History of Engineering

The history of engineering goes back several thousand years. The engineering sciences emerged as institutionalized sciences in France and Germany in the 18th century.
In the early high cultures of Mesopotamia about 6000 years ago, the first engineers were trained as civil servants at temple and palace schools. The first sciences arose in ancient Greece, including mechanics , which is a basic discipline for many engineering sciences as technical mechanics . A large part of the technical knowledge was passed on through various branches of the handicraft that specialized in the course of time. The ancient general blacksmith became sword, pan or farrier. In the Middle Ages , the guilds also established institutions that dealt with the technical and technical knowledge.
Technology flourished in the Renaissance : Engineers like Leonardo da Vinci studied books on mechanics and created many new machines. From around 1550 they began to write technical literature. In the 17th and 18th centuries, the natural sciences increasingly turned to technology: technology was understood as an applied natural science. Schools for engineers have been established in France, including the École polytechnique . In the 19th century, many engineering schools were founded in German-speaking countries. Some of them were upgraded to technical universities, were given the right to award doctorates around 1900 and were thus scientifically equal to universities.
In the 20th century, numerous new disciplines emerged - mostly specializations in existing areas or at interfaces between existing areas. At the same time, there was an increased process of scientification, which was reinforced by computer-aided technologies such as CAD , CIM , CNC or the finite element method .
Research field and delimitation
The term science has two important meanings:
- On the one hand, as the amount of all knowledge, in the engineering sciences, knowledge of technology and
- on the other hand as a social system, in the engineering sciences that means the engineers, research institutes, technical universities.
A history of engineering therefore deals with the state of knowledge about technology in different epochs and with the various researchers and research institutions.
A comprehensive presentation also includes various influences by and on engineering in various other areas. The greatest interactions are with technology itself, which has often been improved by engineers. On the other hand, new technology has often also driven the development of science. For example, automobiles gave rise to the scientific discipline of vehicle technology . But the political, philosophical, social or economic environment also had a positive and sometimes inhibiting effect on the engineering sciences and were in turn influenced by them. The history of engineering has numerous links with the history of natural sciences ; in particular in the 17th, 18th and partly also in the 19th century, technology was understood as applied natural science and the natural sciences themselves understood themselves as application-oriented science. The areas of mechanics, thermodynamics and electrical engineering that emerged at that time are still being worked on by engineers and natural scientists today. From around the middle of the 19th century, they increasingly went their separate ways: The natural sciences dealt with less technically important areas such as quantum mechanics or the theory of relativity and emphasized much more the theory before application. This was increasingly emphasized in engineering.
More precise details on the individual engineering disciplines are left to the corresponding article. Here, the comprehensive history of all or at least the most important disciplines is presented.
Early civilizations
People had their first technical experience as early as the Stone Age. In anthropology, the manufacture of tools is even seen as a distinguishing feature between humans and animals. In this context there is talk of Homo Faber , of “people who make tools”. The first tools were initially simple hand axes , later special tools for drilling, sawing, scraping, fishing or hunting. The beginnings of production technology go back to the beginnings of mankind. In the Neolithic Revolution , people moved from hunting and gathering to farming and raising livestock. The first high cultures of the Hittites , Assyrians , Babylonians and Persians emerged in Mesopotamia .
Technology in Mesopotamia and Egypt

Copper mines were operated in tunnels that were already 20 m underground. The copper ore was then melted into copper in blast furnaces and alloyed with tin to form bronze , which gave the Bronze Age its name. This made basic metallurgy processes known. The wagon wheel , the potter's wheel and the plow were also invented.
In the first big cities, roads and bridges were built, aqueducts were built for the water supply and huge representative buildings were built, such as the palaces of Persepolis or the Egyptian pyramids . Ramps and cranes were used to build them. Applications of the incline and the winch were already known.
In the military sector, siege devices were used to conquer cities that were already surrounded by walls, such as the proverbial walls of Babylon . These were carefully planned and maintained by master builders, as we know from existing building plans. Among other things, they contained scales and dimensions, the condition of the walls and substructures as well as the inner and outer shells.
The origin of the technology is not yet ascribed to a scientific penetration of the world. In many traditional stories, inventions such as the hook plow or writing are sometimes ascribed to so-called cultural heroes, but ultimately they always go back to divine advice and plan. The god Enki , for example, is considered to be an ingenious and pragmatic inventor.
Reputation from craftsmen and engineers

The ancient oriental kings often presented themselves as exemplary engineers throughout the entire epoch. In inscriptions on large buildings they boast of their technical expertise, but also praise the commitment of the workers, who, according to the inscriptions, gave them rich gifts. Many images of the kings show them as basket carriers or carrying bricks. Such a high reputation for physical work and technical ability is in contrast to the later Greco-Roman disdain for the same.
Training of engineers
Engineers were trained in cuneiform reading and writing and arithmetic at palace and temple schools . Other areas were mathematics and measurement technology . The inclination of water channels, the excavation of excavation work, the weight and loading capacity of walls and the inclination of ramps were calculated. The state-sponsored education lasted for several years and the graduates then worked in the state as high-ranking administrative officials. There was still no separation between the military and civil areas: engineers who planned palaces, bridges or canals in peacetime and supervised their construction held high command positions in wartime.
science
The ancient oriental cultures had already developed a high level in astronomy and mathematics . The latter was characterized by practical problems, such as the calculation of areas for dividing arable land after flooding of the Nile or determining the base area of structures. If the exact formulas were not known, one was satisfied with approximate solutions, which is also typical in modern engineering. For the area of the circle, for example, A = (8/9 d) ² with d as the diameter, which gives the value 3.1605 for the number of circles , while the exact value is 3.141 ... It was only in ancient Greece that theory and practice were separated.
Antiquity
In ancient Greece and Rome , iron processing was taken over by the Hittites. In contrast to copper, which also occurs naturally in metallic form, iron must first be melted from ores. The new material gave the Iron Age its name and was used for tools and weapons because it is harder and stronger. However, iron could only be forged with great force while hot and not yet cast and therefore only gradually displaced bronze. Many large structures such as temples and the Colosseum were erected throughout ancient times . The Romans built numerous roads, bridges and aqueducts . Important technical inventions were the pulley block for cranes, the Archimedean screw for draining mines, the gearwheel and the screw . The ancient society was shaped by agriculture and slave economy; Machines were rarely used. The sciences flourished, especially mathematics, mechanics , astronomy and philosophy . Up until modern times, however, they only had an interest in pure knowledge. The implementation in technology or other applications were considered to outsmart nature.
