Rare Earth Hypothesis

The Rare Earth hypothesis ( English for rare earth ') is the assumption that the complex multicellular life of the earth on an unlikely combination especially astrophysical and geological due conditions. The term Rare Earth comes from the 2000 book Rare Earth: Why Complex Life Is Uncommon in the Universe by Peter Ward , a geologist and paleontologist , and Donald Brownlee , an astronomer and astrobiologist . The title of the German edition is Our lonely earth: Why complex life in the universe is improbable .
The rare earth hypothesis is a limitation of the Copernican principle , which proponents of extraterrestrial life included Carl Sagan and Frank Drake . Accordingly, the earth is a typical rock planet in a typical planetary system in a not unusual area of a frequently occurring barred spiral galaxy . Therefore, it is likely that complex life in the universe occurs frequently. The rare earth hypothesis asserts the opposite: The combination of planets, planetary systems and galactic regions (the position of the solar system within the Milky Way system), which is so favorable for complex life as that on earth, is very rare.
If one assumes that complex life occurs very rarely, then the rare earth hypothesis provides a way of avoiding the Fermi paradox : "If extraterrestrial life forms should occur more frequently, why are they not evident ?"
Arguments for the rare earth hypothesis
overview
The rare earth hypothesis assumes that the emergence of complex life requires a number of favorable circumstances. Among other things, this affects the following aspects:
- the location within the galaxy (galactic habitable zone ),
- the nature of the central star,
- the general configuration of the planetary system
- the position within the planetary system (circumstellar habitable zone),
- the size or mass of the planet,
- the presence of a large moon in orbit of the habitable planet,
- the existence of geological conditions for a planetary magnetic field and plate tectonics ,
- the chemical composition of the lithosphere , atmosphere and oceans
- as well as the occurrence of "evolutionary" events, such as massive glaciation and impacts by comets or asteroids at greater intervals.
The emergence of intelligent life may have required other rare preconditions as well.
In order for life on a small, rocky planet to be possible, according to Ward and Brownlee, the values of the parameters would have to be within very narrow limits. The universe is so big that it could contain very many planets similar to Earth. If these planets exist, it can be assumed that they are many thousands of light years apart. These huge distances make it practically impossible for one intelligent species that has arisen anywhere to communicate with another (in order to solve the Fermi paradox). A supernova is a prerequisite for life, but it can also mean its end. Similar is the case with asteroids, which bring chemical compounds and water to Earth, while too much bombardment can lead to mass extinction of living things.
It is also argued that the development of the earth was a stroke of luck and not so easily repeatable. Even if one assumes that there are a thousand solar systems with the same initial state, one would expect that the nature, size and orbital properties of their planets would be too different for a repetition of evolution, similar to the development on earth, in one of them Allow systems. The four inner rocky planets can be used as evidence of this: They are made of very similar original material, but differ fundamentally from one another.
The first forms of life arose at least 3 billion years ago (but could be even older; it is assumed up to 3.8 billion years ago). After that, it took a very long time (until about 600 million years ago) for complex multicellular living beings (animals) to develop, for which there could be a wide variety of reasons. Among other things, oxygen has only been in the atmosphere for about 2 billion years, and the enrichment by living things that operated photosynthesis - which thus became a prerequisite for further development itself - proceeded very slowly.
The length of time from the first appearance of bacteria to the Cambrian explosion about 540 to 490 million years ago suggests that complex life as we know it on Earth takes a very long time to develop (and requires enough oxygen in the atmosphere) , and that the conditions must also be very precise. This development also took place in the oceans. That means that more highly developed life only exists in the last tenth of the earth's previous lifespan. The further development of the species did not take place gradually at that time, but in larger leaps in relatively short times. The complexity of life apparently did not develop linearly (as is usually assumed due to the theory of evolution ), but in very irregular jumps. That is why research into the Cambrian Explosion is of great importance in assessing the likelihood that planets have developed on which life is possible. The rare earth hypothesis also supports the fact that animal life is very rare and that the leap from simple to complex life is very unlikely.
Another argument is that complex life, due to its incomparably more complicated structure, is much more sensitive to cosmic catastrophes than the simply structured unicellular prokaryotes ( bacteria and archaea ).
In addition, it cannot be estimated how high the barrier is between more highly developed animals and intelligent living beings that could communicate with other species in space or even travel or colonize other planetary systems.
The galactic habitable zone
According to the rare earth hypothesis, in most of the known universe, as in large parts of our galaxy, complex life cannot exist. Ward and Brownlee refer to these regions as "death zones". The areas where complex life could occur are called galactic habitable zones . This zone depends primarily on the distance from the galactic center . Increasing distance from the center has the following effects:
- The metallicity of stars decreases, and metals (in astronomy, all elements except hydrogen and helium are called metals) are necessary to form terrestrial planets . The heavy elements on earth also ensure that the plate tectonics is driven by the heat generated by the radioactive decay in the interior of the planet.
- The X-rays and gamma rays from the black hole in the center of the galaxy and from the neutron stars near the center are becoming less intense. Rays of this type are considered dangerous for complex life. Therefore, the rare earth hypothesis argues that the early universe and possibly present regions in the center of the galaxy, where star density is high and supernovae occur more frequently, are unsuitable for the development of complex life. Radiation from a supernova less than a light year away would sterilize the earth. A supernova would affect life up to a distance of 30 light years.
- Gravitational disturbances of planets and planetesimals by nearby stars become less likely, as the star density decreases with increasing distance from the galaxy's center. Therefore, the further the planet is from the center of the galaxy, the lower the risk of the planet being hit by a meteor bolide. A sufficiently large impact could wipe out the entire complex life of a planet. The likelihood of one star getting too close to the other also decreases, which would lead to orbital disruption of the planets, which could end in a collision, a fall into the sun or a departure from the planetary system.
(1) excludes outer regions of a galaxy; (2) and (3) on the other hand the inner regions, globular clusters and the spiral arms of spiral galaxies . These arms are not physical objects, but regions of a galaxy that have a higher rate of star formation as they move very slowly across the galaxy in waves. Especially in globular clusters, the density is extremely high with typically 100,000 stars with a diameter of 10 to 100 light years. The planets would be constantly disturbed by the gravitational effects of the neighboring stars, novae and high-energy rays. If you move from the inside of the galaxy outwards, the chances of finding living-friendly conditions first increase; further out they become less favorable again. The habitable zone is therefore embedded in a ring between the hostile center and the outer areas. The system must be stable for a long time in order to give life the time it needs to develop. The sun is located in this area of the Milky Way system (between the large spiral arms) with low star density.
If a planetary system is now in this habitable zone (i.e. neither in the galactic center nor in the spiral arms), it must still be ensured that it remains in this area as long as the development of complex life requires. If the galactic orbit of the planetary system were eccentric (elliptical or hyperbolic), the planet would also get into the areas of the spiral arms. If, on the other hand, the orbit is almost circular and the orbital speed corresponds to that of the spiral arms, the star will only gradually, if at all, enter the spiral arm region.
Elliptical or irregular galaxies are generally less suitable for life because there is less material available for star formation. This leads to less star formation and old stars dominate. In open star clusters there are mostly very young stars with a typical age of 10 million years. Due to the lack of time and also possible disturbances of the planetary orbits by the neighboring stars, these places are also excluded from life.

Proponents of the rare earth hypothesis conclude from this that a life-giving star must have a near-circular orbit around its galactic center. The required synchronization of the orbital speed of a central star with the wave speed of the spiral arms can only take place within a fairly small range of the distance from the galactic center. This region is called the "galactic habitable zone". Lineweaver et al. calculate that this galactic habitable zone is confined to a ring 7 to 9 kiloparsecs in diameter . No more than 10% of the stars of the Milky Way system fall into this area. Based on conservative estimates of the total number of stars in the galaxy, that leaves about 20 to 40 billion stars. Gonzalez et al. would cut the number in half. They assume that a maximum of 5% of the stars of the Milky Way system fall into the galactic habitable zone.
