause of the glow and are not destroyed by heat, and he called them luciferin . He called luciferase, another component required for lighting that is heat-labile . Nowadays it is known that a taxon-specific luciferase is the enzyme that converts the associated taxon-specific luciferin. By mixing luciferin with luciferase in the presence of oxygen , Dubois was able to mimic natural bioluminescence .
The next investigations were carried out by Edmund Newton Harvey in the early 20th century. He found that there is a specificity in every luciferin-luciferase system. Luciferins of one species cannot be converted by the luciferase of another species. Great advances in structure elucidation were made from the 1950s by the Nobel Prize winner Osamu Shimomura .
properties
Bioluminescent systems are not evolutionarily conserved; the luciferases do not share any sequence homology . Luciferases occur in 17 different phyla and at least 700 mostly marine genera . Apparently they were often " invented "; phylogenetic studies indicated that luciferin-luciferase systems have more than 30 independent origins. The range of color emitted is between blue and red light (400 to 700 nm), with blue tones being the most common, while red colors are rarely observed. This is explained by the fact that most of the bioluminescent organisms are found in the sea and blue light penetrates the water furthest.
definition
According to the classic definition, the luciferin bound to the luciferase is the light emitter. The luciferase converts the luciferin while consuming oxygen; sometimes cofactors such as ATP or ions are required for this. The oxidized luciferin is initially in a transition state I and then - often after decarboxylation and further intermediate steps - reaches an electronically excited state P *. After a very short time (a few nanoseconds) this falls back to its ground state P and emits a light quantum during this time . The converted luciferins are usually also fluorophores , since they can get into an excited state by irradiating light.
Principles
From a biochemical point of view, a lot of energy is required to get into the excited state P *. The emission of photons with a wavelength of 500 nm (green, energy about 2 eV / photon) requires about 250 kJ / mol - for comparison: the hydrolysis of ATP to ADP and phosphate releases about 30 kJ / mol. In addition, the energy can only be released in one step. The energy is supplied by molecular oxygen when its relatively weak double bond is broken and a stronger bond (e.g. in CO 2 , 300 kJ / mol more stable) is formed.
The most common principle is the formation of a four-membered ring, a dioxetane or dioxetanone (α-peroxylactone). After the decarboxylation has taken place , the electronically excited state is formed.
Sometimes the fluorescence does not correspond to what is expected, for example in studies in vitro (in a test tube). There are various reasons for this. For example, luciferins bound to enzymes emit differently when oxidized than free luciferins when excited by light. Sometimes the energy is transferred to a second fluorophore, such as what happens with Aequorin to GFP in Aequorea victoria .
Quantum yield
Whether the conversion of a luciferin by the corresponding luciferase is efficient is determined by the quantum yield . The quantum yield is the number of light quanta emitted per converted luciferin molecule. A quantum yield of 1 would mean that for every converted molecule of luciferin, a light quantum is released. The highest quantum yield Q to date was found for the firefly luciferin from Photinus pyralis with Q = 0.41.
Luciferin species
Luciferin-luciferase systems come in numerous types. There are four main classes of luciferin-luciferase systems in which the luciferin is converted into an electronically excited state after conversion by a luciferase and thus becomes the actual light emitter.
The firefly luciferin, a benzothiazole

Bioluminescent insects are represented in the four orders of Collembola , Hemiptera , Coleoptera and Diptera . However, only the bioluminescence systems from organisms of the latter two orders were examined. In Coleoptera (beetles), representatives from three families can generate light: Phengodidae ( feather fireflies ), Elateridae ( click beetles ) and Lampyridae ( fireflies ).
The American firefly Photinus pyralis ( English firefly ) belongs to the Lampyridae family . It has already been used by Dubois for his studies on luciferine luciferases (see above). The first scientific studies of the bioluminescence reaction in P. pyralis were initiated by Harvey in 1917. In the meantime, this luciferin-luciferase system has been the best studied and is presented below.
Bioluminescent reaction

For the reaction, a luciferase converts the substrate D- luciferin (LH 2 ), a benzothiazole , with the consumption of oxygen (O 2 ). The work of William D. McElroy at the end of the 1940s showed that ATP and magnesium ions are required as cofactors for the reaction :
The ATP is converted into AMP and pyrophosphate (PP i ) and a light quantum (h ν ) is emitted.
Compared to luciferins from other systems (see below), the luciferin from fireflies is a relatively stable compound. The melting point is 205–210 ° C. Its molar extinction coefficient at 328 nm is ε = 18,200 M −1 cm −1 . This luciferin fluoresces and shows an emission maximum at λ max = 537 nm.
The firefly luciferase ( EC 1.13.12.7 ) has a molecular mass of approx. 60–62 kDa , in P. pyralis exactly 61 kDa, and is made up of 550 amino acids. It catalyzes the oxidative decarboxylation of luciferin to oxyluciferin (oxy-L, see also the figure below, box). The reaction takes place in the peroxisomes of the light organ cells. The structure of the P. pyralis luciferase was shown for the first time in 1956 with a resolution of 200 pm . For this analysis, large numbers of fireflies were collected by helpful children, who received a cent reward for each specimen delivered.
Without a bound substrate, the luciferase exists in an open conformation; a large N-terminal and a small C-terminal area form a deep groove. When the substrate is bound, a conformational change leads to the furrow closing. In the mid-1980s, luciferase was successfully incorporated into the genome of the E. coli bacterium and expressed there. Luciferases from fireflies of the Lampyridae family have a very similar structure. However, the differences determine the color of the light emitted. Depending on the type, the emission maximum λ max of the released light is between 530 nm (green) and 635 nm ( red ).
The reaction proceeds best in vitro at a pH of 7.8 and at a temperature of 23-25 ° C. In vivo , the color of the emitted light is yellow-green to yellow (552-582 nm). In the laboratory, the reaction shows a wider range of colors. In an acidic environment the light appears reddish (615 nm), in a neutral environment it appears green-yellow.
Reaction mechanism
The exact mechanism of the reaction is known. The D- luciferin is first adenylated at the carboxy group by ATP , with pyrophosphate being released as a leaving group ( 1 , see figure). Through this activation, the proton at the C4 atom can be abstracted, a carbanion is formed ( 2 ). The luciferin can then be oxygenated at the C4 atom, a linear hydroperoxide is formed ( 3 ). This forms a dioxetanone ring ( 4 ) with elimination of AMP . After decarboxylation , oxyluciferin is formed from it, which can be present either as a monoanion ( keto form , 5 ) or a dianion (enol form). In both cases the oxyluciferin is in an energetically excited state. It reverts to its basic state by releasing a photon (red light or yellow-green light). The oxyluciferin itself has not yet been isolated in its pure form because it is extremely unstable.
The reaction mechanism with formation of a dioxetanone was proven beyond doubt by the work of Shimomura at the end of the 1970s. Isotope-labeled 18 O was used in the reaction (H 2 18 O or 18 O 2 ). The results of this work replaced the previously postulated hypothesis that oxyluciferin is formed by linear bond cleavage. If this really happened, the released carbon dioxide would contain an oxygen atom that comes from water. In fact, it comes from the oxygen.