Technology in antiquity
During the Greco-Roman period, technology developed relatively slowly, which was mostly limited to improving the details of known technologies. There were no major increases in productivity as in the early advanced civilizations by wheel and plow, nor was there any development of new energy sources. Water wheels were known, but were not used extensively until the Middle Ages. The reason is the widespread slave labor, which was cheaper than machines, as well as the resulting disinterest of science in practice.
From the archaic period of Greece (before 500 BC) many small bronze statuettes have been preserved that were still made with full casting. An important innovation was the hollow casting , in which the inside of the workpiece is hollow and thus saves material. Larger bronze statues were cast this way . Helmets and armor were cold from a single piece of bronze with a hammer driven . Iron, on the other hand, could only be forged in a glowing state, but not yet cast because the furnaces had not yet reached the required temperature of around 1500 ° C. Roman mines reached depths of around 200 m and were drained with Archimedean screws that were operated by slaves.
The ancient architects already wrote books about the construction of their works and the difficulties encountered. Although none of these books has survived, Vitruvius and Pliny collected them and evaluated them themselves in preserved books. The Romans paid special attention to their infrastructure and built many kilometers of canals and roads, as well as numerous bridges, aqueducts and ports. They also developed the construction with round arches and vaults and were thus able to span larger rooms. The load-bearing capacity could not yet be calculated, but the buildings show that there was a basic understanding of the force processes and relationships.
The water supply which was ensured by aqueducts played a special role. The skills of the civil engineers can be reconstructed through the tunnel of the Eupalinos , which channeled water from near Samos into the city. The tunnel is completely underground and was driven from both sides. When one encountered impenetrable rock, the planned route had to be abandoned. In order to ensure that the two tunnels met again, extensive knowledge of the geometry was required.
In the Greek theater numerous machines were used to move figures or to change the scenery. Since they gave the viewer the impression that they would move by themselves, they were called automata . The catapult was invented in the military and improved through systematic experiments. In construction, cranes with pulley blocks and running wheels were used . The first watermills appeared at the end of antiquity .
Craft and economy
The ancient economy was characterized by agriculture and slavery . Craftsmen were partly free, partly also slaves. Machines were only used to a limited extent. A number of different professions are attested to in antiquity, representing an early form of division of labor and specialization. For example, there were blacksmiths who specialized in gold, silver, tools or sickles.
In early antiquity, the handicraft was still a relatively high priority. Odysseus , for example, is proud that he made his marriage bed himself. In the 4th century BC However, physical labor quickly loses its reputation. Labor is left to the slaves and strangers and is underestimated. Both the Greeks Plato and Aristotle and the Romans Cicero and Seneca despise wage labor because it is carried out in dependence on others. Politics , but also philosophy , literature and science are considered to be the social justification for liberation from physical labor .

Mythologically, the origin of technology is traced back to Prometheus , who taught people all of the techné . For this he was tied to a rock by Zeus as a punishment until Heracles freed him again. David S. Landes's much-cited work The unbound Prometheus on the Industrial Revolution alludes to this.
The terms technique , technology go back to the Greek techne , which originally meant "to weave, to weave", but soon for all kinds of human creativity. The activities of a carpenter or blacksmith were called techne as well as that of a doctor; Even mathematical reasoning or physical love was so called and sometimes translated as art : craftsmanship, medical art or evidence. In this sense it was understood as the opposite of natural . Technology was something that humans "artificially" created. Via the Latin ars, as in the septem artes liberales, the seven liberal arts, or the Artes mechanicae, the mechanical arts, it made its way into modern times. Here, too, the German “art” was often used in the sense of modern “technology”, for example in terms such as hydraulic engineering, wind art (building windmills) or artist engineer .
Aristotle classified the various techne between empirical knowledge and scientific knowledge. An architect is on a higher level than a craftsman because the latter work only on the basis of experience, while the former can indicate the cause of their work. This was the first time Aristotle made a distinction between builders and craftsmen.
mechanics


The engineering mechanics is that discipline is the result of the scientific nature of technology. The Greek word mechané originally meant “cunning” or “auxiliary”. With the help of a mechané , you typically get out of an emergency. When Odysseus is caught by the Cyclops , Odysseus points his club and blinds him with what is known as mechané . Likewise when the blacksmith god Hephaestus catches Ares with the help of chains. From the 5th century BC The term is used to describe all types of machines, such as cranes or the automatons in the theater. However, the term never lost its original meaning as “cunning” and always shimmered through a little when a machine was used: nature is being outwitted.
This contrast between nature on the one hand and artificially created machines on the other goes back to Plato and Aristotle and determined scientific understanding for the next two millennia. According to Aristotle, every body has a “natural” place to which it strives from an “inner” drive: air upwards, earth downwards. On the other hand, there are the "artificial" or "violent" movements of the machines that are imposed on a body from outside. When a small weight lifts a large one with a machine, nature is outwitted. A stone to which an external force is communicated by a thrower, however, follows this compulsion only as long as this artificial impulse continues directly or indirectly. Then it behaves spontaneously again according to its nature, i.e. falls vertically downwards with constant speed. Aristotle's explanations were essentially retained in the Middle Ages and were expanded into the impetus theory . It was only Galileo Galilei that found the correct mathematical description of free fall : with increasing speed and constant acceleration.
Ktesibios built a water organ , an air pump and a water meter and is therefore considered to be the founder of hydraulics . Philo of Byzantium wrote a partially preserved work on these subjects. Heron built the Heronsball , a ball that could rotate by steam power. However, like most machines, it was viewed more as a toy; the energy was not used to do work. He also wrote a work on mechanics in which he describes the simple machines: lever , winch , wedge , roller and screw . Two applications are specified for the latter: In connection with a pin that slides back and forth in the thread or together with a gear. A screw nut is not mentioned.
The most important mechanic, who was sometimes way ahead of his time, is Archimedes . He is said to have invented the lever balance, the winch with reduction and the Archimedean screw , inventions that were actually used. For the first time he correctly formulated the law of levers and also established hydrostatics , according to legend, when he checked whether a crown is made of pure gold by determining its water displacement.
The ancient mechanics had successfully laid the foundations of a new science that was only surpassed in modern times. Little was new, however: levers and roles, for example, had been known for a long time but had not been scientifically analyzed or described mathematically. The great achievement of antiquity lies in the fact that it was able to trace complex machines back to a few and above all simple machines and thereby explain them.
Science and philosophy

The Greeks were the first people to do science at all. They mostly strived for pure gain in knowledge and achieved great achievements in mathematics, philosophy and mechanics. Implementation in practical applications was rare - the understanding of technology as an applied natural science is of modern origin. In ancient times there was a great contrast between the natural environment and the artificially created technology. A transfer of scientific knowledge seemed impossible and there was a separation between theory and practice.