The sun's orbit around the center of the Milky Way is in fact almost circular. The orbital period is 226 million years. It thus coincides almost exactly with the rotation period of the galaxy. Karen Masters calculated that the sun moves through a large spiral arm of the galaxy every 100 million years. In contrast, proponents of the rare earth hypothesis believe that the sun has not yet crossed a single spiral arm since it was formed. However, some researchers believe that multiple mass extinctions are related to previous spiral arm crossings.
Metallicity
There is no known way to create life without complex chemistry. This chemistry requires metals , that is, elements other than hydrogen and helium. Only hydrogen with 75% and helium-4 ( 4 He) with 25% mole fraction , in addition to lithium that is hardly present today , were created directly after the Big Bang . This happened in the first fusion process, also known as primordial nucleosynthesis . This produced 0.001% deuterium and traces of helium-3 ( 3 He) and beryllium.
Medium-sized stars like the sun first synthesize helium from hydrogen ( proton-proton reaction ). The three-alpha process can later begin in them, during which helium is converted into carbon. All heavier elements can only be hatched in giant stars .

The only known mechanism that creates and distributes metals is a supernova explosion. The presence of metals in stars is revealed by their absorption spectrum . A study of star spectra shows that many, possibly most, stars are poor in metals. Low metallicity characterizes the early universe, globular clusters and other stars that formed when the universe was young, stars in most galaxies that are not large spiral galaxies , and stars in the outer reaches of all galaxies. Therefore, metal-rich central stars, which could enable complex life, are most likely to be found in the quiet areas far away from the center in the large spiral galaxies. In addition, radiation exposure is generally lower in these regions.
It can be assumed that one or more supernovae must have occurred in the stellar neighborhood not too long before the formation of the solar system. The frequency of supernovae decreases over time and with it the formation of heavy metals. Five billion years ago the number of supernovae was even higher.
When the earth and the other terrestrial elements were formed from part of the primordial nebula of the solar system , the light elements could not hold on. In addition, the composition of the elements in the inner area of the flat disk of matter was different than further outside, where the gas planets were formed. As a result, the rocky planets differ fundamentally from the gas giants (which correspond more to the average mass ratio of the elements in the solar system). 99.86% of all matter in the solar system is contained in the sun. The planet Jupiter takes up two thirds of the remaining 0.14%. The sun, a star of Population I , contains about 25% more metals than other nearby, comparable stars and is therefore a special case.
Distribution of elements on earth and their meaning for life
The most common elements in the earth's mantle are (in percent by weight) oxygen (45%), silicon (22%) and magnesium (23% ) . In the chemical compounds SiO 2 ( silicon dioxide ) with 46% and MgO ( magnesium oxide ) with 37.8%, these elements form the main mass with the most common forms. In the earth's core , iron is by far the most common element with a mass fraction of 79.4%. Overall, the earth consists of around 30% oxygen, 30% iron, 15% magnesium, 15% silicon and the rest of other elements .
It is a prerequisite for life in a solar system that matter contains metals. Getting the right amount of carbon for carbon-based living is also important. But it shouldn't be too much either, so as not to force the greenhouse effect too much. Carbon is a fundamental element of life because it can form many variations of compounds with other elements. Although silicon can also form very many compounds (and it could serve as the basis for life in other forms, as some scientists suspect), it is much less suitable than carbon. Hydrogen, which exists in connection with oxygen in the form of seawater, makes up only 0.1%, carbon only 0.05% of the total mass of the earth.
A dense core of iron, nickel and other heavy metals is also important for a planet that should offer space for life . It can create a magnetic field that shields the planet from harmful rays from space. In addition, the heavy metals include numerous elements that only contain radioactive isotopes . Due to their radioactive decay , uranium and thorium in particular contribute to the fact that heat is produced inside a planet and that its cooling is greatly slowed down. The heat is released to the outside by convection , which is a prerequisite for the creation and maintenance of plate tectonics . This in turn is accompanied by volcanic activity , which is an important source of the greenhouse gas CO 2 and thus ensures balanced global surface temperatures.
In addition to oxygen, carbon, nitrogen and hydrogen, life itself needs many different chemical elements. 26 different metals have been found in more advanced life.
A suitable central star
It is assumed that a star has rocky planets within the habitable zone. Although the habitable zone of hot stars like Sirius or Vega is wide, two problems arise:
- Assuming that terrestrial planets formed closer to the central star (as in our solar system), the planet would be too close to the main star to be in the habitable zone. However, this would not rule out a natural moon from a gas giant. Hot stars also emit more ultraviolet rays that would ionize and ultimately erode any planetary atmosphere . Too intense UV light would break down biological molecules. The sun emits less than 10% of its total radiation in the ultraviolet range, whereas hotter stars like Sirius emits more than half.
- As mentioned above, hot stars have a short lifespan. They develop into red giants within a billion years . This period of time may be too short for the development of higher life.
According to these considerations, massive and powerful stars of the types F6 to O (see spectral classes ) cannot offer any multicellular life the necessary conditions, as can all stars that are outside the main sequence such as white dwarfs , neutron stars , pulsars , brown dwarfs (strictly speaking none "Real" stars are) and stars that are in the giant stage. Furthermore, in all likelihood, more highly developed life cannot develop on planets whose sun is a variable star .
On the other hand, the smaller red dwarfs , typically around 10% of the solar mass, have habitable zones with a small radius. This proximity to the main star means that one side of the planet is always facing the star, while the other side is always in the dark - a fact known as bound rotation . The side facing the sun becomes very hot, while the side facing away remains very cold. Such planets within the habitable zone with a short distance to the main star are also exposed to a greater risk of solar flares , which lead to an ionization of the atmosphere and would consequently be hostile to complex life. Rare earth proponents claim that life in such systems is impossible. Still, some astrobiologists suggest that there could be a life zone under the right circumstances. If a planet still had a CO 2 -rich atmosphere, the dark night side could also be supplied with heat. This is a central point in the debate surrounding this theory, since these late K and M stars make up about 82% of all hydrogen-fusing stars.

Rare Earth proponents claim that the “matching” stellar types of a central star range from F7 to around K1. Such stars are rather rare: G-type stars like the Sun - yellow dwarfs between the hotter F and cooler K stars - comprise only about 9% of all hydrogen-fusing stars in the Milky Way. Here, too, the distance between the planet and the star must be sufficiently large and the habitable zone must extend far enough from the star so that the planet is not forced into a bound rotation.
Older stars like the red giants and the white dwarfs are also very unlikely to make life possible. Red giants are common in globular clusters and in elliptical galaxies . White dwarfs are mostly dying stars that have already passed the stage as red giants. The diameter of a red giant has increased significantly compared to the younger star. A planet would be in the habitable phase as long as the star is in the early or middle stage. If the star expanded at the end of its evolution into a red giant, this planet would be literally grilled (although then a planet would theoretically become habitable at a much greater distance).
The energy output of a star over its lifetime should change only slowly. Variable stars such as the Cepheids would be very poorly suited to make life possible. If the energy output of the central star suddenly drops - even for a short time - the water on the planet could freeze. On the other hand, if the energy release increases significantly, the oceans could evaporate, which would increase the greenhouse effect. This would prevent oceans from forming again later.
About two thirds of all stars are members of double or multiple systems . In such systems the formation or long-term survival of planets is only possible under certain circumstances. Studies of the abundance of exoplanets in binary systems have shown that circumbinary (P-type) gas giants, i.e. those that move their orbits around both stars together, are roughly as frequent as planets of approximately the same size orbiting a single star, if the two stars are at a moderate distance from each other (orbit around 7 to 41 days). Circumbinary gas giants, however, are rare in double stars orbiting very closely around each other, which could be related to the presumed formation of such systems under the gravitational influence of a third star plus tidal friction . Planets that orbit only one of the two stars (circumstellar or S-type planets) are three times rarer than in binary systems in which the partner stars are less than 50 astronomical units (about 7.5 billion kilometers) apart with single stars or with double stars with a relatively large distance from each other, regardless of the size of the planet.
