G protein coupled receptors
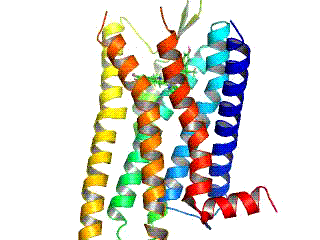
G-protein coupled receptors ( English G protein-coupled receptor , GPCR ) are biological receptors in the cell membrane and the membrane of endosomes , the signals via GTP binding proteins (abbreviated G-proteins ) or forward into the cell inside the endosome ( Signal transduction ). In neurobiology , the term metabotropic receptors is often used for G-protein-coupled receptors in order to distinguish them from another type of receptor, the ligand-gated ion channels (ionotropic receptor).
The family of G-protein-coupled receptors is the largest protein superfamily with more than 1000 different members. G-protein-coupled receptors are responsible for processing light , smell and a variety of taste stimuli . They play a crucial role in inflammatory processes , targeted cell movement ( taxis ), the transport of substances through the cell membrane ( endocytosis and exocytosis ) as well as cell growth and cell differentiation . As target structures, they are also responsible for the action of hormones such as adrenaline or glucagon , and neurotransmitters such as serotonin and acetylcholine . Some viruses also use G-protein-coupled receptors as binding sites for entry into the cell (e.g. HIV ).
definition
All heptahelical receptors, that is, receptors anchored with 7 helices in the cell membrane, which are capable of binding and activating G proteins, are referred to as G protein-coupled receptors in the narrower sense. In addition to binding G-proteins, many of these receptors are also able to interact with other signal-carrying proteins . As in the case of many so-called "orphan GPCRs", receptor proteins for which a coupling with G proteins is only suspected are referred to as G protein-coupled receptors. Sometimes this term is also applied to receptors that are not able to bind and activate G proteins, but can be assigned to this family due to their phylogenetic relationship with other G protein-coupled receptors that meet the classic definition. In contrast, prokaryotic rhodopsins , which are similar in structure to eukaryotic G protein-coupled receptors, but are not capable of binding G proteins and function as ion channels , are not assigned to the family of G protein-coupled receptors.
Occurrence
G protein-coupled receptors are found in almost all living things. They are found particularly numerous in vertebrates . But even invertebrates , protozoa (eg. As amoeba ) and fungi (for example in yeast ) have G protein-coupled receptors. Some plant cell membrane proteins can also be assigned to the G-protein-coupled receptors because of their ability to activate heterotrimeric G-proteins. In particular, they play a role as phytohormone receptors. However, not all of these plant receptors, such as GCR1 from Acker-Schmalwand, are classic heptahelical G-protein-coupled receptors, but like GCR2, which is more similar to bacterial lanthionine synthases, may have different structural motifs . Some photoreceptors with a structure that resembles G-protein-coupled receptors can even be found in archaea ( bacteriorhodopsins ). However, these receptors, which function as ion channels, are not related to photoreceptors in higher animals and are usually not included in the family of G-protein-coupled receptors.
When people 800 G protein-coupled receptors have been about to be identified. These are encoded by around 3% of the human genome . More than half of the G-protein-coupled receptors in humans are assigned to the olfactory receptors (olfactory receptors). The endogenous ligand of over 140 of the approx. 800 G protein-coupled receptors is not known and they are therefore referred to as orphan GPCRs .
structure
Due to their structure, with the exception of the plant GCR2, all G-protein-coupled receptors belong to the superfamily of heptahelical transmembrane proteins (common synonyms: seven transmembrane domain receptors, 7-TM receptors and heptahelical receptors). They consist of subunits with seven (Greek “ hepta ”) helical structures spanning the cell membrane (transmembrane) , which are connected by three intracellular and three extracellular loops. G-protein-coupled receptors have an extracellular or transmembrane binding domain for a ligand . The G-protein binds to the cell inner (intracellular) side of the receptor. The Ballesteros-Weinstein system of nomenclature was developed to compare the structure of the various G-protein-coupled receptors with one another .
For a long time the structure of G-protein-coupled receptors could only be predicted by analogy with the known structure of bacteriorhodopsin. The three-dimensional structure elucidation of a G-protein-coupled receptor in vertebrates , the rhodopsin of domestic cattle , was achieved in 2000 with the help of X-ray structure analysis . The crystallization and structure elucidation of other G protein-coupled receptors, on the other hand, is more difficult because of their physicochemical properties and because of the low density of receptors in the membrane. Therefore, it was not until 2007 that the crystal structure of a ligand-activated G protein-coupled receptor ( human β 2 -adrenoceptor ) could be elucidated using technical tricks such as the use of stabilizing antibodies or the fusion with easily crystallizable proteins.