The luminous efficiency of this reaction is high, since the quantum efficiency Q is 0.41 at a pH of 8.5.
synthesis

How the insects - or microbial symbionts - produce the luciferin is not clearly understood. It is known that D- luciferin is not directly absorbed by the beetle (except for female beetles of the Photuris genus , which eat male fireflies of the Photinus genus ).
Two basic synthetic routes are discussed in the literature:
- One possibility is to recycle the oxyluciferin produced after the light reaction back to luciferin. The key component is that initially oxyluciferin is converted to 2-cyano-6-hydroxybenzothiazole (2C6HB), which is achieved by the luciferin-regenerating enzyme (LRE), e.g. B. in Photinus pyralis is catalyzed. 2C6HB then condenses with a D - cysteine to D - luciferin. This condensation reaction is also used in the chemical synthesis of luciferin (see figure on the right). An alternative route via 2C6HB assumes that this initially forms L- luciferin with L- cysteine . It is then racemized to D- luciferin via intermediate steps (see also figure on the left).
- Both possibilities of these biosynthetic pathways via 2C6HB still have some problems:
- The luciferin-regenerating enzyme is not overproduced in the light-producing organs of bioluminescent beetles. Since oxyluciferin is unstable in aqueous solutions, one would have to expect to find LRE in larger quantities there. In addition, the reactive 2C6HB can react not only with cysteine, but also with other metabolites. Furthermore, it is not clear where, for example, D- cysteine comes from and how one could discriminate between L- cysteine and D- cysteine. From L- cysteine, 2C6HB also reacts to L- luciferin. Although this can take up the luciferase as a substrate, it inhibits the light reaction. In addition, the enzyme that catalyzes the racemization has not yet been verified.
- It is also unclear how the beetles (or symbionts) can produce benzothiazolenes.
- In the case of the Japanese firefly Luciola lateralis , labeling experiments showed that the adult beetle synthesizes luciferin from hydroquinone or 1,4-benzoquinone . L- cysteine is attached twice to 1,4-benzoquinone , so that it forms the D or L form of luciferin. The racemerization of the L- into the D-form is still being investigated. Since 1,4-benzoquinone has a toxic effect in high concentrations, it is only released shortly before synthesis. For this purpose, the scientists suggest that the glucoside arbutin provides hydroquinone as a depot compound, which is then oxidized to 1,4-benzoquinone.
Evolutionary origins

It is possible that the luciferin-luciferase reaction in fireflies evolved from a completely different biological function. It is believed that the luciferin molecule only appeared in a later evolutionary outcome and led to a light reaction. This is supported by the fact that the luciferase can also efficiently condense coenzyme A onto the luciferin molecule and thus fulfills the function of a classic fatty acid CoA ligase . In this context, the luciferase can also use fatty acids such as arachidonic acid , which shares similar structural properties with the luciferin.
Because of this additional catalytic property, the original luciferase could have been a fatty acid CoA ligase. The occurrence of luciferin and the associated light reaction resulted in a selection advantage: the adenylation reaction had prevailed over time. This thesis was demonstrated using the non-luminescent mealworm Tenebrio molitor . This has no luciferin, but fatty acid CoA ligases. Interestingly, a light reaction can also be observed there by adding luciferin. But without knowing how firefly luciferin is biosynthesized, a more detailed evolutionary analysis is difficult.
Luminescence from other insects
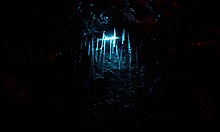
The firefly luciferin also occurs in luminescent insects of the other two families Phenogodidae and Elateroidae . The bioluminescence system made from feather fireflies (e.g. Phrixothrix, English railroad worm ) or click beetles (e.g. the firefly Pyrophorus noctilucus ) is almost identical to that made from fireflies . In the feathered fireflies only the larvae show bioluminescence, the adults do not.
In contrast, luminescent Diptera ( Diptera ) ( Arachnocampa or Orfelia ) not in common with the luciferin from fireflies. Although the 59 kDa luciferase from Arachnocampa luminosa belongs to the same protein family as that of the firefly (31% sequence identity), it cannot convert the firefly luciferin. This luciferase-luciferin system converts a quinoline- like luciferin into an ATP, Mg 2+ and oxygen-dependent reaction . Incidentally, the light emitted by the larvae of the North American fungus mosquito ( Orfelia fultoni ) is the bluest (λ max = 460 nm) that is generated by insects. These live for example in the Waitomo Caves .
Dehydroluciferin
It was shown in vitro that enzyme-bound, adenylated D- luciferin ( D -LH 2 · AMP) can also be converted in a dark reaction. This reacts with oxygen to form hydrogen peroxide and dehydroluciferin (L · AMP). The latter can be released from the luciferase using pyrophosphate, thereby producing ATP.
L · AMP is a potent inhibitor of luciferase. It is unknown whether the formation of dehydroluciferin also takes place under physiological conditions. At least the resulting hydrogen peroxide (H 2 O 2 ) in the peroxisomes could be detoxified quickly.
Tetrapyrrole, the luciferin of dinoflagellates and Euphausiidae
- Dinoflagellate bioluminescence caused by breaking the waves
Beach near Manasquan ( New Jersey , USA)
Image taken near Carlsbad (California, USA)
Picture taken on Seal Beach (California, USA)
The luciferin in this group corresponds to the chemical structure of a linear, open tetrapyrrole and is found among others in dinoflagellates ( Noctiluca , Gonyaulax , Pyrocystis ). Sea lights , which used to be incorrectly referred to as phosphorescence , are largely due to these unicellular algae. The investigations of the luciferin-luciferase system began in the late 1950s on the dinoflagellate Lingulodinium polyedrum (synonym: Gonyaulax polyedra ) through the work of J. Woodland Hastings and co-workers.
For light emission are next to the luciferin and a corresponding, approximately 135 kDa luciferase (LCF), a so-called luciferin-binding protein ( English luciferin-binding protein , LBP) is necessary. LBD is a homodimer , one subunit is 72 kDa. The luciferin in this family is extremely unstable at low pH values (<pH 4), high salt and even low oxygen concentrations. It could be shown that the LBD binds to the dinoflagellate luciferin at pH 8.0, but not at pH 6.3. This is intended to protect the substrate until the reaction occurs, which takes place best at pH 6.3, especially since the luciferase is inactive in a slightly alkaline environment (pH 8.0). For the conversion of the luciferin with oxygen it has been suggested that this takes place via several intermediate stages via radicals. The reaction itself takes place in special organelles , the so-called scintillons . These are on average 0.4 µM in size and mainly contain luciferases, luciferins and LBPs. The light that is produced during this reaction appears blue-greenish (excitation maximum at λ max = 390 nm, emission maximum at approx. Λ max = 470 nm). An enzyme-bound intermediate of the luciferin to be converted serves as the light emitter.