Circumstellar habitable zones
The example of Earth suggests that complex life requires water in a liquid state. That is why the planet must be at a suitable distance from the central star. This area is called the habitable zone . The habitable zone represents a ring-shaped area around the central star. If a planet is too close or too far from its sun, the surface temperature will not allow water to be in a liquid state (although liquid water, such as in Europa , Enceladus and Ceres , could exist beneath the surface). Kasting et al. a. (1993) suggest that the habitable zone of our sun ranges from approximately 0.95 to 1.15 astronomical units . It is important that the planet orbit the star in a stable, not too eccentric orbit. The received solar energy can fluctuate by up to 10% without having a major impact on the habitability. The habitable zone used to be set even closer, until the thermostatic effect of the CO 2 silicate cycle was discovered. The warmer the climate, the higher the continental rate of weathering of silicate rocks, which removes more carbon dioxide from the atmosphere, which reduces the greenhouse effect. Most of CO 2 enters the atmosphere through volcanic emissions.
The habitable zone varies with the spectral type and the age of the central star. Since 95% of the stars are less massive than the sun, it does not represent an average star, as is commonly assumed. The life zone for a main sequence star moves slowly and steadily outwards until it becomes a white dwarf , at which point in time the habitable zone disappears. The habitable zone is closely related to the greenhouse effect , which is caused by water vapor and trace gases such as carbon dioxide (CO 2 ) or methane in the atmosphere. The average surface temperature of the earth is around 40 ° C higher than it would be without this effect.
Since a star becomes more and more luminous over time, the zone that is in the habitable area over the entire time is also known as CHZ (constant habitable zone). In its early days, the sun's radiation output was around 30% less than it is today. In 4 billion years the sun will be twice as bright as it is today. Then the stage begins as a red giant, which can last up to a billion years. There is also the AHZ (animal habitable zone), which is narrower than the habitable zone, as temperatures above 50 ° C would prevent more complex life.
Planetary system
A gas cloud that creates a star can also create gas planets , Jovian planets with little metal, such as Jupiter or Saturn . However, these gas planets do not have a solid surface that should be a requirement for complex life (although their moons might have one). According to Wards and Brownlees' claims, a solar system producing complex life should be structured more or less like our solar system, with smaller dense inner planets and gas planets in the outer areas. More recent research results, however, call this strict requirement into question.
The transport of biogenic molecules from the outer solar system to the inner planets is also important. This material reaches the inner planets by deflecting the large gas planets in the outer solar system. Even today, the earth absorbs around 40,000 tons of matter every year. In the area of the earth there was comparatively little water and carbon. If the earth had been formed from the material that is in the asteroid belt between Mars and Jupiter, there would be too much water. The earth would have been covered with an ocean several hundred kilometers deep and the atmosphere would have been enriched with too much CO 2 . This would have created a strong greenhouse effect.
Gas giants in the neighborhood

When Ward and Brownlee first published their book in 2000, it was believed that gas giants, especially Jupiter in our solar system and to a lesser extent Saturn , were important to the development of life because they kept asteroids away from an inner terrestrial planet that was home to life . Without Jupiter, the number of 10-kilometer impacts was estimated to be 10,000 times as large.
However, recent computer simulations show that the situation is more complex: It seems as if Jupiter causes three times more asteroid impacts than it averts. However, if Jupiter were to be replaced by a planet the size of only Saturn, the asteroid impact rate would again roughly double compared to Jupiter.
Gas giants form particularly in the colder central to outer regions of the solar system, where they accumulated material faster and earlier than the more inward terrestrial planets. If a gas giant had accumulated around 15 times the mass of the earth in the form of dust and solid material, gravity was enough to attract the light gases helium and hydrogen. The higher the mass, the faster the planet grew, until finally there was no more material left.
In that sense, they were taking material away from the planets further in. Had it not been for the outer gas giants, Mars and the planet that formed at the site of the asteroid belt might have become as large as Earth. Seen in this way, Mars is a “victim” of the gas giants and has been hindered in its development by them.
Some scientists assume that there were several large gas giants in the past. Only the last, Jupiter and its outer neighbors, remained in their orbit. The first generation slowly drifted in elliptical orbits towards the sun until they finally fell into it. This could also explain the high number of " Hot Jupiter " discovered so far . Since Jupiter, as in our solar system, which is a billion kilometers or more away from the main star, cannot yet be well proven, the preliminary impression is incorrect or confirms that our solar system is supposed to be an exception. The verified Hot Jupiters also make up a low percentage of planetary systems and cannot yet be regarded as representative.
Gas giants can make a planetary system relatively unstable. A third large gas giant (next to Saturn and Jupiter) alone could have caused planets to be thrown out of the solar system or fallen into the sun. The orbits must also be very circular. A gas giant too close to Earth would also be problematic (after all, Jupiter is five times farther from the Sun than Earth).
Orbit Disorders
A gas giant cannot be too close to an object that houses life (unless the object is one of its moons). Staying in close proximity could disrupt the orbit of a life-bearing planet, either directly or by entering the habitable zone. Other unfavorable arrangements such as very eccentric orbits also pose a problem. The Hot Jupiters discovered in recent years are such unfavorable planets. They could seriously disturb the orbits of the other inner planets.
According to Newton's law of dynamics (Newton's second law), chaotic planetary orbits can arise, especially in a system that contains large planets such as gas giants with highly eccentric orbits ( three-body problem ).
The requirement for stable orbits excludes systems with exoplanets that have large planets with narrow orbits around their central star (Hot Jupiters). It is assumed that such gas giants formed much further away from the central star, but then migrated inward over time. In doing so, they could have mixed up the orbits of the other planets that are circling in the habitable zone.
Impacts and mass extinctions
Statistically speaking, cosmic objects 0.5 to 1.0 kilometers in size impact every 500,000 to 10 million years. Asteroids 10 kilometers in size or other celestial bodies hit the earth about every 100 million years, with the effect of mass extinctions or mass extinctions . The last major impact and its consequences for the biosphere led to the extinction of the dinosaurs 66 million years ago on the Cretaceous-Paleogene border . At the time of the great bombardment around 3.9 billion years ago, the earth was probably sterilized several times. If life had already existed then, it should have developed anew. However, the assumption of a series of impacts within a limited time window is not without controversy.
Water transport by comets
Due to their material, the terrestrial planets are initially very dry when they form. A model by Dr. John Chambers of NASA Ames Research Center showed that water transport from the outer comets to the interior of a planetary system worked best if the planets in the position of Jupiter and Saturn were much smaller than these two gas giants in our solar system. Therefore, the efficiency of water transport in our planetary system was comparatively low. Even smaller gas planets like Uranus or Neptune would provide considerably more water for terrestrial planets. On the other hand, such a planet would soon be completely covered with water, and this would not be conducive to species-rich evolution.
A suitable planet
The planet should be in the habitable zone. Temperatures should be in a range where water can exist in a liquid state. In addition to water, a sufficient amount of carbon (or, for hypothetical other life forms, silicon) should be available. These elements allow a wide variety of connections with other chemical elements that contribute significantly to the formation of amino acids and proteins .
Furthermore, a distinctive atmosphere with oxygen, which protects against harmful radiation and allows metabolism, and a magnetic field, which deflects cosmic rays, are fundamental to life as we know it. The axis of rotation should not be tilted too much, and the rotation should not take too long.
It doesn't have to be a planet. A moon on a larger planet such as a gas giant could also provide the conditions for life at the correct distance from its sun. Possible candidates in our solar system would include the Jupiter moon Europa and the Saturn moons Titan (→ Life on Titan ) and Enceladus . Since they are very far from the sun (and therefore it is too cold on the surface), life could only form in a hypothetical ocean below the surface.
Size of a planet
A planet that is too small cannot hold a dense atmosphere. As a result, the temperature is subject to greater fluctuations and the average temperature drops. Large and consistent oceans would not be possible. A small planet would tend to have a rough surface with high mountains and deep canyons. The core would cool down sooner and plate tectonics would not last as long as it would on a larger planet, or could not occur at all. In addition to the task of ensuring a constant climate, the atmosphere should also be present in the right composition and with the appropriate pressure.
Small rock planets like Earth could be common, says Michael Meyer of the University of Arizona.