In the meantime, the three-dimensional structure, including that of the transmembrane domains, has been established for numerous G-protein-coupled receptors of the physiologically and therapeutically important class A. The structure was mainly determined with the help of X-ray crystal analysis using fusion proteins or methods for thermal stabilization. In this way, among other things, the structure of the β 1 -adrenoceptor , the A 2A - adenosine receptor , the D 3 - dopamine receptor , the opioid receptors κ, μ, δ, nociceptin, the S1P 1 receptor, the muscarinic acetylcholine receptors M 2 and M 3 , the histamine H 1 receptor , the serotonin receptors 5-HT 1B and 5-HT 2B , the chemokine receptors CCR5 and CXCR4 , the neurotensin receptor NTS 1 and the protease-activated receptor PAR1. The three-dimensional structure of the natural human chemokine receptor CXCR1 was determined using nuclear magnetic resonance spectroscopy . Liquid and solid phase spectroscopy are used.
For class A G protein-coupled receptors, structural data are available for the receptors in complex with signal transduction molecules, such as heterotrimeric G proteins and arrestin, and for various activation states. These data provide important insight into the molecular mechanism of receptor activation and signal transmission.
The structural data for the corticotropin-releasing hormone receptor 1 and the glucagon receptor are available for the G-protein-coupled class B receptors . The so far only structurally elucidated G protein receptor of class F, the smoothened receptor , differs from the rhodopsin-like G protein-coupled receptors of class A , due to the lack of many typical structural motifs and pronounced extracellular loops. For numerous other G protein-coupled receptors, at least parts of the structure, such as that of the extracellular domains, could be determined experimentally.
The special role of the plant phytohormone receptor GCR2 among the G protein-coupled receptors could also be confirmed with the help of the X-ray structure analysis. Although it was assigned to the group of G protein-coupled receptors on the basis of biochemical predictions and activation by the phytohormone abscisic acid , it has significantly different physicochemical properties. With its identified 14 helices, it is the only known non-heptahelical G-protein-coupled receptor and is structurally more similar to bacterial lanthionine synthases.
Transmembrane domains
The seven membrane-spanning helical domains of G-protein-coupled receptors, which are arranged counterclockwise when looking at the receptor from the extracellular side, are responsible for anchoring the receptor in the cell membrane. In particular the transmembrane domains III-VI harbor binding sites for a ligand. The transmembrane domains I, II and IV may play a role in the di- and oligomerization of G protein-coupled receptors.
In contrast to the extracellular and intracellular domains , the transmembrane domains are highly conserved within the family of G-protein-coupled receptors. Some amino acid sequences (motifs) within the transmembrane domains are characteristic of many G-protein-coupled receptors. For example, the E / DRY motif of transmembrane domain III and the NPxxY motif of transmembrane domain VII can be found in almost all rhodopsin-like receptors. They are assigned an important role in receptor activation.
Extracellular Domains
Some G-protein coupled receptors, such as B. the metabotropic glutamate receptors have their primary ligand binding site in their extracellular N-terminal domain. These receptors are characterized by long N-terminal amino acid sequences (up to 2800 amino acids), while receptors with intracellular ligand binding domains usually only have short residues (mostly below 30 amino acids). The N-terminus and the extracellular domains of the receptor are often glycosylated .
Large structural differences could also be found in the second extracellular loop (EL 2), which is located near the ligand binding site of the rhodopsin-like G protein-coupled loop. Often either β-hairpin or α-helix motifs can be found in this loop. In the second extracellular loop and at the beginning of the third transmembrane domain of the receptor there are two conserved cysteines capable of forming disulfide bridges , which stabilize the structure of the receptor by linking the transmembrane domains III to V to one another. In contrast to this, the extracellular loop 2 of the κ-opioid receptor, for example, is only stabilized via a disulfide bridge.
Intracellular Domains
The intracellular side of the receptor is equipped with binding sites for G-proteins and other signaling molecules. The amino acids close to the transmembrane domain of the second (IL 2) and third intracellular loop (IL 3) and the C-terminal residue adjoining the 7th transmembrane domain are involved in the binding of G proteins. The intracellular C-terminal part is usually very short (usually less than 50 amino acids). Some G protein-coupled receptors, such as the gonadotropin-releasing hormone receptor , lack this part. An eighth helix (Hx 8) beginning with a conserved cysteine , which runs parallel to the cell membrane, can also connect directly to the intracellular end of the 7th transmembrane domain . The helix 8 often carries fatty acid residues such as palmitic acid and oleic acid residues, which serve to anchor this helix in the cell membrane.
function
The main function of the G-protein-coupled receptors is to transmit signals inside the cell. This signal transmission (signal transduction) takes place in particular via the activation of G proteins. Some G-protein-coupled receptors are also capable of G-protein-independent signal transduction, for example via arrestins .