It has not yet been clarified whether luciferin is derived from chlorophyll a because of its relationship to chlorophyll a or whether it must first be built up gradually from several amino acids ( glycines and glutamic acids ). It is also paradoxical that the oxy-luciferin produced in the light reaction is not a fluorophore.
Component F in krill
A luciferin with almost identical structure was also found in luminescent Euphausiidae ( krill ), e.g. B. Meganyctiphanes norvegica or Euphausia pacifica . There it is referred to as component F , which is ingested through food. The reaction mechanism corresponds to that of the dinoflagellates.
Flavin, a bacterial luciferin
Luminescent bacteria use reduced flavin mononucleotide (FMNH 2 , also known as riboflavin-5-phosphate) for a light-emitting reaction. They occur either - less often - terrestrially ( Vibrio , Xenorhabdus , Photorhabdus ) or freely living in the sea ( Beneckea , Vibrio ) or symbiotic or parasitic in or on other animals. They are responsible for the bioluminescence of many glowing deep-sea fish, where they are kept as symbionts in special lighting organs ( photobacterium ). After all, bioluminescent bacteria can also be found in the nutrient cycle: in the middle depths of the ocean, they adhere to the feces that attract fish through the bioluminescence of those bacteria. After ingestion, they find better conditions for survival in the fish intestine. The bioluminescent bacteria identified so far are all Gram- negative, rod-shaped, mobile and facultatively anaerobic. A known bacterium with bioluminescent properties is, for example, Aliivibrio fischeri . Bioluminescent bacteria have been identified in the families Vibrionaceae , Shewanellaceae and Enterobacteriaceae , and there under the genera Vibrio , Photobacterium , Aliivibrio , Photorhabdus and Shewanella .
The study of bacterial bioluminescence led to particular advances in the 1950s. The researchers Milton J. Cormier and Bernard L. Strehler found out that four factors are necessary for the bioluminescence reaction: In addition to FMNH 2 , a luciferase, molecular oxygen and a long-chain aldehyde of a saturated hydrocarbon are required. The aldehyde, hexadecanal , was known as the “ kidney cortex factor ” prior to its chemical identification because the aldehyde was isolated from the adrenal cortex of pigs . Other aldehydes can also be used for the light reaction, for example decanal or dodecanal . The following table shows the composition from 40 g of isolated bacteria. It is assumed that mainly tetradecanal is implemented.
aldehyde | P. phosphoreum | A. fischeri |
---|---|---|
10C atoms ( decanal ) | <1 nmol | <1 nmol |
11C atoms | <1 nmol | <1 nmol |
12C atoms ( dodecanal ) | 30 nmol | 32 nmol |
13C atoms | 6 nmol | 2 nmol |
14C atoms ( tetradecanal ) | 380 nmol | 29 nmol |
15C atoms | 6 nmol | 6 nmol |
16C atoms ( hexadecanal ) | 180 nmol | 18 nmol |
17C atoms | <1 nmol | 2 nmol |
18C atoms | <1 nmol | <1 nmol |
FMNH 2 and the aldehyde are converted into FMN and a carboxylic acid, depending on the oxygen , according to (see also figure):
This reaction is catalyzed by a bacterial luciferase, a flavin-dependent monooxygenase . Since this simultaneously oxidizes the aldehyde to the carboxylic acid, it is an oxidase with a mixed function. In all luminescent bacteria, the luciferase is a heterodimer with a size of 76 ± 4 kDa . It is composed of an α and β protein subunit (40–42 kDa and 37–39 kDa, respectively), which when separated show hardly any activity. The catalytic center is likely located in the α-subunit. The β subunit is believed to be necessary for stability, structure and quantum yield. The luciferase is active at pH values between 6.0–8.5 ( Photobacterium phosphorerum , A. fischeri ) or 6.0–9.5 ( Benecka harveyi ), but not at temperatures above 30–35 ° C. A crystal structure of the luciferase from Vibrio harveyi was solved with a resolution of 150 pm .
FMNH 2 in free solution is unstable and easily oxidizes. However, when it is enzyme-bound, its stability increases and is attacked nucleophilically by oxygen at position C4a . This creates a 4a hydroperoxide , which is unusually stable. This reacts with the aldehyde to form a peroxyhemiacetal, which ultimately breaks down into a fatty acid and a 4a-hydroxyflavin. The latter is in an excited state and reverts to its basic state when it emits light. The 4a-hydroxyflavin is thus the actual light emitter. In the ground state, this is hydrolyzed to FMN.
During the reaction catalyzed by the luciferase, blue-green light is emitted, which in vitro has an emission maximum at approx. Λ max = 490 nm. In vivo , however, wavelength maxima of 472 nm to 545 nm were observed. The reason for this is the transfer of the excitation energy to fluorescent proteins via FRET . Two classes of fluorescent proteins have been identified: blue fluorescent lumazine proteins (LumPs) with lumazine as the chromophore ( P. phosphoreum , A. fischeri ). Alternatively, the yellow fluorescent proteins (YFPs) form the second class, which have FMN or riboflavin as chromophore ( A. fischeri strain Y-1). With the LumPs, the emission maximum shifts from 490 nm to 476 nm, with YFPs from 484 nm to 534 nm. For energy transfer according to FRET, the luciferin-luciferase complex must be bound to the respective fluorescent proteins. The quantum yield is 0.10-0.16.
The FMNH 2 for the bioluminescence reaction is obtained from riboflavin ( vitamin B2 ) by a riboflavin kinase with consumption of ATP . After the reaction, however , FMNH 2 is regenerated from FMN, which catalyzes a flavin reductase with NAD (P) H consumption. Since the amount of aldehydes occurring in the bacterial cell (see table above) is only sufficient for a very short bioluminescence, the aldehydes are constantly being regenerated. Here, the long-chain aldehyde is recovered from the fatty acid formed in the reaction, which is catalyzed in the so-called fatty acid reductase complex with consumption of ATP and NAD (P) H.
The bioluminescence reaction consumes a lot of energy, since two molecules of NAD (P) H and one molecule of ATP are required to regenerate the components. As a result, this light reaction must be appropriately controlled. In addition, flavin reductases have a higher turnover rate than luciferase. Too much FMNH 2 is produced in uncontrolled activity . Because of its rapid oxidation (see above), a lot of NAD (P) H would be wasted. This emphasizes the need for regulation.
Lux genes
All proteins that have something to do with the bioluminescence reaction are encoded by so-called lux genes (lat. Lux light). The subunits of the luciferase are encoded by the luxA and luxB genes, the luxB gene probably being created by gene duplication from the luxA gene (30% sequence identity). LuxA and LuxB have already been successfully cloned as markers . Lux C, D and E code for the fatty acid reductase complex , here lux C for an NADPH-dependent acyl protein reductase (about 54 kDa), lux D for an acyl transferase (about 33 kDa) and lux E for an acyl protein synthetase (about 42 kDa).
The lux genes are located on an operon that is regulated by a single promoter . The arrangement of the genes ( luxCDABE ) among the bioluminescent bacteria is preserved. In addition, some bioluminescent bacteria have another gene on the lux operon, luxG , which codes for a flavin reductase . Other genes have been identified within the lux operon or in a neighboring operon ( luxR ), such as B. luxF, ribEBHA or luxI .