“According to our observations, about 20% to 60% of the sun-like stars could form earth-like planets, not unlike the processes that led to the formation of the earth. This is very exciting. "
Meyer's team discovered interstellar dust near recently formed sun-like stars. This is seen as a by-product of the formation of Earth-like planets.
the atmosphere
The composition of the atmosphere is controlled by biological processes. The terrestrial atmosphere differs significantly from the atmospheres of the other terrestrial planets, both in pressure and in their material distributions. Since it is in chemical imbalance, it can only be maintained and renewed in this form by living beings. Otherwise oxygen would react quickly with the matter on the surface or with the nitrogen. In addition, without living beings, the CO 2 content of the atmosphere would steadily increase and increase the greenhouse effect. Even from a great distance, one would be able to deduce biological processes in a planet with such or a similar composition of the atmosphere. This search for signatures of atmospheres would therefore have been one of the focal points of the planned search with the Terrestrial Planet Finder .
water
The water cycle is important for life on land. Water is a good solvent. This is important both for the distribution of elements on the surface and for the transport of substances to the cells in living beings. In the regions where there is a lot of water there are about ten times more species of living things than in arid areas.
The origin of the water has not yet been fully clarified. Part of it comes from the earth itself (as a result of the formation of planets), another part goes back to comet impacts (see also origin of terrestrial water ). Overall, water makes up about 0.5% of the earth's mass.
The fact that water ice is lighter than water in its liquid state is important for life in lakes and oceans, since water freezes over from above and life is protected by this insulating layer. The water can form hydrogen bonds. This adhesive property is advantageous for the DNA , since the connections between the bases can easily be separated, e.g. for copying the genetic information.
For life as we know it on earth, the following requirements must be met:
- there must be enough water to form oceans
- water must exist on the surface
- Water must not escape into space
- it must be mainly in a liquid state.
Big moon

The terrestrial moon is unusual because the other rocky planets in the solar system either do not have a moon ( Mercury and Venus ) or have tiny satellites like the likely captured asteroids of Mars . Only the dwarf planet Pluto has a comparatively large moon.
The moon has three important effects on the earth and thus also on life. It stabilizes the earth's axis over a long period of time (so it fluctuates at most one to two percent in the long term), causes the tides (the tidal forces of the moon are twice as great as those of the sun) and slows the earth's rotation.
The formation of the moon is probably due to the impact of a Mars-sized object, Theia , on the young earth. This huge impact also caused the current inclination of the axis of rotation and had an impact on the speed of rotation. After its formation, the moon was only about 25,000 km from the earth's surface and the earth rotated in about 5 hours. It is conceivable that there was also such a collision on Venus, but that it was retrograde and thus caused the extremely slow rotation and that no moon was left either. If the Mars-sized body hadn't hit the ground at this angle and at this speed, there would probably be more water, carbon and nitrogen on earth. A high greenhouse effect might be the result.
The relatively short period of rotation reduces the daily temperature fluctuations and thus makes photosynthesis possible. The rare earth hypothesis also asserts that the axis inclination must not be too large or small (relative to the orbit plane). A planet with a very steeply inclined axis of rotation would cause extreme climatic variations in the seasons that would not be conducive to complex life. The only slightly inclined axis results in constant seasons and this also has a stabilizing effect on the climate. If the earth's axis were inclined more than 54 degrees, the poles would receive more radiation from the sun over the year than the equatorial regions.
On the other hand, a planet with little or no inclination would again lack the stimulating effect on evolution. Seen in this way, the inclination of the earth's axis of rotation is “just right”. The gravity of a large satellite also stabilizes the inclination of the planet's axis of rotation. Without this effect, the axis inclination would fluctuate chaotically. This would make it impossible for complex life to develop. The moon moves away about 4 cm per year. In 2 billion years it will be too far away to be able to continue to stabilize the Earth's axis of rotation in the long term. At the same time, the sun will be much hotter during this time than it is today. If the orbit were opposite to the Earth's axis of rotation, it would get closer and closer to the Earth and finally crash on it. This will be the case with the moon Triton in the distant future when it collides with Neptune , provided that it is not torn apart by the tidal forces beforehand.
If the earth had no moon, the tides of the oceans would be extremely moderate due to the action of the sun alone. A moon strengthens the tidal pools , which in turn are essential for the formation of macromolecules and complex life.
A large satellite also increases the likelihood of plate tectonics due to the tidal action on the planet's surface . After the formation of the moon, the tidal forces were immense. The floods were several hundred meters high and the earth's crust was tumbled through. The impact that led to the formation of the moon may have started plate tectonics in the first place. Otherwise the earth's crust would simply cover the entire earth and leave no room for the oceanic earth's crust . It is possible that the tremendous mantle convection that is necessary to propel continental motion would not have occurred without the inhomogeneity of the earth's crust. However, there is convincing evidence that plate tectonics existed on Mars in the past that did without this initiating mechanism.
If the great impact is the only way for an inner rocky planet to get to a great moon, every planet in the circumstellar habitable zone would have to emerge as a double planet. The smaller companion would have to be massive enough to create a large moon in the event of an impact. An impacting object of this size is not necessarily impossible. Recent work by Edward Belbruno and J. Richard Gott of Princeton University suggest that a suitable object could emerge in the Trojan areas (L4 or L5 Lagrange point ).
Plate tectonics and the carbon cycle

The plate tectonics has an important function for the release of heat from the Earth's interior. It also has an impact on the earth's climate. It works like a thermostat and ensures that the global average temperature on earth is kept largely constant for billions of years. The greenhouse gas carbon dioxide (CO 2 ) is continuously withdrawn from the earth's atmosphere through various processes . This includes the binding of carbon in biomass or biogenic minerals through photosynthesis. Much of this process takes place in the oceans, including a. in the form of carbonate precipitation (limestone formation). However, CO 2 also dissolves directly in sea water. Furthermore, CO 2 is bound by continental rock weathering . The wetter and warmer the earth's climate, the higher the continental rate of weathering, which removes more CO 2 from the atmosphere and thus weakens the greenhouse effect. Conversely, dry and cool climates cause less binding of carbon dioxide, which in turn leads to warming. Due to the subaeric volcanism at subduction zones , CO 2 is released into the atmosphere. a. returned to the upper mantle from the recirculation of limestone, where it was broken down again into CO 2 . Due to these interactions and feedbacks (the list is not complete), the temperature range of +5 ° to +40 ° C, which is so important for higher life, is maintained in most areas of the world.
A planet exhibits plate tectonics only if its chemical composition allows it. The only known long-lived source of the required heat is radioactive decay deep inside the planet. Continents have to be made of less dense granite so that the basalt rocks of the ocean floors can submerge below. It is believed that subduction, a fundamental element of plate tectonics, only works properly when water-saturated oceanic sediments act as lubricants. This could also be the reason why there are no plate tectonics on Venus and Mars. These processes may have existed earlier, when liquid water might still exist on the surface of these two planets. With Venus, the heat build-up inside the planet caused its entire surface to melt about a billion years ago. By the time the earth's crust solidified for the first time (more than 4 billion years ago), around half of the heat had already escaped from the earth's interior.
Without plate tectonics there would be no larger, contiguous land masses and no high mountains in the form in which we know them. The formation of today's continental cores took place in a relatively short time. From 2.7 to 2.5 billion years ago, the proportion of continental crust grew from 5 to 30%. Plate tectonics also ensures the continuous enrichment or return of chemical elements and compounds into the oceans, both directly through material replenishment from the earth's mantle and through the water cycle, with dissolved material entering the sea through rivers. Plate tectonics used to be more intense and the plates moved faster because more heat was stored in the earth's interior. It was particularly intense u. a. at the time of the Cambrian explosion. At that time a huge land mass ( pannotia ) broke up into smaller continents.