The scientific areas are basically structured differently: All areas for which a mathematical description is available are assigned to mathematics. In addition to arithmetic and geometry, this also applies to music, astronomy and mechanics, which is now a branch of physics . Physics itself, also known as natural philosophy in ancient times , is not mathematized and deals with all areas that deal with the environment. The movement theory of Aristotle belongs to this area. Plato and Aristotle had a great influence on the ancient understanding of the world. The first divided the world into an area above the moon and one below it. In the sky the planets move with mathematical precision on circular orbits, on the other hand a straight-line movement prevails that cannot be described mathematically. Plato's theory of ideas , which he illustrates in the famous allegory of the cave , also had a great influence . His distinction between ideas, on the one hand, and the actually perceptible material world, on the other, hampered the advancement of the natural and technical sciences, but was very fruitful in mathematics: the Greeks rearranged the traditional mathematical knowledge and axiomatized it. This enabled them, for example, to prove the Pythagorean theorem - which had long been known - by reasoning.
The ancient ideas were to be dominant for the next two millennia. They were carried through Neoplatonism into the Christian Middle Ages, preserved and mostly only critically questioned in modern times. Newton finally overcame the contrast between heaven and earth by tracing both the movement of the planets and the fall of an apple on earth to gravity. However, Galileo only found the laws of fall because he abstracted from disturbing influences, simply removed them in his mind and thus arrived at the phenomenon that is called an idea in the Platonic sense.
middle Ages
In the Middle Ages, many were monasteries , castles and cathedrals built. Military technology also improved - in addition to the castles, above all in the field of catapults and tribocks . The wind and water mills , known since late antiquity, spread throughout Europe and became an important source of energy. They often drove flour mills, but also hammer mills and other machines. The mill builders were experts in the field of mechanics and were important in the development of mechanical engineering.
Technology in the Middle Ages


In the Middle Ages there was a slow but continuous development of technology.
The invention of the collar increased the horse's usable pulling power many times over. Horseshoes and stirrups favored the emergence of powerful knight armies . Plowing wheels and three-field farming increased labor productivity in agriculture. Iron processing also made progress: wires were now drawn and no longer laboriously twisted, the furnaces reached the temperatures required for the production of cast iron . With annealing , carburizing and refining processes were available that influence the carbon content of iron and thus hardness, strength, forgeability and castability. However, these processes were explained by the “purifying power of fire”. It was not until the 18th century that it was found that the carbon content explains the differences between the various ferrous materials.
In the early Middle Ages, it was mainly monasteries that were expanded, and later the cities. The discovery of pointed arches , ribbed vaults and buttresses , which better transmit the forces, made the Gothic style possible with large windows. A famous building, for example, is the Notre-Dame Cathedral . From a military point of view, the castles were of great importance and changed the system of warfare: there were hardly any major field battles, the siege was predominant. For this purpose, the ancient catapults were further developed into a tribock .
With the new windmills and the water mills known from antiquity but rarely used , two new sources of energy were developed that could replace muscle power. They spread rapidly across Europe and were mainly used to drive flour mills, but also to drain mines or to drive machines or hammer mills. They are of great importance for the development of mechanical engineering: The mill builders were wandering craftsmen who knew a lot about mechanical problems and transmission elements such as gears or shafts. They are considered to be the forerunners of modern machine builders. The expansion of the mills themselves also only reached its peak in modern times; in Germany only in 1880, long after the invention of the steam engine.
Crafts, guilds and economy
Through the abolition of slavery, favored by Christianity, the work had a higher reputation again. In the early Middle Ages, the monasteries were mainly devoted to work and prayer. Work eventually became a calling to be called by God. This reputation also favored some technical developments: horseshoes and collars , for example, were technically quite simple innovations, but they had a great effect on labor productivity.
From the 12th century onwards, the importance of cities increased. This is where the guilds were formed, which paid attention to the quality of the products and the training of the crafts. They are thus an early form of institutionalized knowledge. The division of labor slowly increased: the ancient armorsmith became an armorer and armor polisher. In many trades there were technical innovations that made work easier, including the spinning wheel and the rocker lathe, which, in contrast to the older pull-cord lathe, could be operated alone.
Engineers and builders were mostly experienced craftsmen - there was no formal training yet. In documents of the 11th century, the Latin name ingeniator appears for the first time , which did not exist before. It goes back to ingenium (spirit, sharp intellect) and migrated to the Romance languages until the late Middle Ages. B. French Ingénieur or Italian Ingegnere . In German, however, they have long been referred to as "builders" or "work masters".
Science, philosophy and religion
The ancient ideas migrated via Neoplatonism into early Christianity, where they were reinterpreted by the early church fathers such as Augustine . In particular, God was given much more consideration. For example, he created both heaven and earth. So if order can be found in the movement of the planets in the sky, this also applies to the area of the earth. The technology in the form of the seven mechanical arts was considered to be given by God to compensate for the shortcomings caused by the expulsion from paradise. They consisted of textile processing, handicrafts, sea and land trade, gardening and agriculture as well as agriculture, food industry, medicine and jousting .
From the 12th century onwards, numerous universities were founded in Europe where the septem artes , the seven liberal arts, were taught. It was the trivium of grammar , rhetoric and dialectics or logic and the quadrivium of arithmetic , geometry , music and astronomy . Her studies prepared for a subsequent study of medicine, theology or jurisprudence . Since the universities were shaped by Christianity and the humanities, it was difficult to establish the natural sciences in modern times. Instead, new academies were founded specifically for the natural sciences, which was to be repeated in the 19th century with the engineering sciences.
Renaissance
During the Renaissance , Leonardo da Vinci designed a large number of machines, some of which were far ahead of their time. From the middle of the 16th century, the so-called machine books were created , in which engineers addressed princes in Latin, but often also to their colleagues in living languages. Educated engineers also turned to the rediscovered ancient writings on mechanics and used their findings. In the 17th and 18th centuries, scholars and scientists turned more to practical problems. Many areas of physics, especially mechanics, have now been mathematically developed. Galileo Galilei , for example, dealt with the laws of the case and found a mathematical formulation. It happened more and more often that scientific findings could be converted into technical innovations. In the 18th century, in particular in France, institutions for engineers were created for the first time: First, the military engineers were grouped into corps , later numerous special schools for artillery, fortress construction, mining and road and bridge construction were founded. In 1794 the Ecole Polytechnique was founded, which heralded the turning point for the scientification of engineering sciences during industrialization.