Activation of G proteins
Almost all G protein-coupled receptors are capable of direct activation of a (heterotrimeric) G protein consisting of three subunits (α, β and γ). The activation of a G protein is a multi-step process that includes the binding of a ligand to the receptor, the conformational change of the receptor, and the binding and activation of a G protein, and is subject to the laws of thermodynamics .
Step 1: binding the G protein
Activated receptors interact with heterotrimeric G proteins, which are thereby activated themselves. The receptor shows a selectivity for one (for example β 1 -adrenoceptor : G s ) or for several different (for example β 2 -adrenoceptor: G s and G i / o ) G protein subtypes. This complex of receptor and heterotrimeric G protein is part of a larger network on which other signal-transmitting proteins, such as. B. GIRK channels , phospholipase C and protein kinase C are involved. With the help of this network, a fast activation of the signal transduction cascade of G-protein-coupled receptors, often lasting only milliseconds to a few seconds, can be explained. Whether the receptor interacts with the G protein through collision coupling, or whether receptors are also associated with the G protein in the inactive state, is the subject of current research.
Step 2: ligand binding
Depending on the type of ligand , the binding to the receptor takes place at its extracellular, transmembrane or intracellular domains:
- The covalently bound ligand 11-cis-retinal , which functions as a prosthetic group , is an integral part of the light receptor rhodopsin (Fig. A) on its transmembrane domains.
- Amines (e.g. acetylcholine , adrenaline , histamine and serotonin ), nucleotides (e.g. ATP ), eicosanoids (e.g. prostacyclin ) and some lipids (e.g. ceramides ) bind to transmembrane binding sites of their receptors (Fig a).
- Neuropeptides (e.g. oxytocin and vasopressin ) occupy several transmembrane and extracellular binding sites of their receptors at the same time (Fig. B).
- Proteinases (e.g. thrombin and trypsin ) specifically cleave a short N-terminal fragment of their receptors, the protease-activated receptors . As a result of the proteolytic cleavage, an intrinsic ligand (“ tethered ligand ”) is exposed at the newly formed receptor N-terminus, which in a second step attaches to a transmembrane binding site (Fig. C).
- Proteohormones (e.g. glucagon ) bind primarily to extracellular domains of the receptor. After a change in the conformation of the receptor, the peptide hormone binds secondary to transmembrane binding sites (Fig. D).
- Some small substances (e.g. glutamate and calcium ions ) only bind to extracellular receptor domains. The attachment of the ligand changes the conformation of the extracellular domains so that they come into contact with intracellular domains (Fig. E).
Step 3: activation of the receptor
If a ligand binds to a receptor and this is activated by the ligand ( agonist ), this attachment usually leads to the salt bridge between the 3rd and 7th transmembrane domains of the G-protein-coupled receptor being broken. This activated receptor gains more flexibility and changes its three-dimensional structure. By changing the conformation of the receptor, it can now function as a GTP exchange factor (GEF) for the bound G protein.
Many G protein-coupled receptors are in a balance between inactive and activated state even in the absence of an agonist. Attachment of an agonist shifts the equilibrium towards the active state, while inverse agonists shift the equilibrium towards the inactive state. Receptors, which can be located even in the absence of an agonist in an activated state is called constitutively active (English: c onstitutively a ctive , abbreviated ca ). Mutations in the receptor can also cause constitutive activity (“ constitutive active mutants ”, CAM). Such mutated G-protein-coupled receptors are associated with certain diseases (e.g. certain forms of retinopathia pigmentosa due to constitutively active rhodopsin) and occur in certain herpes viruses . Furthermore, in experimental pharmacology, G-protein-coupled receptors are now also being changed specifically to CAMs in order to use them in the search for new drugs.
Step 4: activation of the G proteins
Heterotrimeric G proteins can bind GTP and GDP, the GDP-binding form is inactive. The activation of the receptor ensures the exchange of GDP for GTP on the α subunit of the G protein. The G-protein complex becomes unstable when the GTP binds. As a result, the conformation of the heterotrimeric G protein changes and it can dissociate into the α and βγ subunits. The activated G proteins can now transmit the exogenous signals transmitted by the ligand into the cell interior (signal transduction).
Step 5: signal transduction
The activated subunits of the G protein are responsible for further signal transduction . Depending on the subunit, other cell or membrane-bound proteins are activated or deactivated. For example, the α-subunits of G s / olf modulate the activity of adenylyl cyclase , while α-subunits of G q / 11 proteins activate phospholipase C. These enzymes are then involved in the formation of a secondary messenger substance .
Step 6: G protein inactivation
The intrinsic GTPase activity of the α-subunit of the G protein cleaves the bound GTP into GDP + P i after a time and with the help of proteins that increase the GTPase activity ( GTPase activating protein , GAP) . The α-subunit of the G-protein can thus reassociate with the βγ-subunit and again bind to a receptor (see step 1). So there is self-regulation.