Plate tectonics is the cause of continental drift . This in turn has various effects on the global climate and the living world. The drift of large land masses across the polar regions leads to large-scale glaciations, which in turn has feedback on the climate and the like. a. as a result of an increase in albedo , d. H. the value of the reflection of sunlight from the earth's surface. If larger land masses break apart, this leads to the isolation of the living worlds on the land and on the shelves , since the seas represent barriers that are difficult to bridge for most land dwellers and the deep sea or the open ocean for most shelf dwellers. Seen globally, this increases biodiversity. The current configuration of the continents with five relatively isolated continental blocks (North America, South America, Africa-Eurasia, Australia and Antarctica) favors a rather high global biodiversity. There are around 3 to 30 million different species today.
NASA scientists C. McKay and H. Hartmann believe, however, that plate tectonics in particular delayed the development of higher life for a long time, because it prevented the important prerequisite, the availability of sufficient oxygen, for so long. The oxygen is previously consumed by oxidation. Only after oxygen saturation in the sea can oxygen accumulate in the atmosphere. However, plate tectonics require more oxygen due to the constantly new oxidation process. The two scientists also assume that if life existed on Mars, it could have formed much faster (possibly within 100 million years).
"Inertia-related exchange event"
There is strong evidence that the rate of continental drift was abnormally high during the Cambrian Explosion. In fact, in 15 million years (or less) continents moved from arctic to equatorial latitudes and vice versa. Kirschvink et al. have proposed the following, albeit controversial, explanation: a 90 ° change in the inclination of the earth's axis was caused as a result of the imbalance in the distribution of the continental masses relative to the earth's axis. The result was huge changes in the climate, in the ocean currents and other changes that affected the whole earth in a short time. They called their hypothesis inertia conditional exchange event (Engl. Inertial interchange event ). This scenario is not yet recognized by science, but if such an event occurred it would be a very unlikely event. If such an event was necessary to enable the evolution from simple life to more complex life (such as sponges and coral reefs ), that would be another reason why complex life should be so rare in the universe.
Climate, temperature and the greenhouse effect
The global average temperature without the greenhouse gases would be −18 ° C. In fact, it is 33 ° C higher at +15 ° C. The gases in the atmosphere that contain three or more atoms are responsible for this. These ensure that infrared radiation is not radiated back into space unhindered and thus lead to a warming of the earth. In this context, particular mention should be made of carbon dioxide (CO 2 ), water vapor, ozone (O 3 ) and methane (CH 4 ), which consists of five atoms. Although these are so-called atmospheric trace gases with only small proportions by volume (water vapor has up to 4%, CO 2 0.04%), they are considered to be decisive for the climate on earth. The greenhouse effect is particularly important for animal life, which develops best at a temperature of +2 ° C to +45 ° C.
If the sun gave off less radiation for a longer period of time, the continents would be covered with thick ice sheets and the oceans with a thick layer of sea ice. Even if the sun shines again with normal luminosity, the strong albedo would radiate too much heat again to thaw the oceans again. This corresponds to a “self-reinforcing global runaway icehouse effect ”. Conversely, with longer-term increased energy output from the sun, the oceans would evaporate, and even afterwards, with normal luminosity, there would no longer be any appreciable cooling due to the greenhouse effect of the water vapor ("self-reinforcing global greenhouse effect" or global runaway greenhouse effect ), cf. . galloping greenhouse effect .
Magnetic field
On the one hand, the magnetic field protects against deadly rays and solar wind ; on the other hand, it ensures that the atmosphere is not gradually eroded, as was the case with the planet Mars .
Why simple life should be more common
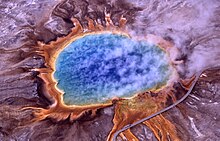
However, some proponents of the rare earth hypothesis believe that simple life could come about with relative ease. However, the assumption has not been proven, since no life forms are known except on earth.
In the early 1980s, small organisms were found in environments that were so extreme that until then it was assumed that life could not exist there.
Deep underground, at depths of thousands of feet in boiling water and near the black smokers ( black smokers ) were bacteria and archaea discovered that under extreme conditions such as high heat, high pressure, completely without oxygen and without light be able to live. Species have been discovered that do best at 105 ° C and reproduce up to 112 ° C. But unicellular organisms have also been found that can withstand temperatures of up to 121 ° C. Other species are characterized by the fact that they live in very salty, acidic or basic environments. Living beings have also been found in icebergs and frozen sea ice. Many of them do not use light as an energy source (photosynthesis), but rather hydrogen sulfide or methane (see also extremophiles ).
There is life in the earth's crust too. When drilling in South Africa, living things were detected at a depth of 3.5 kilometers at temperatures of over 70 ° C. The astronomer and geologist Thomas Gold suggested that the mass of all living things below the surface could be up to seven times the mass of the living things that live on the surface. Seen in this way, life could also be possible under the surface of Pluto , for example .
Accordingly, one can conclude that simple life does not place as high demands on its surroundings as was previously assumed. This encourages hopes that there could be life on other celestial bodies under harsh conditions. This would also apply to planets that are far away from their sun, as these living beings would not be dependent on its warming light. This also applies to moons, such as the Jupiter moon Europa in our solar system or the even more distant Saturn moons Titan and Enceladus . 3 billion years ago, Mars had much higher temperatures, the atmosphere was denser, and there was water on the surface. So it shouldn't have been too difficult for microbes to evolve and live on Mars. If the extremophiles are included, the concept of the habitable zone would have to be redefined.
Whether life can only exist on a planet now (and was transported here) or whether it originated there is an important difference. It is worth mentioning, however, that it is precisely the oldest living beings like these that have hardly changed that seem to be those that can thrive even under very inhospitable conditions. The most resistant are the archaea, there are also some thermophilic bacteria. Due to unfavorable climates such as long ice ages or cosmic catastrophes, more highly developed organisms can quickly become extinct, whereas simple life can bridge these times longer.
Open questions about the origin of life
There are still several unresolved questions here, such as whether RNA was the precursor to DNA . Since they are mutually dependent, this poses a " chicken and egg problem " as to which came first. Messenger RNA could, however, have taken over partial tasks from DNA ( RNA world hypothesis ). In addition to the actual task of forming proteins, the task of storing the construction plans can also be partially taken over.
Likewise, the question of whether all life developed from a single DNA molecule that emerged for the first time or whether several molecules emerged independently of one another is still unanswered. The process of transition from inanimate to animate matter is also open. Even crystals exhibit similar properties to replicating biological forms. In addition, it would have to be answered when and why the formation of eukaryotes or separation from the prokaryotes occurred. This did not happen until one to one and a half billion years after the prokaryotes first appeared. Due to their simpler structure, prokaryotes can exist in the temperature range from 0 to 100 ° C, eukaryotes only up to around 60 ° C. They have been reliably proven at the earliest 1.6 billion years ago, i.e. around the time when animal life was able to form due to sufficient oxygen for the first time.
It is uncertain to what extent the extremophiles and the specimens are similar 3 billion years ago or whether the genome has changed significantly since then. It could be that there was a period of evolutionary experimentation when a single species ultimately prevailed. While prokaryotes have particularly adapted to chemical processes for energy production, eukaryotes have developed more in the direction of size and variations in body structure.
Viruses are a special case because they are not viable on their own, but can nevertheless multiply by infiltrating and infecting other cells in order to spread their own genetic material.
Answering these questions would help to better estimate the likelihood of primitive life arising.
Situation in the early days of the earth

Life should have developed very quickly on the primordial earth after the great bombardment subsided (around 3.8 billion years ago) or the settlement should have happened quickly at this time. Living things were detected as early as 3.5 billion years ago at the latest. Stanley Miller (co-initiator of the Miller-Urey experiment ) claimed that the transition from prebiotic matter to the first cyanobacteria could have taken place within 10 million years.
First life forms
Contrary to older beliefs, the primordial atmosphere was not reducing (with a high proportion of methane and ammonia), but consisted primarily of carbon dioxide (100 to 1000 times more than today). Only the ocean water had chemically reducing properties due to the lack of oxygen. It was hot (both the atmosphere and ocean water), the water covered the entire surface of the earth except for a few volcanoes, and many chemical elements and compounds were present due to the violent volcanic activity. Time and again, a large part of the sea evaporated through cosmic impacts, only to then rain down again. The intense UV radiation (due to the lack of free oxygen, no ozone layer could form), which reached the surface almost unhindered, was an obstacle to life.