The lux genes from A. fischerii are used for in vitro experiments .
Coelenterazine, the chemical component common to many marine bioluminescent organisms

The luciferin coelenterazine was discovered through the work of Milton J. Cormier on the sea feather species Renilla reniformis and Frank H. Johnson on the jellyfish A. victoria . This is very widespread among bioluminescent marine organisms, for example among members of the cnidarians ( Cnidaria ), rib jellyfish ( Ctenophora ), molluscs ( Molusca ), arthropods ( Arthropoda ) and chordates ( Chordata ). Coelenteratzine was not discovered in terrestrial organisms or annelids ( Annelida ). Sometimes they are also found in small amounts in non-bioluminescent organisms, such as the fire sponge Microcina prolifera . This also does not contain any luciferase.
Coelenterazine has an aminopyrazine basic structure and is present as a light-emitting component as bona fide luciferin. Often it is also bound as a chromophore in photoproteins such as B. Aequorin , Obelin or Symplectin . Coelenterazine derivatives are also used by numerous marine organisms.
Unmodified, coelenterazine is hardly stable in neutral aqueous solutions, where it is easily oxidized by atmospheric oxygen. It is more stable in methanol. There it fluoresces yellowish ( ε = 9800 M −1 · cm −1 , λ max = 435 nm). In general, coelenterazines react with oxygen to form coelenteramides. Decarboxylation occurs here and the anion of a coelenteramide is formed. This is also the light emitter, so that generally blue light is emitted. This reaction can be biocatalyzed (bioluminescence), but also takes place spontaneously ( chemiluminescence ). The bioluminescent response is explained in the following paragraph.
mechanism
In 1962 the photoprotein aequorin was isolated from Aequorea victoria and in 1974 coelenterazine was identified as the luciferin. How the biochemical mechanisms for the luciferin-luciferase system with imidazole pryazines now take place was shown in 2000 using A. victoria . Aequorin plays an essential role here. Aequorin is a small photoprotein and is located on the edge of the screen in the jellyfish. With Aequorin, the luciferin ( coelenterazine ) is already connected to the protein part by a peroxide bridge. As a result, the photoprotein already carries the oxidizing agent O 2 with it. In enzyme-bound form, coelenterazine can also be stored for a longer period of time. Aequorin has three binding sites for calcium ions. When calcium ions bind to it, the conformation of the protein changes in such a way that an intramolecular reaction with the coelenterazine is triggered. This initially reacts to form an unstable dioxetanone ring, so that after splitting off CO 2 , the anion of coelenteramide is finally formed. After relaxation into the ground state, a light quantum with a wavelength of λ max = 465 nm is emitted. Because of this blue glow, the protein is also known as the blue fluorescent protein (BFP). The photoprotein is finally regenerated in the presence of coelenterazine and molecular oxygen.
Aequorea victoria does not fluoresce blue, but green. This is because the blue fluorescent protein transfers the energy of the bioluminescence reaction without radiation to the so-called green fluorescent protein ( GFP ).
Watasenia luciferin
The bioluminescent deep-sea octopus Watasenia scintillans was first described in 1905 (then still as Abraliopsis scintillans ). He has numerous photophores all over his body that glow bluish like a starry sky. A modified coelenterazine is required for the bioluminescence reaction. This is a disulfate of coelenterazine and was isolated from the liver of the squid in 1976. It is known as Watasenia luciferin. In neutral aqueous solutions it is unstable and tends to auto-oxidize ( chemiluminescence ), which is induced in particular by the presence of hydrogen peroxide and iron (II) ions. The luciferin is strongly fluorescent in aqueous solutions (λ max = 400 nm).
The Watasenia luciferin is converted by a not yet isolated, membrane-bound luciferase, as a result of which blue light is emitted (λ max = 470 nm). The reaction has an optimum pH of 8.8 and an optimum temperature of 5 ° C and, in addition to molecular oxygen, requires ATP and Mg 2+ . The quantum yield is 0.36. For the reaction mechanism, it was suggested that the luciferin is adenylated by means of ATP so that it can bind to the luciferase. The further course of the reaction includes the formation of a dioxetanone ring and finally that of the coelenteramide anion, as generally shown above. This creates light between 400 and 580 nm (λ max = 470 nm).
Vargula luciferin
The mussel crabs of the species Vargula hilgendorfii (also known as Cypridina hilgendorfii until 1962 ) excrete a luminescent liquid into the seawater if they feel threatened. Biochemical studies on the luciferase-luciferin system contained in these shellfish were initiated by Harvey at the beginning of the 20th century. It has now been carefully examined.
The luciferin, vargulin , was isolated in 1957 and identified as the imidazole pryazine component in 1966. It is soluble in water, methanol and alcoholic solvents. Vargulin has a yellow color in neutral solutions and shows an absorption maximum in methanol at λ max = 432 nm with a molar extinction coefficient of ε = 9000 M −1 · cm −1 . It is also slightly fluorescent in aqueous solutions (excitation maximum at λ max = 540 nm). Vargulin is very unstable and is particularly oxidized by atmospheric oxygen, but also by lead (IV) oxide . Light can also be emitted, so that a chemiluminescent reaction is present here - especially in organic solvents such as diglyme .
In the mussel crabs, vargulin is converted to coelenteramide, oxyluciferin, by a luciferase, with blue light being released ( λ max = 463 nm). The luciferase is a 60-70 kDa monomer with 555 amino acids. It is high in cysteines and is an acidic protein ( isoelectric point of 4.35).
During the bioluminescence reaction, vargulin is bound to the luciferase and oxygenated at the C2 atom. This creates a peroxide anion which cyclizes to form the dioxetanone ring. This decarboxylates spontaneously and forms the anion of the coelenteramide, which is in an excited state. After a light quantum has been emitted, it reverts to its basic state and oxyluciferin is released. The light emitter of the reaction is the oxyluciferin bound to the luciferase. The quantum yield is temperature and pH dependent and is Q = 0.30. Etioluciferin is also formed as a side reaction of 10 to 15%, but no light is emitted.
As early as 1966 it was assumed that that luciferin is composed of L - arginine , L - isoleucine and L - tryptophan . There are now more and more indications for this.
Dehydrocoelenterazine from Symplectoteuthis oualaniensis
Symplectoteuthis oualaniensis (Japanese name Tobi-ika ) is asquid widespreadin the Pacific and Indian Oceans . The first study of its bioluminescence was published in 1981. The squid converts dehydrocoelenterazine through a special photoprotein, which is called " symplectin ". As with other photoproteins (aequorin, obelin) it is present there as a chromophore covalently bound via a cysteine. The light emitted during the conversion is bluish; various emission maxima λ max were specified (456 nm, 470 nm, 480 nm). Bound dehydrocoelenterazine is oxygenated at the C2 position; after the bioluminescence reaction, coelenteramide and apo “symplectin” are formed. The latter is regenerated to “symplectin” by a molecule of dehydrocoelenterazine.