Alternative signal transduction pathways
Many G protein-coupled receptors are not only capable of activating G proteins, but also of binding and activating other molecules that control cell processes. In this way, G-protein-coupled receptors can control alternative signal transduction pathways independently of G-protein. Examples of this are the Frizzled receptors, which can activate β-catenin via the Wnt signaling pathway , the β 2 receptor, which can modulate the function of the Na + / H + exchanger independently of the G protein , and the 5-HT 2B Receptor whose eNOS activating function is at least partially also G protein-independent. The function of G-protein-coupled receptors can also be regulated by G-protein-independent activation of arrestin and Homer proteins .
Regulation of the receptor function
For the function of G-protein-coupled receptors, their localization on the cell surface is a prerequisite. Cellular processes that lead to an increase in the number of receptors on the cell membrane and thus the receptor function are called up-regulation . In contrast, the removal of functional receptors from the cell membrane ( down-regulation ) represents a mechanism for terminating the receptor function.
Up-regulation
The up-regulation of G-protein-coupled receptors is a multi-step process. The starting point is the protein biosynthesis of the receptor on the endoplasmic reticulum , which can be regulated indirectly, among other things, by G-protein-coupled receptors.
The transport of the receptor synthesized in this way via Golgi vesicles to the cell membrane can be controlled by chaperones and chaperone-like proteins, including proteins that modify receptor activity . Regulation of membrane transport is also possible by glycosylation of the receptor. The importance of the di- and oligomerization observed for many G-protein-coupled receptors as a prerequisite for their transport to the cell membrane and thus for their functionality is also discussed. The incorporation of G protein-coupled receptors into the cell membrane can be controlled, for example, by Homer proteins, PSD-95 and spinophilin .
Down regulation
The effects mediated by G-protein-coupled receptors decrease after a longer period of activation. The phosphorylation of intracellular domains of the receptor (C-terminal serine or threonine residues) by protein kinases plays a key role in this down -regulation .
Phosphorylation by second messenger activated kinases
Protein kinases that are activated via G-protein-mediated production of second messengers (secondary messenger substances) (such as protein kinase A activated by cyclic adenosine monophosphate (cAMP) or protein kinase C activated by diacylglycerol and calcium ) can contain many G-protein-coupled Phosphorylate receptors. This often interrupts signal transduction via the receptor, since the phosphorylated receptor has a lower affinity for G proteins. This regulation can therefore act as a negative feedback mechanism.
Phosphorylation by G protein-coupled receptor kinases
G-protein-coupled receptor kinases ( GRKs for short ) essentially have two functions. First, they can interact with the Gα and Gβγ subunits of the heterotrimeric G proteins, which means that they no longer contribute to signal transduction. They can also act as GAP (GTPase-activating protein) and accelerate the conversion of GTP * Gα to GDP * Gα. Second, they are serine / threonine kinases and, as such, can phosphorylate G-protein-coupled receptors.
Consequences of phosphorylation
- Conformational change of the receptor: the conformation of the receptor changes due to the strongly negative charge of the phosphate residue and the associated electrostatic interaction forces. The new conformation is often less favorable for the receptor G-protein interaction or influences the affinity of the receptor, so that the receptor signal is weakened. This type of desensitization is often carried out by the second messenger-dependent protein kinases A and C.
- Interaction with beta-arrestins: By binding arrestin to the receptor, which is mainly phosphorylated by GRKs, sterically binding of the G proteins is prevented (= short-term regulation within a few minutes). In addition, the beta-arrestins serve as “scaffold” molecules for a large number of other proteins, clathrin and the MAP kinases in particular .
- Internalization: The binding of the receptor-arrestin complex to clathrin leads to the removal of the phosphorylated receptor from the cell surface into the interior of the cell in the form of membrane vesicles (clathrin-coated pits). The receptor can then be broken down intracellularly, recycled and thus brought back to the surface, or it can also persist as an intracellular receptor. This regulation usually takes place within 10 to 30 minutes and is often reversed within 30 to 60 minutes after the receptor stimulus has been removed. Long-term regulation over days or months is often due to transcription regulation.
The binding of MAP kinases to the receptor-arrestin complex means that these are no longer stimulated via G proteins, but directly by the receptor. This change in the signal transduction mechanism also takes place within about ten minutes.
Classification
Classification by function
A first systematic classification of the G-protein-coupled receptors took place in the early 1990s on the basis of functional characteristics and is continued to this day:
- Class A (or 1) (Rhodopsin-like ( Rhodopsin ))
- Class B (or 2) (secretin receptor family ( secretin ))
- Class C (or 3) (metabotropic glutamate / pheromone ( glutamate receptor ))
- Class D (or 4) (Fungal mating pheromone receptors)
- Class E (or 5) (cyclic AMP receptors ( cAMP ))
- Class F (or 6) (Frizzled / Smoothened ( Wnt signal path ))
Using this system, the G-protein-coupled receptors of vertebrates and invertebrates were divided into five groups (A – E). Group A represented rhodopsin- related receptors, glycoprotein receptors were divided into group B, and metabotropic glutamate receptors into group C. Group D and E receptors are not found in vertebrates. They function as pheromone receptors in yeast and as cAMP receptors in nematodes . With the discovery of new G protein-coupled receptors, this system has been expanded in recent years. Outside of the ABCDE system described above, separate groups have been established for the G-protein-coupled receptors of plants, for the olfactory receptors of insects, for the chemoreceptors of nematodes and for the "frizzeled / smoothened" receptors of higher animals.