Technology in the Renaissance

The old castles with their high but thin walls offered little protection against the new cannons . The new fortresses were built flat and with thick walls; Their special aesthetics can only be seen from a bird's eye view or on the construction plans: they often had a star-shaped floor plan, the conception of which required extensive knowledge of geometry and surveying. Still, they were primarily functional. They had no blind spots and withstood the fire. Fortress construction developed into an independent field of knowledge. The gunsmiths who worked with artillery knew how to make cannons, how to straighten them , how to maintain them and how to handle gunpowder. The princes often asked for recipes to be written down so that they could be preserved in the event that the gunsmith fell in battle. The artillery also became an independent field of knowledge. Among other things, scientists dealt with the question of the mathematical description of the bullet's trajectory.
The mechanical wheel clocks built by precision mechanics have been continuously developed; and its accuracy increased from a deviation of 15 minutes per day to 15 seconds. They were considered the epitome of the then modern technology and have also been studied by scientists. Huygens was able to show that the period of oscillation of a pendulum is independent of the deflection if it moves on a cycloid and was also able to build a clock that implemented this.
Two important innovations were Gutenberg's papermaking and movable type letterpress printing . Both made it possible to print numerous books that a large part of the population could afford. In the beginning, Luther's Bible was printed very often; from around 1550 onwards, numerous technical and scientific books were added.
Two structures are of particular importance for civil engineering . The Florence Cathedral was almost finished at the beginning of the 15th century. The only thing missing was the dome, which seemed impracticable because of the huge diameter of 45 meters at the time. There was no way to erect a falsework in the required dimensions. However, Brunelleschi found out through theoretical considerations that he would be able to build the dome without scaffolding if it were given an elliptical shape. This already showed a slow transition from the builders' experience to theoretical knowledge on the part of the engineers. The turning point for the civil engineering brought the renovation of St. Peter's Basilica in 1742. Here you familiar the first time on the calculations of mathematicians on basics of mechanics, as they consider the incorporation of further pull rings as reinforcement for the dilapidated building to be sufficient. The suggestion of the experienced builder to remove the entire dome was rejected.
There were design drawings and ideas for a whole range of machines, but these were not or could not be implemented in practice. These include many machines by Leonardo da Vinci , the turbine and forerunner of the steam engine . Machines were no longer rated only according to their basic functionality, but also according to their efficiency. Many attempts have been made to build a perpetual motion machine . It was not until 1775 that it was possible to show that such a machine basically does not exist.
Crafts, manufactories and economy
The economy was still largely determined by handicrafts and guilds. The latter now had an inhibiting effect on technical development. There were fears of job losses and innovations were forbidden. In many cases the guilds had the state on their side.
The state also had a great influence on the economy thanks to the mercantilism that was prevalent in continental Europe . An attempt was made to improve the state budget in the long term by promoting trade. For this purpose, infrastructure measures such as the construction of roads, bridges and canals were used to facilitate trade, schools for building technology were founded and scientists were commissioned to solve practical problems. These should publish their results and thus directly promote the economy. In mining , which was of great economic importance, the problem of drainage was urgent. The increasing importance of this problem motivated scientists to deal with hydrostatics and dynamics. Great efforts were made to carry out the drainage mechanically, which after many failures led to a steam-driven pump in 1698 and to the first piston steam engine in 1712. Schools were established in the 18th century to train French engineers who were commissioned by the state to take care of mining, road and bridge construction, fortresses and artillery.
With the manufacture , a new type of production facility developed. There was a high degree of division of labor in them, which led to higher productivity. The example of the English economist Adam Smith , who describes the manufacture of pins, is famous . By breaking down production into individual work steps - cutting off the wire, sharpening it, putting on the head - the quantity produced could be increased many times over. The new production method was only worthwhile if there was a correspondingly high demand for standardized, i.e. identical, products. Since the military had such a need for weapons, ammunition, uniforms and other equipment, the state itself often set up new factories. It was not until the beginning of the 19th century that a corresponding engineering school was founded with the Ecole Centrale des Arts et Manufactures (e.g. Central School for Technology and Manufactures).
The precision engineering was a new branch of industry, which dealt with the manufacture of clocks and measuring instruments, mostly consisted of brass, which is easier to handle than iron, but at the same precise machines allowed as the use of wood. The precision mechanics also built the first machine tools that were specially designed for precision. Their lathes, for example, had tool holders that could be moved using cranks. Blacksmiths could only work iron relatively imprecisely, but could produce larger workpieces. Locksmiths dealt with the finishing of iron parts, which was usually done by filing. These trades then worked together in industrialization and thus founded industrial mechanical engineering , which ultimately led to the engineering discipline of mechanical engineering . Since scientists needed measuring devices more often, the instrument makers came into contact with them more often and thus with modern research. The need to use mathematics to build the instruments themselves reinforced this exchange of experiences. The precision mechanic James Watt was to be commissioned in the 18th century at the University of Glasgow to repair a model of the atmospheric steam engine according to Thomas Newcomen . In doing so, he found a decisive improvement that helped the steam engine to break through.
Renaissance engineers

The engineers of the Renaissance are usually referred to as " artist engineers ". As a rule, they come from the trade, but they already had a very good knowledge of scientific areas, which they acquired through self-study. This includes in particular various mathematical areas, such as metrology, geometry or mechanics. The more educated among them also read the ancient works of Vitruvius, Heron or Archimedes. Most worked at princely courts and thus came into contact with nobles and scholars and commuted between them and the craft practice. Leonardo da Vinci is a typical representative . Due to the new requirements of technology, they noticed that the old traditional knowledge was no longer sufficient and began to independently research the scientific foundations of their specialist areas. Da Vinci made, for example, material-technical investigations and also some experiments. On the one hand, they used the methods of scholars, but on the other hand they set themselves apart from them by emphasizing that practical knowledge and experience are essential. The Italian war engineer Buonaiuto Lorini emphasized that mathematically exact surfaces or lines do not exist in practice. Here lies the difference between the theoretical mathematician and the practical "mechanic".
From around 1550, technical specialist literature spread. While previously the specialist knowledge was passed on personally, a formalization and canonicalization of the knowledge of an engineer has now developed. In his book Die Ingenieurs Schul of 1630, Faulhaber dealt with geometry, topography, geography, trigonometry, logarithms and arithmetic, as well as optics, music, architecture, which was already differentiated into military and civil construction, astronomy and mechanics. These areas were often still counted as mathematics. In the mathematical textbook by Christian Wolff in 1710, in addition to today's mathematical areas, artillery, hydrostatics, perspective drawing and chronology were treated.
The technical literature also had an impact on the self-image of the engineers: For the first time they saw themselves as a group and developed a sense of their class. Previously, the areas of activity were too different and personal acquaintances were more important than belonging to an abstract group. In their books they always emphasized their own intellectual activity, which is based on science, and thus set themselves apart from the craft. They also stressed that they would create new things and act for the common good.