The cradle of life was evidently not the tepid ponds, as Darwin thought, but rather the seabed, protected from meteorites and UV radiation, or it was deep under the earth's surface. The first living things were possibly mesophilic (heat-loving) archaea (the thermophilic archaea only emerged later due to even more extreme conditions).
The theory of panspermia, on the other hand, says that life did not originate on earth, but rather originated from early Mars, which was probably much more life-friendly billions of years ago. Almost 10 percent of the material that was hurled from Mars by cosmic impacts into space landed on earth.
The first animals appeared about 1.2 billion years ago. However, this is largely controversial as no fossils have been found that appear to be more than 600 million years old. On the other hand, however, this is what geneticists claim because of the " molecular clock ". Larger ( macroscopic ) animals emerged around 600 million years ago. This raises the question of why it took so long and whether certain environmental conditions (changed chemical composition of the seas, free oxygen in the atmosphere and oceans, ice age, continental drift) are required or whether it was only a matter of time before evolution needs. This knowledge is also important in assessing whether animal life can develop on other planets. Oxygen is also a major factor in the growth of living things. Without oxygen, they usually remain so small that they are invisible to the naked eye.
Snowball earth
There were at least two global ice ages during which the entire earth (except for the equatorial regions) froze over (as opposed to more recent ice ages, which only affected the polar regions to regions of medium latitude). The first was 2.5 billion years ago, the second around 600 to 800 million years ago. Both coincide in time with important transitions, the first appearance of the eukaryotes and the beginning of the development of the animals (Cambrian explosion). After the first global Ice Age, also known as " Snowball Earth " (English. Snowball Earth ) denotes suddenly much free oxygen was present. The end of the Ice Age was triggered by the accumulation of greenhouse gases in the atmosphere from volcanoes. The creatures that survived the Ice Age at greater depths or near volcanoes or geysers had to make radical changes. Those who did that evolved in a different direction with the oxygen that was now available. Another reason for the rapid development at that time could have been the progressive isolation of living beings as a result of plate tectonics.
Cambrian explosion
In the course of the Cambrian explosion , many phyla developed , some of which are now extinct. This fact is of central importance, since all biological blueprints were "designed" in this relatively short time and the range was higher then than it is now. Reasons for this evolutionary development could be the increasing oxygen content, the abundant phosphate reserves , the rapid continental drift, a possible tilting of the earth's axis or a combination of these factors.
In the late Carboniferous about 300 million years ago, the volume fraction of oxygen in the atmosphere was up to 35 percent, higher than ever before (and also afterwards; it is currently around 21 percent). This favored growth in size , especially in the case of various arthropods .
Mass extinction
Even if the - hypothetical - largest mass extinction in the history of the earth, the Great Oxygen Catastrophe, may only have affected bacteria and arks, the term mass extinction usually refers to higher, i.e. multicellular , life. In the past 600 million years there have been around 15 major such events. The event on the Permian Triassic boundary 252 million years ago is considered by far the most devastating .
Possible causes for mass extinctions are: regionally strongly increased volcanic activity (especially the formation of magmatic large provinces ), increased radiation exposure from supernovae or gamma-ray bursts as well as impact events , disturbances in the material cycle (especially the carbon cycle ) or rapid climate change . The triggers mentioned can be mutually dependent (e.g. volcanism and impact events as triggers for disturbances in the material cycle and consequently climate change). Climate stability and the frequency of events with extra-planetary sources also depend on the closer cosmic environment of a planet (see gas giants in the vicinity , impacts and mass extinctions and the Great Moon ).
Situation on the planet Mars
Depending on the definition, Mars lies on the edge or just outside the habitable zone . There are no traces of life on him to this day, neither present nor past. This also means that it cannot be ruled out that there ever was life on Mars.
In any case, there used to be a lot more water and a denser atmosphere than today, although compared to the earth, there was much less water and an atmosphere that has always been thinner. The fact that there must have been water in the past can be seen from the dry river valleys and other erosion phenomena.
Some researchers believe that a large impact might have caused huge local damage, but the global impact of the disaster would have been less severe than it was on Earth, as the climate would have been less severe due to the small amount of water vapor and weaker greenhouse effects due to the thinner atmosphere changed so that any existing life would not have been so dramatically affected.
Research and ways to prove it
In evaluating the spectrum of the Earth's atmosphere in the infrared wave range, residents of a more or less distant star system could make the interesting discovery that, in contrast to most other planets, the Earth's atmosphere contains shares of water vapor, ozone and carbon dioxide. This is not in chemical equilibrium. Thus, they could conclude that the planet must have surface water (that the planet allows liquid water and must therefore be in the habitable zone). The detection of ozone would lead to the conclusion that there is free oxygen in the atmosphere, which would not exist without living organisms that carry out photosynthesis, since otherwise it would react quickly with other substances and can only be replenished through continuous formation.
The proven signature of oxygen, nitrogen and water vapor in the atmosphere of an exoplanet would be a strong indicator of biological processes. Oxygen makes up a large proportion of the earth (85% by volume and 45% by weight) and is in principle toxic to living beings, unless they have adapted to how it is handled and usable. They must also have developed protective mechanisms for this reactive substance.
The evaluations of the materials of the moon, which were brought to earth by the Apollo missions, have not, as expected, brought any results that could indicate life.
The Viking program did not deliver any clearly positive results from Mars either. It is still unclear what results can be expected from the Mars Sample Return program.
The SETI project searches for radio waves (and in the optical field) for clues to a higher civilization. The search is mainly focused on the frequency of neutral hydrogen at 1.42 GHz .
Rare Earth Equation
The following discussion was taken from Cramer. The rare earth equation is Ward and Brownlee's answer to the Drake equation . It calculates the number of Earth-like planets in the Milky Way that harbor complex life as:
in which:
- is the number of stars in the Milky Way system . The number cannot be estimated well because the exact mass of the Milky Way is not known. In addition, the number of very small stars is not known. makes up at least 100 billion and could be up to 500 billion if there are many faint stars.
- is the average number of planets in a star's habitable zone. This zone is very narrow because it is restricted by the fact that the average temperature over the entire time it takes complex life to develop must be in the area in which water remains liquid. Hence, the upper limit is likely.
We accept . The rare earth hypothesis can then be viewed as the product of nine rare earth factors listed below. This product is no larger than 10 −10 , but it could even be as little as 10 −12 . In the latter case it could be less than 1 or close to 0. Ward and Brownlee do not calculate the value of because there is no data on some of the factors listed below. Earth, a rocky planet, orbits a G2 star in a quiet corner of the great barred spiral galaxy, and home to the only intelligent species we know is our earth itself.
- is the proportion of stars in the galactic habitable zone (Ward, Brownlee and Gonzalez estimate this factor to be 0.1).
- is the proportion of stars in the Milky Way system with planets.
- is the proportion of planets that are rocky ("metallic") rather than gaseous.
- is the proportion of habitable planets where microorganisms develop. Ward and Brownlee believe this percentage is not too low.
- is the proportion of planets where complex life develops. 80% of the time from the time the first microorganisms arose, there was only bacterial life. Therefore, Ward and Brownlee believe that the proportion must be very small.
- is the fraction of the total life span of a planet during which complex life exists. Complex life cannot last indefinitely, since the energy output of a star that allows complex life to flourish gradually increases, depending on the star's spectral type, until the central star develops into a red giant, and then devours all planets in the habitable zone. If you allow enough time, a catastrophe can wipe out all of life, and this danger increases with duration.
- is the proportion of habitable planets with a large moon. If the formation of the moon is correctly explained by the collision theory, this number is very small.
- is the proportion of planetary systems with large gas giants. That number could be quite large.
- is the proportion of planets that are affected by an extinction event with a sufficiently small probability. Ward and Brownlee claim that the low number of such events on Earth that have occurred since the Cambrian Explosion is unusual. This value is also assumed to be relatively low.