Phylogenetic classification
An alternative system of classification of the human G protein-coupled receptors has been proposed based on phylogenetic studies (GRAFS or Fredriksson system). According to this system, G-protein-coupled receptors are divided into five main groups: the glutamate, rhodopsin, adhesion, frizzled / Taste2 and secretin groups.
The importance of G-protein-coupled receptors for medicine
Medicinal substances
In modern medicine , G-protein-coupled receptors occupy a key position: About 30% of all prescription drugs currently on the market act on G-protein-coupled receptors. These drugs include the commonly prescribed beta blockers , neuroleptics , antihistamines , opioids and sympathomimetics . New drugs that act via G-protein-coupled receptors, such as the triptans , setrons and sartans , have also achieved a high level of importance in recent years.
Drug group | Examples | indication | Receptor (s) | Explanations |
---|---|---|---|---|
α 2 agonists | Clonidine | Arterial hypertension | α 2 adrenoceptors | As agonists at α 2 -adrenoceptors in the central nervous system, they lower the activity of the sympathetic nervous system and thereby reduce blood pressure. |
Alpha blockers | Prazosin , tamsulosin | Arterial hypertension , prostatic hyperplasia | α 1 adrenoceptors | As antagonists of α 1 -adrenoceptors, they lower the tone of the smooth muscles in blood vessels and in the urogenital tract . |
Anticholinergics | Atropine , ipratropium , tiotropium | Urinary incontinence , bronchial asthma , bradycardiac arrhythmias | Muscarinic acetylcholine receptors | As antagonists of muscarinic receptors, they lower the tone of the smooth muscles in the bronchi and urogenital tract. Inhibit the heart rate- lowering effects of acetylcholine . |
H 1 antihistamines | Diphenhydramine , Loratadine , Cetirizine | Allergic reaction | H 1 receptors | As antagonists, inhibit the effect of histamine , which is released in allergic reactions, on H 1 receptors. |
H 2 antihistamines | Ranitidine , famotidine , cimetidine | Control of gastric acid production ( reflux diseases , gastric ulcer ) | H 2 receptors | As antagonists at H 2 receptors, inhibit the histamine-mediated release of gastric acid . |
AT 1 antagonists | Losartan , candesartan , irbesartan , valsartan , telmisartan | Hypertension, heart failure, coronary artery disease | AT 1 receptors | As angiotensin II antagonists at AT 1 receptors, they lower the tone of the smooth muscles in blood vessels. |
Beta blockers | Atenolol , bisoprolol , carvedilol , metoprolol , timolol | Arterial hypertension , heart failure , coronary artery disease , migraines , glaucoma | β 1 adrenoceptors | As antagonists of β 1 -adrenoceptors, lower heart rate among other things. Lowering intraocular pressure. |
Bradykinin receptor antagonists | Icatibant | Hereditary angioedema | Bradykinin receptors | As antagonists, block the effects of bradykinin, which mediate inflammatory symptoms. |
Dopamine agonists | Pergolide , cabergoline , pramipexole , ropinirole | Parkinson's disease , restless legs syndrome | Dopamine receptors | As agonists at dopamine receptors, they mimic the effects of dopamine. |
Endothelin receptor antagonists | Bosentan , sitaxentan , ambrisentan | Pulmonary hypertension | Endothelin receptors | As antagonists at ET 1 receptors, they lower the tone and remodeling of the pulmonary blood vessels. |
Coreceptor antagonists | Maraviroc | HIV | Chemokine receptors CCR5 or CXCR4 | Block the chemokine receptors necessary for the entry of HI viruses. |
NK1 receptor antagonists | Aprepitant | Vomit | NK1 receptors | As antagonists, inhibit NK1 receptors in the vomiting center. |
Neuroleptics | Haloperidol , risperidone , clozapine , olanzapine | schizophrenia | D 2 receptors , 5-HT 2A receptors | Lead to an antipsychotic effect via a priority inhibition of D 2 receptors (typical neuroleptics) or 5-HT 2A receptors (atypical neuroleptics). |
Opioids | Morphine , codeine , fentanyl , loperamide | Pain , anesthesia , cough , diarrhea | Opioid receptors | As agonists at the opioid receptors μ, κ and δ lead to spinal and supraspinal analgesia and central inhibition of the coughing stimulus. |
S1P receptor modulators | Fingolimod | multiple sclerosis | Sphingosine-1-phosphate receptors | Internalization of S1P receptors. |
Triptans | Sumatriptan , naratriptan , zolmitriptan | migraine | 5-HT 1B receptors | Migraine therapeutic effect via stimulation of 5-HT 1B receptors in cerebral blood vessels and neurons . |
research
The principle of signal transmission by hormones by means of receptors and secondary messenger substances was postulated for the first time in 1960, the transmission by G proteins was developed in the 1970s to 1980s. For this work, in 1971 Earl W. Sutherland Jr. "for his discoveries about the mechanisms of action of hormones " and in 1994 Alfred G. Gilman and Martin Rodbell "for the discovery of cell communication and in particular the discovery of G proteins " were awarded the Nobel Prize for Physiology or medicine honored. In 2012, Brian Kobilka and Robert Lefkowitz were honored with the Nobel Prize in Chemistry for their work on G-protein-coupled receptors .