The closely related squid Symplectoteuthis luminosa (Japanese name Suji-ika ) also shows bioluminescence. The chemical components involved and the mechanism of the bioluminescence reaction are similar to those of S. oualaniensis . Large amounts of dehydrocoelenterazine can be isolated from the liver of the squid.
Bioluminescent mushrooms

Since the ancient times are bioluminescent mushrooms known whose fruiting bodies produce a constant glow. Bioluminescence was generated in 1959 in the mycelium of the fungi Collybia velutipes and Armillaria mellea by combining cold and cooked extracts with the addition of NADPH.
In 2015, hispidine was identified as a luciferin precursor in the fungus Pholiota squarrosa . This is converted by a soluble NADP (H) -dependent hydroxylase to luciferin 3-hydroxyhispidine (( E ) -6- (3,4-dihydroxystyryl) -3,4-dihydroxy-2H-pyran-2-one), thereby O 2 and NADP (H) consumed. The luciferin thus formed is oxidized by luciferase with oxygen, which emits visible light. Isolation of the underlying luciferin is difficult because of its instability. The oxy-luciferin formed is a derivative of caffeic acid , which is converted into caffeic acid after hydrolytic cleavage of pyruvate and is used as a starting material for the synthesis of hispidine.
At least 100 species of fungi of the order Agaricales use the same biochemical reaction to generate light. The ecological significance is not yet known, presumably the light is supposed to attract insects, which then spread the fungal spores.
Non-classical luciferin-luciferase systems
The Latia Luciferin
The luciferin found in New Zealand's freshwater snail ( Latia neritoides ) is a terpenoid aldehyde and is known as Latia luciferin. The luciferin is highly hydrophobic , fat-soluble and a colorless liquid. Its absorption maximum is λ max = 207 nm, the molar extinction coefficient is 13.700 M −1 . Because it is unstable, it can spontaneously hydrolyze to formic acid and an aldehyde. However, the latter is not active for the bioluminescence reaction. If the enol formyl group is replaced by an enol ether group, the luciferin is also no longer active.
The Latia luciferin is catalytically converted into a ketone ( oxy- luciferin), which is catalyzed by a 173 kDa, colorless and non-fluorescent luciferase ( EC 1.14.99.21 ). It is a homohexamer, the individual subunits are approx. 30 kDa in size.
In addition to the luciferin, the luciferase and oxygen, a cofactor, the 39 kDa purple protein, is required for the reaction . This is red fluorescent and seems to be a kind of activator for the bioluminescence reaction. However, it is not absolutely necessary for this, as it can be replaced by ascorbate and NADH, for example. The bioluminescence reaction can take place even without the purple protein. The reaction creates an oxidized luciferin molecule and two formic acid molecules from one molecule of luciferin, oxygen and water:
This releases light, the emission maximum of which is λ max = 536 nm. Therefore, the slime of this snail, which z. B. secreted after mechanical stimuli, light green. The reaction efficiency of this bioluminescence reaction is very low, since the quantum yield Q is approx. 0.003 (25 ° C) or 0.0068 (8 ° C). To increase the quantum yield, NADH (0.25 mM) and ascorbate (1 mM) can be added to the reaction, so that it rises to 0.009 (25 ° C). However, this creates other reaction products, which is represented by the following equation:
Whether a dioxetane ring is formed as an intermediate in the reactions is still under discussion. In contrast to the oxy-luciferin of the firefly, the resulting oxy-luciferin is not a fluorophore. It is assumed that the energy released during this reaction is transferred to the actual emitter, a protein-bound flavin or a flavin-like group.
Luciferin from Diplocardia longa
The luciferin of the Diplocardia longa worm is a simple aldehyde, N -isovaleryl-3-aminopropanal. This is soluble in polar solvents ( methanol , ethanol , acetone , methyl acetate ), but not in non-polar solvents such as hexane or carbon tetrachloride . The special thing about the bioluminescence reaction is the fact that hydrogen peroxide is required instead of molecular oxygen. The corresponding luciferase, an approximately 300 kD large, strongly asymmetric enzyme, then converts the activated form, a peroxide adduct. The luciferase probably needs copper, blue-green light is emitted (λ max = 507 nm). However, it is not known what purpose bioluminescence can have in worms in general. Here, too, the actual emitter of the bioluminescence reaction still has to be identified.
The quantum yield of this reaction is very low with Q = 0.002.

Luciferin from Fridericia heliota
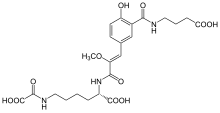
In Siberia , Fridericia heliota , a small (15 mm long, 0.5 mm wide, 2 mg heavy) white-yellowish looking earthworm, a blue bioluminescence (λ max = 478 nm) was discovered in the little bristle . This occurs after contact or mechanical irritation in the area of its epidermal cells. The luciferin-luciferase system is unique, it does not react with any other known system. In addition to oxygen, ATP and Mg 2+ are required for the reaction .
During the bioluminescence reaction, the lysine residue is oxidatively decarboxylated. The response is likely to be similar to that of firefly luciferin. As an initial reaction, ATP binds to the lysine residue, followed by a dioxetanone ring.
Applications
Diagnosis
With the help of the luciferin-luciferase system from fireflies, the presence of ATP in samples can be quickly checked. This is used, for example, in the food industry to detect bacterial contamination. ATP occurs only in living organisms, which can be detected in food through the bioluminescence reaction.
Since Aequorin's light reaction is dependent on calcium ions, this system can measure the concentration of calcium ions. The first application dates back to 1967, when intracellular changes in calcium concentrations in muscle cells were detected with the aid of aequorins. After cloning aequorin in bacteria, it was possible to measure the calcium concentration of bacterial cytosol. It is also possible to clone aequorin in eukaryotic cells. In transgenic plants , for example, it was possible to measure the change in the cytosolic calcium concentration after touching the plant or after a cold shock.
Genetic engineering / biotechnology
Luciferases are often used as markers in molecular biology : organisms that have received the gene and incorporated it into their genome light up when they receive luciferin. In this way, it can be demonstrated whether genes that one would like to introduce into organisms are actually expressed. To do this, the gene to be expressed is linked to a gene that codes for a luciferase. Such a reporter gene can also be used to identify promoter regions in the genome. The luciferase genes from Photinus pyralis and Renilla reniformis are mostly used commercially . Both enzymes are often used in the same approach ( dual luciferase assay ).
It is also possible to use the light reaction to measure protein-protein interactions , signals during signal transduction processes and the activity of cellular receptors.
The use of luciferase reporters has also been established for living animal model organisms ( bioimaging ). In the field of cancer research , markers can be used to track the growth of tumors or the formation of metastases . In addition, protein expression can be visualized in living animals using luciferase-luciferin systems.
See also
literature
- Osamu Shimomura : Bioluminescence: Chemical Principles and Methods. Word Scientific Publishing Company, 2006, ISBN 981-256-801-8 .
- T. Wilson, JW Hastings: Bioluminescence. In: Annu. Rev. Cell Dev. 14, 1998, pp. 197-230. PMID 9891783 .