In the 17th century, the military engineers who were involved in the construction of fortresses or artillery were grouped into independent corps. This was the first time there was an institutionalization, as well as a separation between military and civil construction. The training initially took place within the corps, but several schools were soon established. France was the pioneer of this development.
Technical literature
After the rediscovery of ancient writings by the humanists, the old technical works by Archimedes, Heron, Vitruv or Pliny were also reissued and read by interested engineers in particular. They also began to write books themselves. In the 14th and 15th centuries, they were hardly widespread and also very expensive. From the middle of the 16th century, they spread rapidly. Some of them addressed noble readers and described fancy mechanisms which, because of their unnecessary complexity and enormous dimensions, would hardly have been functional. Others are aimed at engineers or the general public as readers. They were written in the respective national languages - i.e. not in Latin - and contained numerous illustrations. The functionality was explained with the accompanying texts. However, it was not a matter of instructions for replicating. In addition, dimensions, material specifications or the gear ratios of gearboxes were missing. However, the engineers noted this information very meticulously in their private files, as is known from numerous preserved estates. This reluctance was intended to protect the knowledge capital of engineers: They wanted to prevent their personal knowledge from becoming general knowledge. The motivation for writing the books was based on the desire for social recognition and prestige. They also sent some books as recommendations to princes if they wanted to offer themselves as engineers. Gradually these works, often referred to as machine or mill books, became more and more precise. One went over to the newly discovered central perspective, from around 1700 onwards one found dimensioned floor plans and elevations as parallel projection . In addition, economic aspects began to be taken into account, especially efficiency. Most of the authors described the state of the art at that time and in some cases also added new knowledge and began to use scientific theories. It was then possible to build on this systematically organized knowledge in the 19th century and promote the scientificization of engineering.
The work Delle Fortificationi of Lorini treated forts and cannons. Alberti wrote De re architectura in 1452 . The work deals with architecture and construction. He largely follows ancient authors such as Vitruvius, but also gives new things such as a theory of dome construction or mathematical rules for vault construction, which are, however, still very simple. In 1540 Biringuccio published his multi-volume De la Pirotechnia , which deals with metallurgy, the manufacture of cannons and fireworks, i.e. everything that has to do with fire in the broadest sense. The ten-volume De re Metallica about mining by Georg Agricola , published posthumously in 1556, stands out in particular. Numerous machines and processes are described very precisely and depicted realistically. In 1569 Besson wrote Theatrum instrumentarum et machinarum , the first modern machine book. In Branca La machine 1629 is the first picture of a turbine contained. It was not until the end of the 19th century that technology had progressed sufficiently to be able to build functional examples. The work Schauplatz der Maschinen by Jakob Leupold from 1727 is considered the last major machine book before industrialization. It contains numerous factual, sober illustrations which, thanks to their dimensions, allow the machines to be reproduced. In his architecture hydraulique from 1753, Bélidor already combined theoretical and practical knowledge and used the new infinitesimal calculus to solve hydraulic problems.
Cannon boring machine in dimensioned parallel projection from the Encylopédie by Diderot , 18th century.
philosophy
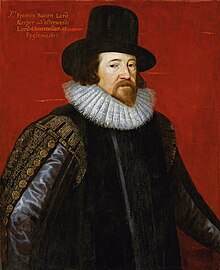
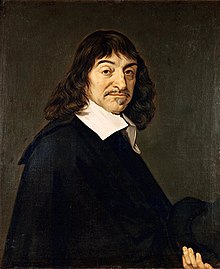
Francis Bacon and René Descartes are of particular importance for understanding science and technology and their relationship to one another .
The English statesman Bacon attacked the predominant science of the scholastics in his novel Novum Organum . They cited ancient authors such as Aristotle or Ptolemy, the founder of the geocentric worldview, and accepted them as authorities. Based on the statements they accepted as true, further statements were obtained through logical inference. This method is known as deduction . Bacon opposed the induction propagated by him . Based on concrete observations of nature and experiments one should come to new knowledge. The truths would be in the things themselves and not in any books. The underlying philosophical theoretical approach is therefore called empiricism (from Latin empiricus , following experience). Thus the previous method of the craftsmen, who always thought from experience, was taken up in science and the separation of theory and practice was eliminated. The saying “knowledge is power” also comes from Bacon, expressly referring to scientific knowledge. It should then be used for human benefit. The Royal Society , founded in 1660, explicitly referred to Bacon in its statutes and advocated research into the natural sciences through experiments and the promotion of industry.
The French philosopher, mathematician and natural scientist Descartes, on the other hand, was an advocate of the mathematical-deductive method and rationalism . He thereby favored the mathematization of the natural sciences. The mechanical conception of nature, which looks at the world like clockwork, also comes from him. Nature and technology no longer form a contradiction, which is why it was now also possible to use technology to learn something about nature. Various measuring devices, telescopes in astronomy and microscopes in biology were now used. By using technology, humans can make themselves users and masters of nature. Natural science was practiced to make natural processes usable for humans and to convert the new knowledge into technical progress. Many scientists were therefore guided in their research by problems in technical practice.
The German polymath Leibniz made “theory and practice” his motto and gave it to the Society of Sciences he founded . This clearly shows the predominant claim of science to solve practical problems.
Science in the Renaissance

The mathematization of physics was started by Galileo. Based on theoretical considerations, he was able to show that with constant acceleration, the distance covered by a freely falling object is proportional to the square of the time. He then checked the laws of fall obtained in this way through experiments. In addition to free fall, he also examined the trajectory of thrown objects, which he correctly identified as parabolas . This also provided a mathematical description of bullet trajectories. Galileo, however, abstracted from disturbing and difficult to describe influences such as air resistance and swirl. In addition to the statics known since antiquity, he set the new kinematics , the theory of movements that do not take forces or acceleration into account. This takes into account the dynamics and the kinetics , which were established by Newton through his axioms named after him and the law of gravitation. Through the latter, the movement of the planets around the sun and the fall of an apple on earth were traced back to the same cause and the Platonic opposition between heaven and earth was finally overcome.
To describe the various dynamic problems, Newton and Leibniz developed the infinitesimal calculus, an area that is used today in many technical fields. The acceleration of a body can be represented as a derivative of the speed, for example. Basically, most mathematicians understood their discipline to be clearly application-oriented and operated it very pragmatically. The aim was to make rapid progress and only secure the terms with exact definitions to the extent that was just necessary. Even Euler made differentials zero.