The rare earth equation, unlike the Drake equation , does not consider the factor of probability that complex life will evolve into intelligent life that technology discovers and develops (Ward and Brownlee are not evolutionary biologists either ). Barrow and Tipler summarize the consensus among biologists that the path of evolution from primitive Cambrian chordates - for example Pikaia - to Homo sapiens was a highly unlikely event. For example, the large human brain has brought adaptive disadvantages in that its metabolism is very expensive, it requires a long pregnancy and a childhood that accounts for more than 25% of the average total human lifetime. Other unlikely characteristics of humans are:
- It is the only currently existing bipedal land vertebrate (other than birds). Combined with the unusual hand-eye coordination, this enables skillful manipulation of the physical-material environment with his hands.
- A vocal machine that allows more controlled sound shaping than that of any other mammal and enables speech . The language makes it may only be possible to cooperate that people share knowledge and create culture.
- The ability to formulate abstract concepts to the extent that the invention of mathematics, the discovery of the sciences and technology made possible. It should be noted in what short time mankind has gone through scientific and technological development.
Advocates
Proponents of the rare earth hypothesis include:
- Stuart Ross Taylor, a specialist on the solar system, is firmly convinced of the hypothesis, but its correctness is not the central point for him, but a brief introduction to the subject of the solar system and its formation. Taylor concludes that the solar system is very unusual because it has been shaped by an extremely large number of random events and factors.
- Stephen Webb, a physicist, presents and refutes possible solutions to the Fermi paradox . The rare earth hypothesis appears as one of the few remaining solutions at the end of the book.
- Simon Conway Morris , a paleontologist , argues mainly that evolution was convergent . He dedicates Chapter 5 to the rare earth hypothesis and cites it with approval. But while Morris agrees that the earth could very likely be the only planet in the Milky Way System that is home to complex life, he sees the evolution of complex life in the direction of intelligent life as quite likely - contrary to Ernst Mayr's views, which are discussed in Section 3.2 the following reference.
- John D. Barrow and Frank J. Tipler (1986. 3.2, 8.7, 9), cosmologists close to creationism, vehemently defend the view that humans are very likely to be the only intelligent living beings in the Milky Way system, perhaps even in the entire universe. But this hypothesis is not the central theme of her book, but a thorough study of the anthropic principle and how the laws of physics fit in peculiar ways to reveal the complexity in nature.
- Ray Kurzweil , a computer pioneer and self-proclaimed Singularitarianer , claims in humanity 2.0 (English title The Singularity Is Near ) that the coming singularity requires that the Earth was the first planet, was built on the sentient, technology-use life. Although other Earth-like planets could exist, Earth must be the only evolutionarily advanced culture, otherwise evidence would have been seen that another culture would have experienced the technological singularity and used all of its computing power in the physical universe.
criticism
Criticism of the rare earth hypothesis is expressed in various forms and arguments.
Exoplanets are common
There are currently 4122 exoplanets known in 3063 planetary systems (as of October 2019), and more are being discovered all the time. Alan Boss of the Carnegie Institution for Science estimates that there could be about 100 billion Earth-like planets in the Milky Way System alone. Boss believes many could have simple life forms and there could be thousands of civilizations in the galaxy. He suspects that every sun-like star has on average one Earth-like planet in its system.
Most of the exoplanets discovered so far, however, are gas giants that orbit very closely (usually much closer than the distance from Mercury to the Sun ) around their central star. It can be assumed that such planetary systems do not harbor any earth-like, water-rich planets with permanently stable orbits. Since massive objects close to the central star are most likely (and first with the methods currently used) to be discovered, one cannot in any way conclude that such systems are typical. Rather, it is a matter of a currently existing measurement artifact , and the level of knowledge about the structure of alien planetary systems will probably change with the progress in astronomical research.
Evolutionary biology
Central to the rare earth hypothesis is the following claim about evolution : While microbes may be common in the universe, complex life does not. But to date, the only evolutionary biologist who fully agrees with this view is Simon Conway Morris (2003). The hypothesis concludes that complex life is rare, as it can only develop on the surface of an earth-like planet or on a suitable moon of a planet. Some biologists, such as Jack Cohen, believe that this assumption is too restrictive and too unimaginative. You see them as a circular argument . Earth-like planets may indeed be very rare, but non- carbon complex life may have evolved in other environments. According to astronomer David Darling , the rare earth hypothesis is neither a hypothesis nor a statement, just an explanation of how life came about on earth. In his view, Ward and Brownlee have done nothing more than enumerate the factors that seem best suited to their case.
“It is not important which circumstances are unusual on earth. There are peculiarities in every planet in space. What matters is whether any of the circumstances on earth are not only unusual, but also fundamental to complex life. In this respect we have not seen anything that would suggest that this is the case. "
See also
- Astrogeology
- Extraterrestrial life
- Extrasolar planet
- Geological history
- Carbon chauvinism
- Cosmochemistry
- Panspermia
literature
- Donald Brownlee, Peter D. Ward: Rare Earth: Why Complex Life is Uncommon in the Universe. Springer Verlag 2000, ISBN 0-387-95289-6 .
- Donald Brownlee, Peter D. Ward: Our Lonely Earth: Why Complex Life is Unlikely in the Universe. Springer Verlag, 2001, ISBN 3-540-41365-0 .
- Milan M. Cirkovic, Robert J. Bradbury: Galactic Gradients, Postbiological Evolution, and the Apparent Failure of SETI. (PDF; 455 kB) In: New Astronomy. Volume 11, pp. 628-639.
- Neil F. Comins: What if the moon didn't exist? Voyages to Earths that might have been . HarperCollins, 1993.
- Simon Conway Morris : Life's Solution . Cambridge Univ. Press, 2003. See chpt. 5; many references.
- Jack Cohen, Ian Stewart : What Does a Martian Look Like: The Science of Extraterrestrial Life. Ebury Press, 2004 2002, ISBN 0-09-187927-2 .
- John G. Cramer: The 'Rare Earth' Hypothesis. In: Analog Science Fiction & Fact Magazine . September 2000.
- Guillermo Gonzalez , Donald Brownlee, Peter Ward: The Galactic Habitable Zone: Galactic Chemical Evolution. In: Icarus. Vol. 152, 2001, pp. 185-200. bibcode : 2001Icar..152..185G
- James F. Kasting , DP Whitmire, RT Reynolds: Habitable zones around main sequence stars. In: Icarus. Volume 101, 1993, pp. 108-128.
- Joseph L. Kirschvink, Robert L. Ripperdan, David A. Evans: Evidence for a Large-Scale Reorganization of Early Cambrian Continental Masses by Inertial Interchange True Polar Wander. In: Science. Volume 277, 1997, pp. 541-545.
- Andrew H. Knoll: Life on a Young Planet: The First Three Billion Years of Evolution on Earth . Princeton Univ. Press, 2003.
- Lewis Dartnell: Life in the Universe, a Beginner's Guide. One World, Oxford 2007.
- Charles H. Lineweaver, Yeshe Fenner, Brad K. Gibson: The Galactic Habitable Zone and the Age Distribution of Complex Life in the Milky Way. In: Science. Volume 303, 2004, pp. 59-62, doi: 10.1126 / science.1092322 .
- Lissauer: How common are habitable planets? In: Nature. Volume 402, 1999, pp. C11-14.
- Nikos Prantzos: On the Galactic Habitable Zone. In: J. Bada u. a. (Ed.): Strategies for Life Detection . To appear in Space Science Reviews . 2006. arxiv : astro-ph / 0612316
- Hugh Ross: Some of the parameters of the galaxy-sun-earth-moon system necessary for advanced life. In: The Creator and the Cosmos. 2nd Edition. NavPress, Colorado Springs CO 1993.
- Stuart Ross Taylor: Destiny or Chance: Our Solar System and Its Place in the Cosmos . Cambridge Univ. Press, 1998.
- Frank J. Tipler : Intelligent Life in Cosmology. In: International Journal of Astrobiology. Volume 2, 2003, pp. 141-148.
- Stephen Webb: Where is Everybody? ( If the universe is teeming with aliens, Where is Everybody ?: Fifty solutions to the Fermi paradox and the problem of extraterrestrial life ) Copernicus Books (Springer Verlag) 2002.