G protein-coupled receptors are still among the most intensely studied targets for the development of new drugs in the drug industry . In particular, new receptors that have been discovered within the last 20 years, such as cannabinoid receptors , CGRP receptors, chemokine receptors, endothelin receptors, leptin receptors, neurokinin receptors and neuropeptide Y receptors, are of particular interest .
Receptor oligomers
literature
- TH Ji, M. Grossmann, I. Ji: G protein-coupled receptors. I. Diversity of receptor-ligand interactions. In: J. Biol. Chem. , 273, 1998, pp. 17299-17302.
- U. Gether, BK Kobilka: G protein-coupled receptors. II. Mechanism of agonist activation. In: J. Biol. Chem. , 273, 1998, pp. 17979-17982.
- RJ Lefkowitz: G protein-coupled receptors. III. New roles for receptor kinases and beta-arrestins in receptor signaling and desensitization. In: J. Biol. Chem. , 273, 1998, pp. 18677-18680.
- KL Pierce, RT Premont, RJ Lefkowitz: Seven-transmembrane receptors. In: Nat Rev Mol Cell Biol . 9, 2002, pp. 639-50
- RJ Lefkowitz, EJ Whalen: Beta-arrestins: traffic cops of cell signaling. In: Curr. Opin. Cell Biol. , 16, 2004, pp. 162-168.
- RJ Lefkowitz, SK Shenoy: Transduction of receptor signals by beta-arrestins. In: Science 308, 2005, pp. 512-517.
- R. Fredriksson, HB Schiöth: The Repertoire of G-Protein Coupled Receptors in Fully Sequenced Genomes. In: Mol Pharmacol . , 67, 2005, pp. 1414-1425.
- SJ Hill: G-protein-coupled receptors: past, present and future. In: Br J Pharmacol . , 147, 2006, pp. S27-S37.
- Harvey Lodish, Arnold Berk, S. Lawrence Zipursky , Paul Matsudaira, David Baltimore: Molecular Cell Biology. 4th edition, p. 922 ff.
- Alberts et al .: Molecular Biology of the Cell. 4th ed., Pp. 852 ff.
Web links
- Classification of the G-protein-coupled receptors (English)
- IUPHAR Receptor Database - detailed list of GPCR-like receptors of the International Union of Pharmacology (English)
- Modern Drug Discovery - It's a GPCR world . (English)
- GPCRDB Tools - Visualization and Analysis of GPCR Data (English)
Individual evidence
- ↑ R. Irannejad, JC Tomshine u. a .: Conformational biosensors reveal GPCR signaling from endosomes. In: Nature. Volume 495, number 7442, March 2013, pp. 534-538, doi: 10.1038 / nature12000 , PMID 23515162 .
- ^ S. Pandey, SM Assmann: The Arabidopsis putative G protein-coupled receptor GCR1 interacts with the G protein alpha subunit GPA1 and regulates abscisic acid signaling . In: Plant Cell . 16, 2004, pp. 1616-1632.
- ↑ a b X. Liu, Y. Yue, B. Li et al .: AG protein-coupled receptor is a plasma membrane receptor for the plant hormone abscisic acid . In: Science . 315, No. 5819, March 2007, pp. 1712-1716. doi : 10.1126 / science.1135882 . PMID 17347412 .
- ↑ N. Tuteja: Signaling through G protein coupled receptors . In: Plant Signal Behav . 4, No. 10, October 2009, pp. 942-947. PMID 19826234 . PMC 2801357 (free full text).
- ↑ a b J.-H. Chen, J. Guo, J.-G. Chen, SK Nair: Crystal Structure of Arabidopsis GCR2 . 2013. doi : 10.2210 / pdb3t33 / pdb . Retrieved May 7, 2013.