- LF Greer, AA Szalay: Imaging of light emission from the expression of luciferases in living cells and organisms: a review. In: Luminescence. 17, 2002, pp. 43-74. PMID 11816060 ; doi: 10.1002 / bio.676 .
- K. Teranishi: Luminescence of imidazo [1,2- a ] pyrazin-3 (7H) -one compounds. In: Bioorg Chem. 35 (1), 2007, pp. 82-111. PMID 17007903 .
- Thérèse Wilson, J. Woodland Hastings: Bioluminescence: Living Lights, Lights for Living . Harvard University Press, 2013, ISBN 978-0-674-06716-5 .
- Aleksandra S. Tsarkova, Zinaida M. Kaskova, and Ilia V. Yampolsky: A Tale Of Two Luciferins: Fungal and Earthworm New Bioluminescent Systems . In: Accounts of Chemical Research . tape 49 , no. 11 , November 15, 2016, p. 2372-2380 , doi : 10.1021 / acs.accounts.6b00322 .
Web links
- Luciferin-luciferase systems (Engl.)
- Luminescence - Chemiluminescence - Bioluminescence by Dieter Weiß (Uni Jena)
-
Overview page (American Society for Photobiology)
- Bruce R. Branchini: Chemistry of Firefly Bioluminescence.
- J. Woodland Hastings, Wayne Schultz, d Liyun Liu: Dinoflagellate Bioluminescence.
- Leo Yen-Cheng Lin, Edward A. Meighen: Bacterial Bioluminescence.
- Vadim R. Viviani: Terrestrial Bioluminescence.
Individual evidence
- ^ Karl Ernst Georges : Comprehensive Latin-German concise dictionary . 8th, improved and increased edition. Hahnsche Buchhandlung, Hanover 1918 ( zeno.org [accessed September 24, 2019]).
- ↑ Waldemar Adam: Biological light . In: Chemistry in Our Time . tape 7 , no. 6 , 1973, p. 182-192 , doi : 10.1002 / ciuz.19730070605 .
- ↑ EN Harvey: Bioluminescence . Academic, New York 1952.
- ^ JW Hastings: Bioluminescence. In: N. Sperelakis (Ed.): Cell Physiology. 3. Edition. Academic Press, New York 2001, pp. 1115-1131.
- ^ PJ Herring: Systematic distribution of bioluminescence in living organisms. In: J Biolumin Chemilumin. 1 (3), 1987, pp. 147-163. PMID 3503524 .
- ↑ a b c d e f g h i j k Eveline Brodl, Andreas Winkler, Peter Macheroux: Molecular Mechanisms of Bacterial Bioluminescence . In: Computational and Structural Biotechnology Journal . tape 16 , November 15, 2018, ISSN 2001-0370 , p. 551-564 , doi : 10.1016 / j.csbj.2018.11.003 , PMID 30546856 , PMC 6279958 (free full text).
- ↑ Schmidt-Rohr, K. (2020). "Oxygen Is the High-Energy Molecule Powering Complex Multicellular Life: Fundamental Corrections to Traditional Bioenergetics" ACS Omega 5 : 2221-2233. Http://dx.doi.org/10.1021/acsomega.9b03352
- ↑ EH White et al. : The chemi- and bioluminescence of firefly luciferin: an efficient chemical production of electronically excited states. In: Bioorg. Chem. Vol. 1, No. 1-2, 1971, pp. 92-122, doi: 10.1016 / 0045-2068 (71) 90009-5 .
- ↑ a b c d Osamu Shimomura, pp. 1ff.
- ↑ External identifiers of or database links to D- luciferin : CAS number: 2591-17-5, EC number: 219-981-3 , ECHA InfoCard: 100.018.166 , PubChem : 5484207 , ChemSpider : 4588411 , Wikidata : Q3265801 .
- ↑ GA Keller et al. : Firefly luciferase is targeted to peroxisomes in mammalian cells. In: Proc Natl Acad Sci USA . 84 (10), 1987, pp. 3264-3268. PMID 3554235 ; PMC 304849 (free full text, PDF).
- ↑ H. Fraga: Firefly luminescence: a historical perspective and recent developments. In: Photochem Photobiol Sci. 7 (2), 2008, pp. 146-158. PMID 18264582 ; doi: 10.1039 / b719181b .
- ↑ a b J. C. Day et al. : Evolution of beetle bioluminescence: the origin of beetle luciferin. In: Luminescence. 19 (1), 2004, pp. 8-20. PMID 14981641 ; doi: 10.1002 / bio.749 .
- ^ A b c J. W. Hastings: Chemistries and colors of bioluminescent reactions: a review. In: Genes. 173 (1 Spec No), 1996, pp. 5-11. PMID 8707056 ; doi: 10.1016 / 0378-1119 (95) 00676-1 .
- ↑ O. Shimomura et al. : Source of oxygen in the CO 2 produced in the bioluminescent oxidation of firefly luciferin. In: Proc Natl Acad Sci USA. 74 (7), 1977, pp. 2799-2802. PMID 16592418 ; PMC 431296 (free full text, PDF).
- ↑ M. DeLuca, ME Dempsey: Mechanism of oxidation in firefly luminescence. In: Biochem Biophys Res Commun . 40 (1), 1970, pp. 117-122. PMID 5456946 ; doi: 10.1016 / 0006-291X (70) 91054-5 .
- ↑ FI Tsuji et al .: Mechanism of the enzyme-catalyzed oxidation of Cypridina and firefly luciferins studied by means of 17 O 2 and H 2 18 O. In: Biochem Biophys Res Commun. 74 (2), 1977, pp. 606-613. PMID 836314 ; doi: 10.1016 / 0006-291X (77) 90346-1 .
- ↑ Y. Ando et al. : Firefly bioluminescence quantum yield and color change by pH-sensitive green emission. In: Nat. Photonics. 2, 2008, pp. 44-47; doi: 10.1038 / nphoton.2007.251 .
- ↑ T. Eisner et al. : Firefly "femmes fatales" acquire defensive steroids (lucibufagins) from their firefly prey. In: Proc Natl Acad Sci USA. 94 (18), 1997, pp. 9723-9728. PMID 9275191 ; PMC 23257 (free full text, PDF).
- ↑ K. Gomi, N. Kajiyama: Oxyluciferin, a luminescence product of firefly luciferase, is enzymatically regenerated into luciferin. In: J Biol Chem . 276 (39), 2001, pp. 36508-36513. PMID 11457857 ; PDF (free full text access)
- ↑ K. Niwa et al .: Stereoisomeric bio-inversion key to biosynthesis of firefly D-luciferin. In: FEBS Lett . 580 (22), 2006, pp. 5283-5287. PMID 16979628 ; doi: 10.1016 / j.febslet.2006.08.073 .
- ↑ N. Lembert: Firefly luciferase can use L-luciferin to produce light. In: Biochem J . 317 (Pt 1), 1996, pp. 273-277. PMID 8694774 ; PMC 1217473 (free full text, PDF).