- Paul Davies: The Fifth Miracle: The Search for the Origin and Meaning of Life. Touchstone Pr, 2000, ISBN 0-684-86309-X .
- Hansjürg Geiger: In search of life in space. How life is created and where it can be found . Kosmos (Franckh-Kosmos), 2005, ISBN 3-440-10504-0 .
Web links
- Reviews from Rare Earth :
- Athena Andreadis , PhD in Molecular Biology .
- Tal Cohen , PhD student in computer science
- Galactic Habitable Zone . In: Astrobiology Magazine. May 18, 2001.
- Gregg Easterbrook: Are We Alone? In: The Atlantic Monthly . August 1988. Article anticipating the Rare Earth Hypothes
- Stars and Habitable Planets . Solstation.com
- Victor Stenger : The Anthropic Coincidences: A Natural Explanation . In: The Skeptical Intelligencer. Volume 3, No. 3, 1999.
- 'Hundreds of worlds' in Milky Way . BBC
Individual evidence
- ↑ Brownlee and Ward (2000), pp. Xxi-xxiii.
- ↑ a b Stephen Webb: If the Universe Is Teeming with Aliens ... Where Is Everybody? Fifty Solutions to Fermi's Paradox and the Problem of Extraterrestrial Life . 1st edition. Copernicus Books (Springer Verlag), New York 2002, ISBN 0-387-95501-1 .
- ^ Morphology of Our Galaxy's 'Twin'. Spitzer Caltech, accessed December 11, 2015 .
- ↑ Donald Brownlee, Peter D. Ward: Rare Earth. Copernicus, 2000, pp. 27-29.
- ^ Charles H. Lineweaver, Yeshe Fenner, Brad K. Gibson: The Galactic Habitable Zone and the Age Distribution of Complex Life in the Milky Way. In: Science. Volume 303, 2004, pp. 59-62, arxiv : astro-ph / 0401024 .
- ↑ Donald Brownlee, Peter D. Ward: Rare Earth. Copernicus, 2000, p. 32.
- ^ A b Guillermo Gonzalez , Donald Brownlee and Peter Ward: The Galactic Habitable Zone: Galactic Chemical Evolution. In: Icarus. Vol. 152, 2001, pp. 185-200. bibcode : 2001Icar..152..185G
- ↑ How often does the Sun pass through a spiral arm in the Milky Way? ( Memento from January 17, 2015 in the Internet Archive ) Karen Masters, Curious About Astronomy
- ^ Lewis Dartnell: Life in the Universe. One World, Oxford 2007, p. 75.
- ↑ Donald Brownlee, Peter D. Ward: Rare Earth. Copernicus, 2000, pp. 15-33.
- ↑ Patterns of elemental abundances in the solar system ( Memento of October 27, 2009 in the Internet Archive ) (PDF; 4.9 MB)
- ↑ a b joy.chara.gsu.edu The One Hundred Nearest Star Systems, Research Consortium on Nearby Stars.
- ↑ David V. Martin: Populations of planets in multiple star systems. In: Hans J. Deeg, Juan Antonio Belmonte (Eds.): Handbook of Exoplanets. Springer, Cham 2018, doi: 10.1007 / 978-3-319-55333-7_156 ( arxiv : 1802.08693 )
- ^ M. Hart: Habitable Zones Around Main Sequence Stars. In: Icarus. Volume 37, 1979, p. 351.
- ^ RT Reynolds, CP McKay, JF Kasting: Europa, Tidally Heated Oceans, and Habitable Zones Around Giant Planets. In: Advances in Space Research. Volume 7, No. 5, 1987, p. 125.
- ↑ James F. Kasting , DP Whitmire, RT Reynolds: Habitable zones around main sequence stars. In: Icarus. Volume 101, 1993, pp. 108-128.
- ↑ Donald Brownlee, Peter D. Ward: Rare Earth. Copernicus, 2000, p. 18.
- ^ J. Horner, BW Jones: Jupiter - friend or foe? I: the asteroids . In: International Journal of Astrobiology . tape 7 , no. 3 & 4 , 2008, p. 251-261 , doi : 10.1017 / S1473550408004187 , arxiv : 0806.2795 .
- ^ TC Hinse: Chaos and Planet-Particle Dynamics within the Habitable Zone of Extrasolar Planetary Systems (A qualitative numerical stability study). (PDF; 3.9 MB) Niels Bohr Institute, accessed on October 31, 2007 : “Main simulation results observed: [1] The presence of high-order mean-motion resonances for large values of giant planet eccentricity [2] Chaos dominated dynamics within the habitable zone (s) at large values of giant planet mass. "
- ↑ "Once you realize that most of the known extrasolar planets have highly eccentric orbits (like the planets in Upsilon Andromedae), you begin to wonder if there might be something special about our solar system" (UCBerkeley News quoting Extra solar planetary researcher Eric Ford. ) Robert Sanders: Wayward planet knocks extrasolar planets for a loop. April 13, 2005, accessed October 31, 2007 .
- ^ Clark R. Chapman: Impacts on the Earth by asteroids and comets - assessing the hazard. In: Nature. 367, January 6, 1994, pp. 33-40, doi: 10.1038 / 367033a0 , bibcode : 1994Natur.367 ... 33C
- ↑ Lissauer 1999, as summarized by Conway Morris 2003: 92; see also Comins 1993.
- ↑ Planet-hunters set for big bounty , BBC
- ^ Taylor 1998.
- ↑ Dartnell, pp. 69-70.
- ↑ A formal description of the hypothesis can be found in: Richard Lathe: Fast tidal cycling and the origin of life . In: Icarus . tape 168 , no. 1 , March 2004, p. 18–22 , doi : 10.1016 / j.icarus.2003.10.018 (Quote: “Tidal cycling, resembling the polymerase chain reaction (PCR) mechanism, could only replicate and amplify DNA-like polymers. This mechanism suggests constraints on the evolution of extra-terrestrial life. "). Less formalized in: James Schombert: Origin of Life. University of Oregon, accessed October 31, 2007 : "with the vastness of the Earth's oceans it is statistically very improbable that these early proteins would ever link up. The solution is that the huge tides from the Moon produced inland tidal pools, which would fill and evaporate on a regular basis to produce high concentrations of amino acids ” .
- ^ New Map Provides More Evidence Mars Once Like Earth. December 10, 2005, accessed February 2, 2011 .
- ^ E. Belbruno, J. Richard Gott III: Where Did The Moon Come From? In: The Astronomical Journal . tape 129 , no. 3 , 2005, p. 1724–1745 , doi : 10.1086 / 427539 , arxiv : astro-ph / 0405372 .
- ↑ Stuart Ross Taylor: Destiny or Chance: Our Solar System and Its Place in the Cosmos . Cambridge University Press, Cambridge, England, 1998.
- ↑ Joseph L. Kirschvink, Robert L. Ripperdan, David A. Evans: Evidence for a Large-Scale Reorganization of Early Cambrian Continental Masses by Inertial Interchange True Polar Wander. In: Science , July 25, 1997, Vol. 277, No. 5325, pp. 541-545.
- ↑ Donald Brownlee and Peter D. Ward: Rare Earth. Copernicus, 2000, pp. 144-147.
- ↑ Cramer (2000)
- ↑ Donald Brownlee and Peter D. Ward: Rare Earth. Copernicus, 2000, pp. 271-275.
- ↑ Stuart Ross Taylor: Destiny or Chance: Our Solar System and Its Place in the Cosmos . Cambridge Univ. Press, 1998.
- ^ Simon Conway Morris : Life's Solution . Cambridge Univ. Press, 2003. See Chapter 5; many references.
- ^ Jean Schneider: Interactive Extra-solar Planets Catalog. In: The Extrasolar Planets Encyclopedia. Retrieved February 2, 2011 .
- ↑ Galaxy may be full of 'Earths,' alien life
- ↑ For a detailed criticism of the rare earth hypothesis on this topic, see Cohen and Ian Stewart (2002).
- ^ David Darling: Life Everywhere: The Maverick Science of Astrobiology . Basic Books / Perseus, 2001.