Euler was active in numerous theoretical and practical areas of mathematics and mechanics. He translated the book Principles of Artillery and added extensive comments. His turbine theory was way ahead of its time and exceeded the mathematical knowledge of the engineers of the time. However, it was so complete that an engineer around 1900 could use it without any problems. Euler also developed a new theory of ships , which brought innovations for the first time since Archimedes' hydrostatics. However, in his theory he underestimated the interaction between ship and water. In the field of hydrodynamics he also contributed new things and applied infinitesimal calculus here as well. His treatise on optics was immediately put into practice in the construction of improved lenses. He also dealt with buckling problems and the description of a beam clamped on one side, a standard problem of strength theory that was already formulated by Galileo. Hooke was also active in the field of strength theory , who described the elongation of a wire as proportional to the mechanical tension. The connection was named in his honor as Hooke's law .
Personalities like Torricelli and Pascal worked in the field of pneumatics . Otto von Guericke found out that the atmospheric pressure can do work. His experiment with the Magdeburg hemispheres became famous . At first there were no applications, but Thomas Newcomen built on Guericke's new findings when he built the first functioning steam engine, in which negative pressure is generated by condensation of water and the resulting vacuum lets the ambient pressure do the work.
For a long time there were no mathematical descriptions for objects of investigation such as light , heat , electricity and magnetism . These phenomena have been investigated through numerous experiments and are therefore classified as part of the so-called “Baconian sciences”. For a long time, it was debated whether light was a wave, as Huygens' experiments suggested, or particles, as suggested by Newton's. Today this contradiction has been overcome with the wave-particle dualism . Research into these areas established the physical fields of thermodynamics (thermodynamics) and electrostatics , from which the engineering disciplines of technical thermodynamics and electrical engineering emerged in the 19th century.
Although at the beginning of the Renaissance one hoped for a direct advance in technology through scientific research, this was rarely the case, especially in areas that were particularly urgent, such as the drainage of mines. Nevertheless, important theoretical foundations were laid here on which engineering sciences could later build. These application problems had various causes. On the one hand, the engineers and craftsmen lacked the necessary knowledge of mathematics and Latin as the language of science to be able to understand the new findings. On the other hand, the scientists often lacked awareness of what is technically feasible at all. Their idealizations, such as the abstraction of friction, air resistance or material constants, on the one hand made a first mathematical treatment possible in the first place, but on the other hand made it difficult to apply it.
Academies
The new natural sciences could hardly establish themselves at the old universities because they were too much attached to the old scholastic tradition and the influence of the church inhibited scientific research. For this reason, numerous new academies were founded in the 17th and 18th centuries that were dedicated to research and often referred to bacon.
In England, therefore, some scholars, merchants, technicians and nobles joined together in 1660 to form the Royal Society . Despite the name ( Royal Society ), it was an association of private individuals that was financed by membership fees and donations. According to Hooke, one of her secretaries, she wanted to "increase the knowledge of natural things and all useful trades, manufactures, mechanical practices and inventions through experimentation." The Society published its own journal, Philosophical Transactions . About half of your contributions dealt with basic scientific research and topics from the commercial-technical area. Papin, Savory and Newcomen published here, for example, their innovations regarding the heat engines. After Newton took over the presidency in 1703, the technical contributions decreased rapidly, one concentrated on the natural sciences. The Society of Arts, founded in 1754, then devoted itself to technology .
In the German-speaking area, Leibniz founded the Society of Sciences in 1700 , and he assumed its chairmanship. Leibniz's motto “theory and practice” became their motto, which was also reflected in the new member Jakob Leupold . He was an engineer and had an apprenticeship as a craftsman as well as a dropped out mathematics degree.
In 1666 the French Minister Colbert founded the Académie des Sciences on the model of the English Society in order to promote the trade. Their scientists were paid by the state and could normally devote themselves to their research at their own discretion. Since these were mostly dedicated to basic research, rapid progress in the trades was largely absent. Sometimes the scientists were entrusted with special tasks by the state, such as surveying France or testing machines and devices. The Académie was supposed to decide whether they were new and useful inventions. Gallon published all 377 submitted inventions in 6 volumes and wanted to promote their application.
Two publications by the Académie deserve special mention as large companies. The Descriptions des arts et métiers (description of techniques and trades) by Bignon and Réaumur and the Encyklopadie des sciences, des arts et des métiers (encyclopedia of natural sciences, techniques and trades) by Diderot and d'Alembert .
The "Descriptions" were based on an order from Colbert to examine the existing trades and factories, to prepare precise drawings and process descriptions and to promote the economy through their publication. Work began in 1695 and was only finished after Réaumur's death. Between 1761 and 1789 121 volumes with over 1000 copperplate engravings were published. Réaumur himself was active as a scientist in many fields and invented the temperature scale named after him and a process for making improved steel, which he published. Even so, it didn't catch on, possibly because the hut owners couldn't read.
The "Encyclopedia" was published between 1751 and 1780 in 21 volumes and over 3100 copperplate engravings and was supposed to represent the entire knowledge of mankind. A large part was devoted to technology. In contrast to the “Descriptions”, the “Encyclopedia” went beyond the mere representation of the technology and embedded it in the overall social context. Many famous scientists and scholars, like Voltaire, could be won as authors. There were many critical voices in it that ultimately contributed to the French Revolution .
Relationship between science and practice
Science and practice, i.e. their application, are in a diverse relationship to one another. During the Renaissance, craftsmen and engineers began to study scientific theories, and scientists wanted to solve practical problems, but they were not always successful. Euler was asked to plan the water supply for the water features and fountains in Sansouci. The system was built according to his calculations, but did not deliver a drop of water. Leibniz tried to build a windmill with a vertical shaft in the Harz Mountains, but was not able to explain his plans to the local craftsmen. According to modern studies, the construction was actually functional. When the French King Louis XIV needed a system for the water games in Versailles that would pump water up a 162 m high mountain in nearby Marly , the responsible minister Colbert turned to the Académie des Sciences. Huygens and Papin suggested using gunpowder to generate the necessary energy. The experiments in the presence of the minister showed that this idea was not very promising. He eventually went public and hired the carpenter Rennequin Sualem to build it. He had already built a similar system, but could neither read nor write. The Marly water lifting system consisted of 14 water wheels and 221 pumps and was considered a technical masterpiece that attracted many visitors.
The further development of steam engines gives a good insight into the relationship between theory and practice at that time. Papin made the first attempts to move a piston with steam, but could not get beyond an experimental apparatus. The first working steam engine was the pump of the English engineer Savery , which he called "The miner's friend" (The miner's friend). However, under the difficult conditions of mining, it was hardly usable and did not prevail. It was not until the English blacksmith Newcomen built a usable piston steam engine that was used to pump out pit water in mining, but was only poorly energy efficient.