- ↑ A. Levoye, J. Dam, MA Ayoub, JL Guillaume, R. Jockers: Do orphan G-protein-coupled receptors have ligand-independent functions? New insights from receptor heterodimers. In: EMBO reports. Volume 7, number 11, November 2006, pp. 1094-1098, doi: 10.1038 / sj.embor.7400838 , PMID 17077864 , PMC 1679777 (free full text).
- ↑ K. Palczewski, T. Kumasaka, T. Hori, CA Behnke, H. Motoshima, BA Fox, I. Le Trong, DC Teller, T. Okada, RE Stenkamp, M. Yamamoto, M. Miyano: Crystal structure of rhodopsin : AG protein-coupled receptor. In: Science. Volume 289, Number 5480, August 2000, pp. 739-745, PMID 10926528 .
- ↑ SG Rasmussen et al .: Crystal structure of the human β 2 adrenergic G-protein-coupled receptor . In: Nature . 450, No. 7168, 2007, pp. 383-387. PMID 17952055 .
- ↑ V. Cherezov et al .: High-resolution crystal structure of an engineered human beta2-adrenergic G protein-coupled receptor . In: Science . 318, No. 5854, 2007, pp. 1258-1265. PMID 17962520 .
- ^ T. Warne et al .: Structure of a beta (1) -adrenergic G-protein-coupled receptor. Nature . 2008 Jun 25. Epub ahead of print, PMID 18594507
- ^ V Jaakola et al .: The 2.6Å Crystal Structure of a Human A 2A Adenosine Receptor Bound to an Antagonist . In: Science , Epub 2008 Oct 2, PMID 18832607
- ↑ Protein Data Bank: 3PBL Structure Summary
- ↑ a b H. Wu et al .: Structure of the human κ-opioid receptor in complex with JDTic . In: Nature . 485, No. 7398, 2012, pp. 327-332. doi : 10.1038 / nature10939 . PMID 22437504 .
- ↑ A Manglik et al. (2012) PMID 22437502
- ↑ S. Granier et al. (2012) PMID 22596164
- ↑ AA Thompson, W. Liu, E. Chun, V. Katritch, H. Wu, E. Vardy, XP Huang, C. Trapella, R. Guerrini, G. Calo, BL Roth, V. Cherezov, RC Stevens: Structure of the nociceptin / orphanin FQ receptor in complex with a peptide mimetic. In: Nature. Volume 485, number 7398, May 2012, pp. 395-399, doi: 10.1038 / nature11085 , PMID 22596163 , PMC 3356928 (free full text).
- ↑ MA Hanson et al .: Crystal structure of a lipid G protein-coupled receptor . In: Science . 335, No. 6070, 2012, pp. 851-855. doi : 10.1126 / science.1215904 . PMID 22344443 .
- ↑ K. Haga et al .: Structure of the human M2 muscarinic acetylcholine receptor bound to an antagonist . In: Nature . 482, No. 7386, 2012, pp. 547-551. doi : 10.1038 / nature10753 . PMID 22278061 .
- ^ AC Kruse, J. Hu, AC Pan, DH Arlow, DM Rosenbaum, E. Rosemond, HF Green, T. Liu, PS Chae, RO Dror, DE Shaw, WI Weis, J. Wess, BK Kobilka: Structure and dynamics of the M3 muscarinic acetylcholine receptor. In: Nature. Volume 482, number 7386, February 2012, pp. 552-556, doi: 10.1038 / nature10867 , PMID 22358844 , PMC 3529910 (free full text).
- ↑ T. Shimamura et al .: Structure of the human histamine H1 receptor in complex with doxepin . In: Nature . 475, No. 7354, 2012, pp. 65-70. doi : 10.1038 / nature10236 . PMID 21697825 .
- ↑ C. Wang, Y. Jiang, J. Ma et al .: Structural Basis for Molecular Recognition at Serotonin Receptors . In: Science . March 2013. doi : 10.1126 / science.1232807 . PMID 23519210 .
- ↑ Q. Tan, Y. Zhu, J. Li, Z. Chen, GW Han, I. Kufareva, T. Li, L. Ma, G. Fenalti, J. Li, W. Zhang, X. Xie, H. Yang, H. Jiang, V. Cherezov, H. Liu, RC Stevens, Q. Zhao, B. Wu: Structure of the CCR5 chemokine receptor-HIV entry inhibitor maraviroc complex . In: Science . 341, No. 6152, 2013, pp. 1387-1390. doi : 10.1126 / science.1241475 . PMID 24030490 .
- ↑ B. Wu, EY Chien, CD Mol et al .: Structures of the CXCR4 Chemokine GPCR with Small-Molecule and Cyclic Peptide Antagonists . In: Science . October 2010. doi : 10.1126 / science.1194396 . PMID 20929726 .