- ↑ Y. Oba et al. : Biosynthesis of Firefly Luciferin in Adult Lantern: Decarboxylation of L-Cysteine is a Key Step for Benzothiazole Ring Formation in Firefly Luciferin Synthesis. In: PLoS ONE. 8 (12), 2013. PMC 3877152 (free full text). PMID 24391868 .
- ↑ Y. Oba et al .: Firefly luciferase is a bifunctional enzyme: ATP-dependent monooxygenase and a long chain fatty acyl-CoA synthetase. In: FEBS Lett. 540 (1-3), 2003, pp. 251-254. PMID 12681517 ; doi: 10.1016 / S0014-5793 (03) 00272-2 .
- ↑ Oliver C. Watkins et al. : New Zealand glowworm (Arachnocampa luminosa) bioluminescence is produced by a firefly-like luciferase but an entirely new luciferin . In: Scientific Reports . tape 8 , no. 1 , February 19, 2018, p. 3278 , doi : 10.1038 / s41598-018-21298-w , PMID 29459729 .
- ^ A b S. M. Marques, JC da Silva Esteves: Firefly bioluminescence: a mechanistic approach of luciferase catalyzed reactions. In: IUBMB Life. 61 (1), 2009, pp. 6-17. PMID 18949818 ; doi: 10.1002 / iub.134 .
- ↑ a b c Osamu Shimomura, p. 249ff.
- ↑ D. Morse et al. : Role of a luciferin-binding protein in the circadian bioluminescent reaction of Gonyaulax polyedra. In: J Biol Chem . 264 (20), 1989, pp. 11822-11826. PMID 2745419 ; PDF (free full text access)
- ↑ D. Morse, M. Mittag: Dinoflagellate luciferin-binding protein. In: Methods Enzymol. 305, 2000, pp. 258-276. PMID 10812606 .
- ↑ M. Fogel, JW Hastings: A substrate-binding protein in the Gonyaulax bioluminescence reaction. In: Arch Biochem Biophys . 142 (1), 1971, pp. 310-321. PMID 5545485 .
- ↑ LW Schultz et al. : Crystal structure of a pH-regulated luciferase catalyzing the bioluminescent oxidation of an open tetrapyrrole. In: Proc Natl Acad Sci USA. 102 (5), 2005, pp. 1378-1383. PMID 15665092 ; PDF (free full text access).
- ^ M. Fogel, JW Hastings: Bioluminescence: mechanism and mode of control of scintillon activity. In: Proc Natl Acad Sci USA. 69 (3), 1972, pp. 690-369. PMID 4501583 ; PMC 426536 (free full text, PDF).
- ^ A b T. Wilson, JW Hastings: Bioluminescence. In: Annu. Rev. Cell Dev. 14, 1998, pp. 197-230. PMID 9891783 .
- ↑ C. Wu et al .: Tracer studies on dinoflagellate luciferin with [ 15 N] -glycine and [ 15 N] -l-glutamic acid in the dinoflagellate Pyrocystis lunula. In: Tetrahedron Letters. 44 (6), 2003, pp. 1263-1266; doi: 10.1016 / S0040-4039 (02) 02815-0 .
- ^ LF Greer, AA Szalay: Imaging of light emission from the expression of luciferases in living cells and organisms: a review. In: Luminescence. 17, 2002, pp. 43-74. PMID 11816060 ; doi: 10.1002 / bio.676 .
- ↑ a b c d e f Osamu Shimomura, p. 30ff.
- ↑ a b S. C. Tu: Activity coupling and complex formation between bacterial luciferase and flavin reductases. In: Photochem Photobiol Sci. 7 (2), 2008, 2008, pp. 183-188. PMID 18264585 ; doi: 10.1039 / b713462b
- ^ AJ Fisher et al .: The 1.5-A resolution crystal structure of bacterial luciferase in low salt conditions. In: J Biol Chem. 271 (36), 1996, pp. 21956-21968. PMID 8703001 ; PDF (free full text access)
- ↑ W. Duane, JW Hastings: Flavin mononucleotide reductase of luminous bacteria. In: Mol Cell Biochem. 6 (1), 1975, pp. 53-64. PMID 47604 .
- ↑ A. Rodriguez et al .: Purification of the acyl coenzyme A reductase component from a complex responsible for the reduction of fatty acids in bioluminescent bacteria. Properties and acyl transferase activity. In: J Biol Chem. 258 (8), 1983, pp. 5233-5237. PMID 6833298 ; PDF (free full text access)
- ↑ John Lee, Franz Müller, Antonie JWG Visser: The Sensitized Bioluminescence Mechanism of Bacterial Luciferase . In: Photochemistry and Photobiology . November 28, 2018, ISSN 1751-1097 , doi : 10.1111 / php.13063 , PMID 30485901 .
- ^ O. Shimomura et al .: Widespread occurrence of coelenterazine in marine bioluminescence. In: Comp. Biochem. Physiol. 65B, 1980, pp. 435-437. doi: 10.1016 / 0305-0491 (80) 90044-9
- ^ AK Campbell, PJ Herring: Imidazolopyrazine bioluminescence in copepods and other marine organisms. In: Mar. Biol. 104 (2), 1990, pp. 219-225; doi: 10.1007 / BF01313261 .
- ^ O. Shimomura: Presence of coelenterazine in non-bioluminescent marine organisms. In: Comp Biochem Physiol. 86B, 1987, pp. 361-363. doi: 10.1016 / 0305-0491 (87) 90306-3
- ↑ O. Shimomura et al .: Extraction, purification and properties of aequorin, a bioluminescent protein from the luminous hydromedusan, Aequorea. In: J Cell Comp Physiol . 59, 1963, pp. 223-239. PMID 13911999 .
- ^ O. Shimomura et al .: Mechanism of the luminescent intramolecular reaction of aequorin. In: Biochemistry . 13 (16), 1974, pp. 3278-3286. PMID 4152180 .
- ↑ JF Head et al .: The crystal structure of the photoprotein aequorin at 2.3 Å resolution. In: Nature , 405 (6784), 2000, pp. 291-293. PMID 10830969 ; PDF (free full text access)
- ↑ O. Shimomura, FH Johnson: Calcium binding, quantum yield, and emitting molecule in aequorin bioluminescence. In: Nature. 227 (5265), 1970, pp. 1356-1357. PMID 4393938 .
- ↑ S. Watase: The luminous organ of firefly squid. In: Dobutsugaku Zasshi. 17, 1905, pp. 119-123. (jap.)
- ↑ S. Inoue et al .: Squid bioluminescence III. Isolation and structure of Watasenia luciferin. In: Tetrahedron Lett. 17 (34), 1976, pp. 2971-2972. doi: 10.1016 / S0040-4039 (01) 85503-9
- ↑ a b Osamu Shimomura, p. 200ff.