Nevertheless, there was also a strong belief in science among engineers and practitioners. Many of them were convinced of the correctness of the Galilean trajectory parabola , although it could not be confirmed in practical tests with cannons. Here played swirl and drag a significant role, so two sizes, one of which Galileo had abstracted. In Germany, the long-barreled cannons also prevailed for a long time, as their superiority has been scientifically proven, although the short-barreled Swedish guns clearly provided the better results.
The Hessen-Grossmann thesis by Boris Hessen and Henryk Grossmann states that theoretical mechanics did not emerge from theory, but through occupation with the machines available at the time, especially those that were used in production, as well as clocks. Hessen also formulated the reverse of his thesis: Because there were still no steam engines and electrical machines (electric motor or generator), thermodynamics and electricity theory remained at a very modest level. Important scientific breakthroughs only occurred when the respective machines were already in place. Grossmann also claims an indirect economic influence on the development of mechanics: While in antiquity the desire to expand production led to a desire for more available slaves, in the late Middle Ages and early modern times the same desire led to improved means of production. The desire for low transport costs also led, among other things, to efforts to reduce the drag of ships. It was only here that the scientifically formulable question about the optimal geometry of the ship arose and was answered by hydromechanics.
Formalization of training
year | Surname |
1675 | Corps des engineers du génie militaire |
1716 | Corps des engineers des ponts et chaussées |
from 1720 | several École d'Artillerie |
1747 | École nationale des ponts et chaussées |
1748 | École royale du génie de Mezieres |
1760 | Ecole de la Marine |
1783 | Ecole des Mines |
1794 | École polytechnique |
Because of the prevailing absolutism as a form of government and mercantilism as an economic form, the continental European states had great influence on the economy and the military. Many princes had their territories measured. These include the famous land survey of Bavaria 1554–1568 or the land survey of France. It was carried out by the Paris Akadémie since 1668 in over a century of work. The fortress builder Vauban directed the construction of hundreds of fortresses across France under Finance Minister Colbert in the 17th century . The Canal du Midi should offer new trade routes in the south of France and thus support the economy in order to ultimately improve the state budget. With a length of 240 km and over 200 locks, its construction was the largest construction project since ancient times. For the same reasons, the construction of roads and bridges in France was promoted, which noticeably shortened travel times. Similar construction projects were only carried out on a much smaller scale in German-speaking countries. Since the Thirty Years' War devastated large parts of the country and killed many people, cobblestone streets outside the cities could hardly be afforded. In liberal England, the state hardly interfered in economic affairs and left building projects to private initiatives.
In the 17th century, the artillery engineers of the individual regiments were combined to form the "Corps d'Artillerie". In 1675, Vauban combined the French fortress builders into a separate corps , the Corps des ingénieurs du génie militaire (for example, the corps of military pioneers ), which by 1700 already contained around 300 engineers. They were stationed at the numerous fortresses and took care of administration and maintenance. New fortresses were hardly ever built. Due to the loss of importance of the fortresses in the Napoleonic Wars of Movement, they were increasingly used for road construction and military logistics. Around 1800 their number was around 8,000. The training initially took place within the corps. After an apprenticeship period of one to two years, there were exams that ensured selection.
The civil builders were combined in 1716 to form the Corps des ingénieurs des ponts et chaussées (for example: Corps of road and bridge engineers ). This led to a separation between military and civil engineering. The 20 to 200 engineers were divided into three hierarchical levels and assigned to the individual tax districts where they planned new construction projects.
Various regiments of the artillery initially set up schools for the training of the next generation temporarily and from 1720 onwards. This was the first time in the world that the technology was taught in schools and the gap between technical experience and literary-scientific education began to close. The lessons contained both theoretical elements such as mathematics and drawing, as well as practical elements. Some of the lessons also took place in the field. Many bourgeois and petty aristocrats used these schools for a career in the military, as here and with the fortress builders more attention was paid to personal suitability than in the rest of the military, where aristocratic origins and letters of recommendation were more important. Napoleon , for example, graduated from an artillery school. Around 1800 the number of artillery engineers was around 1000 due to the high demand in the Napoleonic Wars.
In 1747, the École nationale des ponts et chaussées ( Bridge and Road School ) was the first school for civil civil engineers to be established in order to standardize the training of engineers. The duration of the lessons was not exactly fixed and was between two and seven years. The lessons were varied and included calculus, drawing, cartography, swimming and horse riding. There were also experiments, for example on the strength of building materials. The approximately 500 engineers in the Corps came to a much greater extent from the middle classes than in military engineering.
A year later, the École royale du génie ( Royal School for Military Pioneers ) was founded in Mézières for military engineers. The lessons lasted one to two years and of all schools had the highest mathematical and scientific level.
The Ecole des Mines was founded in 1783 on the model of the German mining academies , which at the time, like all German mining, were considered particularly progressive. Modern processes and techniques were used particularly often here.
In 1794, the École polytechnique was finally founded, which is of particular importance for engineering. On the one hand, it is the last engineering school of the Ancien Régime and has the highest scientific level of these. But it is also the first school of a completely new type. Here, in two years of instruction, the common mathematical and scientific foundations were laid for further studies at one of the special schools for fortress construction, artillery, mining etc. These special schools were now called Ecole d'Application ( application school ). What was really new was not that mathematics and natural sciences were understood as the basis of engineering, but rather the recognition that the various engineering disciplines have a common basis here. This division into basic and application subjects has proven itself in engineering courses up to the 21st century. Following the example of the Ecole Polytechnique, numerous so-called polytechnical schools emerged in the 19th century in German-speaking countries , which eventually became the technical universities .
industrialization
The industrial revolution occurred in England in the middle of the 18th century . Thomas Newcomen built the first working steam engine in 1712, which was decisively improved by James Watt in the second half of the century and which expanded rapidly from around 1800. The new puddling process made it possible to produce steel in large quantities, which was used for the construction of steam engines, textile machines, locomotives and rails as well as machine tools .
In the course of the 18th century, numerous engineering schools were founded in France, which dealt with road and bridge construction, mining, military fortification and artillery, among other things. In 1794 the École polytechnique was founded, in which the common mathematical and scientific fundamentals of the various disciplines were taught. After graduation, graduates attended one of the aforementioned special schools. For the needs of industry, the École Centrale des Arts et Manufactures was founded, which trained for higher positions in companies, and several Ecole des Arts et Métiers , which trained for middle positions (master level).