- ^ JF White et al .: Structure of the agonist-bound neurotensin receptor . In: Nature . 490, No. 7421, 2012, pp. 508-513. doi : 10.1038 / nature11558 . PMID 23051748 .
- ↑ C. Zhang et al .: High-resolution crystal structure of human protease-activated receptor 1 . In: Nature . Epub ahead of print, 2012. doi : 10.1038 / nature11701 . PMID 23222541 .
- ↑ SH Park et al .: Structure of the chemokine receptor CXCR1 in phospholipid bilayers . In: Nature . 491, No. 7426, 2012, pp. 779-783. doi : 10.1038 / nature11580 . PMID 23086146 .
- ↑ Casey O'Connor, Kate L. White, Nathalie Doncescu, Tatiana Didenko, Bryan L. Roth, Georges Czaplicki, Raymond C. Stevens, Kurt Wüthrich, Alain Milon: NMR structure and dynamics of the agonist dynorphin peptide bound to the human kappa opioid receptor . In: Proceedings of the National Academy of Sciences . 112, No. 38, 2015, pp. 11852–11857. doi : 10.1073 / pnas.1510117112 .
- ↑ K. Hollenstein, J. Kean, A. Bortolato, RK Cheng, AS Doré, A. Jazayeri, RM Cooke, M. Weir, FH Marshall: Structure of class B GPCR corticotropin-releasing factor receptor 1 . In: Nature . 499, No. 7459, 2013, pp. 438-443. doi : 10.1038 / nature12357 . PMID 23863939 .
- ↑ FY Siu, M. He, C. de Graaf, GW Han, D. Yang, Z. Zhang, C. Zhou, Q. Xu, D. Wacker, JS Joseph, W. Liu, J. Lau, V. Cherezov , V. Katritch, MW Wang, RC Stevens: Structure of the human glucagon class B G-protein-coupled receptor . In: Nature . 499, No. 7459, 2013, pp. 444-449. doi : 10.1038 / nature12393 . PMID 23863937 .
- ^ C. Wang, H. Wu, V. Katritch et al .: Structure of the human smoothened receptor bound to an antitumour agent . In: Nature . May 2013. doi : 10.1038 / nature12167 . PMID 23636324 .
- ↑ CA Johnston, BR Temple, JG Chen et al .: Comment on “AG protein coupled receptor is a plasma membrane receptor for the plant hormone abscisic acid” . In: Science . 318, No. 5852, November 2007, p. 914; author reply 914. doi : 10.1126 / science.1143230 . PMID 17991845 .
- ↑ S. Tsunoda, J. Sierralta, Y. Sun, R. Bodner, E. Suzuki, A. Becker, M. Socolich, CS Zuker: A multivalent PDZ domain protein assembles signaling complexes in a G-protein-coupled cascade. In: Nature , 388, 1997, pp. 243-249.
- ^ M. Nobles, A. Benians, A. Tinker: Heterotrimeric G proteins precouple with G protein-coupled receptors in living cells. In: Proc. Natl. Acad. Sci. USA , 120, 2005, pp. 18706-18711.
- ↑ Jeremy M. Berg, John L. Tymoczko, Lubert Stryer : Biochemistry. 5th edition. Freeman, New York 2002, ISBN 0-7167-4684-0 , available online from the NCBI Bookshelf.
- ↑ Püschel et al .: Pocket Textbook Biochemistry . Thieme-Verlag, 2011, p. 318 ( online ).
- ↑ C. Patterson, GA Stouffer, N. Madamanchi, MS Runge: New tricks for old dogs: nonthrombotic effects of thrombin in vessel wall biology. In: Circulation research. Volume 88, Number 10, May 2001, pp. 987-997, PMID 11375267 (review).
- ^ B. Trzaskowski, D. Latek, S. Yuan, U. Ghoshdastider, A. Debinski, S. Filipek: Action of molecular switches in GPCRs – theoretical and experimental studies. In: Current medicinal chemistry. Volume 19, number 8, 2012, pp. 1090-1109, PMID 22300046 , PMC 3343417 (free full text) (review).
- ↑ CM Tan, AE Brady, HH Nickols, Q. Wang, LE Limbird: Membrane trafficking of G protein-coupled receptors. In: Annu. Rev. Pharmacol. Toxicol. , 44, 2004, pp. 559-609.
- ↑ GPCRDB: Information system for G protein-coupled receptors
- ^ R. Fredriksson, MC Lagerstrom, LG Lundin, HB Schiöth: The G-protein-coupled receptors in the human genome form five main families. Phylogenetic analysis, paralogue groups, and fingerprints. In: Mol. Pharmacol. 63, 2003, pp. 1256-1272, PMID 12761335
- ↑ Joachim Rassow, Karin Hauser, Roland Netzker, Rainer Deutzmann: Biochemistry (= dual series ). 4th edition. Thieme, Stuttgart 2016, ISBN 978-3-13-125354-5 , p. 561 .