- ↑ K. Teranishi, O. Shimomura: Bioluminescence of the arm light organs of the luminous squid Watasenia scintillans. In: Biochim Biophys Acta. 1780 (5), 2008, pp. 784-792. PMID 18294462 ; doi: 10.1016 / j.bbagen.2008.01.016
- ^ FI Tsuji: Role of molecular oxygen in the bioluminescence of the firefly squid, Watasenia scintillans. In: Biochem Biophys Res Commun. 338 (1), 2005, pp. 250-253. PMID 16165097 .
- ↑ a b J. G. Morin: Based on a review of the data, use of the term 'cypridinid' solves the Cypridina / Vargula dilemma for naming the constituents of the luminescent system of ostracods in the family Cypridinidae. In: Luminescence. 26 (1), 2011, pp. 1-4. PMID 19862683 ; doi: 10.1002 / bio.1178
- ^ FH Johnson, O. Shimomura: Enzymatic and nonenzymatic bioluminescence. In: Photophysiology. (7), 1972, pp. 275-334. PMID 4376836 .
- ↑ S. Kato et al .: Identification of the biosynthetic units of Cypridina luciferin in Cypridina (Vargula) hilgendorfii by LC / ESI-TOF-MS. In: Tetrahedron. 60 (50), 2004, pp. 11427-11434; doi: 10.1016 / j.tet.2004.09.080 .
- ^ S. Kato et al .: Stereoselective incorporation of isoleucine into Cypridina luciferin in Cypridina hilgendorfii (Vargula hilgendorfii). In: Bioscience, Biotechnology, and Biochemistry . 70 (6), 2006, pp. 1528-1532. PMID 16794342 ; doi: 10.1271 / bbb.60066 ; PDF (free full text access).
- ↑ FI Tsuji, GB Leisman: K / Na-triggered bioluminescence in the oceanic squid Symplectoteuthis oualaniensis. In: Proc Natl Acad Sci USA. 78 (11), 1981, pp. 6719-6723. PMID 16593119 ; PMC 349121 (free full text, PDF).
- ↑ Konstantin V. Purtov et al .: The Chemical Basis of Fungal Bioluminescence. In: Angew Chem Int Ed Engl. 54 (28), 2015, pp. 8124-8128. PMID 26094784 ; doi: 10.1002 / anie.201501779
- ↑ a b Alexey A. Kotlobay et al .: Genetically encodable bioluminescent system from fungi . In: Proceedings of the National Academy of Sciences . tape 115 , no. 50 , December 11, 2018, p. 12728-12732 , doi : 10.1073 / pnas.1803615115 , PMID 30478037 .
- ↑ External identifiers or database links for Latia-Luciferin : CAS number: 21730-91-6, PubChem : 5280505 , ChemSpider : 4444143 , Wikidata : Q27102292 .
- ↑ a b O. Shimomura, FH Johnson: The structure of Latia luciferin. In: Biochemistry. 7 (5), 1968, pp. 1734-1738. PMID 5650377 .
- ↑ a b O. Shimomura et al .: Reactions Involved in Bioluminescence Systems of Limpet ( Latia neritoides ) and Luminous Bacteria. In: Proc Natl Acad Sci USA. 69 (8), 1972, pp. 2086-2089. PMID 4506078 ; PMC 426874 (free full text, PDF).
- ↑ a b c d Osamu Shimomura, p. 182ff.
- ↑ a b c d Y. Ohmiya et al .: Bioluminescence in the Limpet-Like Snail, Latia neritoides. In: Bull. Chem. Soc. Jpn. 78 (7), 2005, pp. 1197-1205; doi: 10.1246 / bcsj.78.1197 .
- ↑ M. Nakamura et al .: Synthesis of Latia luciferin benzoate analogues and their bioluminescent activity. In: Tetrahedron Letters. 45 (10), 2004, pp. 2203-2205, doi: 10.1016 / j.tetlet.2004.01.027 .
- ^ Vadim R. Viviani: The Biological and Biochemical Diversity of Terrestrial Bioluminescence. accessed on May 2, 2015.
- ↑ a b Osamu Shimomura, p. 238ff.
- ^ NG Rudie et al .: Purification and properties of luciferin from the bioluminescent earthworm, Diplocardia longa. In: Photochem Photobiol. 23 (1), 1976, pp. 71-75. PMID 1265130 .
- ↑ NG Rudie et al .: Earthworm bioluminescence: characterization of high specific activity Diplocardia longa. luciferase and the reaction it catalyzes. In: Biochemistry. 20 (2), 1981, pp. 344-350. PMID 6258637 .
- ↑ a b M. A. Dubinnyi et al .: Novel mechanism of bioluminescence: oxidative decarboxylation of a moiety adjacent to the light emitter of Fridericia luciferin. In: Angew Chem Int Ed Engl. 54 (24), 2015, pp. 7065-7067. PMID 25913753 ; doi: 10.1002 / anie.201501668
- ↑ VN Petushkov et al .: A novel type of luciferin from the Siberian luminous earthworm Fridericia heliota: structure elucidation by spectral studies and total synthesis. In: Angew Chem Int Ed Engl. 53 (22), 2014, pp. 5566-5568. PMID 24737705 ; doi: 10.1002 / anie.201400529
- ^ HA Neufeld et al .: A rapid method for determining ATP by the firefly luciferin-luciferase system. In: Experientia , 31 (3), 1975, pp. 391-392. PMID 1116561 .
- ↑ J.-M. Hawronskyj, J. Holah: ATP: a universal hygiene monitor. In: Trends Food Sci. Tech. 8, 1997, pp. 79-84; doi: 10.1016 / S0924-2244 (97) 01009-1 .
- ↑ MR Knight et al .: Recombinant aequorin as a probe for cytosolic free Ca2 + in Escherichia coli. In: FEBS Lett. 282 (2), 1991, pp. 405-408. PMID 2037058 ; doi: 10.1016 / 0014-5793 (91) 80524-7 .
- ↑ JM Kendall et al .: Targeting aequorin to the endoplasmic reticulum of living cells. In: Biochem. Biophys. Res. Commun. 189 (2), 1992, pp. 1008-1016. PMID 1472014 .
- ↑ MR Knight et al .: Transgenic plant aequorin reports the effects of touch and cold-shock and elicitors on cytoplasmic calcium. In: Nature . 352 (6335), 1991, pp. 524-526. PMID 1865907 .
- ↑ M. Hampf, M. Gossen: A protocol for combined Photinus and Renilla luciferase quantification compatible with protein assays. In: Anal Biochem . 356 (1), Sep 1, 2006, pp. 94-99. PMID 16750160 .
- ↑ pjk-gmbh.de: Renilla Assays .
- ↑ promega.de: Dual Luciferase Reporter Assay System .
- ↑ E. Lim et al .: In vivo bioluminescent imaging of mammary tumors using IVIS spectrum. In: J Vis Exp. 26. pii, 2009, p. 1210. PMID 19404236 ; doi: 10.3791 / 1210 ; with video .
- ↑ CE O'Connell-Rodwell et al .: In vivo analysis of heat-shock-protein-70 induction following pulsed laser irradiation in a transgenic reporter mouse. In: J Biomed Opt. 13 (3), 2008, p. 030501. PMID 18601518 ; PDF (free full text access).