In order to catch up with the great lead in industrialization over England, numerous so-called polytechnic schools were founded in Germany in the 19th century, which were based on the French Ecole Polytechnique. By 1870 they had already exceeded the level of training in England, which there was technical and practical, but unsystematic. The German polytechnic schools, whose training performance was quickly reflected in the success of the German iron and steel industry and mechanical engineering, were upgraded to higher technical schools (later engineering schools ) and partly to technical universities towards the end of the 19th century . At the turn of the 20th century, the latter were finally given the right to award doctorates and were thus on an equal footing with older universities. Some of them like the TH Charlottenburg developed a Europe-wide broadcast, u. a. to Russia, whose most important trading partner was Germany. Many technical colleges were later converted into universities or technical universities .
technology
At the beginning of industrialization , three types of machines were particularly important for mechanical engineering : First, textile machines such as spinning machines ( spinning jenny ) or weaving machines . Second, the steam engines , including Newcomen's atmospheric piston steam engine and Watt's double-acting piston steam engine. Third, the machine tools , which include lathes, milling, planing, slotting and drilling machines, and machine hammers. They were all invented by so-called tinkerer engineers, most of whom came from the trade, without any special theoretical knowledge or assistance from scientists. Improvements were also made by practitioners: Smeaton systematically varied the dimensions of Newcomen's steam engine and was thus able to increase its efficiency considerably. In mechanical engineering, which emerged in polytechnic schools from around 1800, these machines were then the main area of the new engineering science. Textile and machine tools were part of mechanical technology, a forerunner of manufacturing technology . The steam engines were examined in more detail in technical thermodynamics .
The previously common charcoal was replaced by hard coal , which, however, required new processes in metallurgy and metallurgy due to its contamination . This ultimately resulted in the new puddle iron , crucible cast steel and coal coking, which also come from practitioners. In the course of high industrialization, other processes were added that further improved the quality and cost-effectiveness of steel production: the Bessemer , Thomas and Siemens-Martin processes . A transition towards scientific innovations can be seen here. A large part of the steel was used for bridge and railway construction, which has also been scientifically studied. In machine dynamics , for example, different vibration phenomena on steam locomotives were dealt with .
The tar that fell from hard coal coking aroused the interest of chemists, who founded the new field of tar chemistry and were now able to manufacture paints and medicines from tar . Other chemical innovations, all of which started out from chemistry as a science and quickly found application in the chemical industry, were explosives , fertilizers and plastics . In this context one speaks of a " science-based technology ", a technology that is based on science. This includes in particular the new electrical engineering . Here, new scientific findings were quickly converted into technical innovations. Gauss said: “Science attracts applications like a magnet does iron.” At the beginning of the 19th century, electricity was used for telegraphs , and later also for city lighting. The dynamo-electric principle from Siemens helped achieve the breakthrough in electrical engineering . This made it possible to convert the mechanical energy of steam engines into electrical energy via a generator , to transmit it via lines with almost no loss and to use it again with electric motors . The batteries that were customary up to now became superfluous. From around 1880, the electrical engineering industry became increasingly important. At about the same time, the new engineering field of electrical engineering emerged.
Factories and economy
The new machines brought about an epoch-making transition from hand-tool technology to machine-tool technology, which contemporaries already saw as an industrial revolution . Up-and-coming industries were initially the textile industry, mechanical engineering, mining (coal and ores) and heavy industry. The chemical industry and industrial electrical engineering were added later.
Industrialization began in England , which was several decades ahead of all other countries. It led to a political and economic supremacy in England. In the other European countries, the state therefore endeavored to catch up with the arrears as quickly as possible through economic development. The most important instrument at that time was the establishment of engineering schools and the promotion of science and education . Engineering institutions were almost always founded by the government and not by a civil society.
The scientification of these industries can be exemplified in mechanical engineering. At the beginning of industrialization, it was the existing craft businesses and factories that procured machines and often built them for their own use, thus establishing the first factories . In the second phase, engineers founded new businesses directly as factories - without going through craft businesses. The training of engineers varied from state to state. In England and America it was people who had worked their way up in factories from unskilled workers to foremen and factory managers, through which they had gained a lot of experience. In Germany and France, they were graduates from engineering schools such as Borsig or Maffei . In times of high industrialization , special design offices were set up in which scientifically trained engineers found employment. Around 1900 the production engineers who were entrusted with production began to deal with the scientific basis of their work and with the question of how production could be made more economical. The resulting wave of rationalization culminated in Taylor's “ scientific management ” and led to new departments in the industry in which engineers dealt with work preparation . This also established the engineering field of business administration , a forerunner of manufacturing technology . At around the same time, industrial management , a sub-area of the new business administration , was developed in Germany .
In the last decades of the 19th century, many industrial companies started doing research themselves. Taylor, for example, researched the scientific basis of machining and also invented high-speed steel . However, it was typical for the chemical and electrical engineering industries to have their own research departments, where scientists began to hire scientists.
science
At the beginning of the 19th century, France was still the leading scientific nation. Many famous scientists and mathematicians worked at the École polytechnique , founded in 1794 . Engineering continued to be viewed as an applied science. In England, on the other hand, engineers gained a lot of practical experience with new materials such as steel and concrete , new machines or structures. While most of the scientific knowledge came from France, where Descartes had a great influence, almost all technical innovations came from England. Contemporaries put it more simply: “The French formulate what the English simply do.” Over the course of the century, Germany became the leading scientific nation. In the engineering sciences a mixed system of the practically oriented English system and the mathematical and scientific system from France established itself, with multiple fluctuations between the two extremes. England, France, and the United States began to imitate the German system towards the end of the century.
Technical mechanics
The classical mechanics , as a branch of physics was founded by Galileo and Newton and d'Alembert and Legendre developed. However, the French Coulomb , Navier , Coriolis and Poncelet are considered to be the founders of the actual technical mechanics .
Coulomb was active in the field of structural engineering and dealt with the arching and beam bending theory , the determination of the earth pressure against lining walls and the law of friction named after him . He also solved the Galilean bending problem in 1773. However, his findings have not yet been applied in practice. Only through the new special schools did they get into the training of master builders. Navier managed to combine the various theories and research results into a teaching building. His book on mechanics formed the basis for structural engineering and strength theory . He examined a large number of constructions, extracted from them the properties essential for engineering calculations and gave solutions for the underlying equations. Where he could not find exact solutions, he gave approximate solutions with which one was on the safe side - a typical engineering procedure. Coriolis and Poncelet were active in the field of dynamics and the problems associated with moving machine parts such as those in mills, steam engines or turbines. They introduced the concept of "living force", as the product of mass and the square of the speed, later half of it was used, which exactly corresponds to kinetic energy . This made it possible to determine the efficiency of machines based on theoretical considerations.
By using long steel rods as construction material, so - called half - timbered structures were used more often. However, their calculation was very time-consuming with the conventional methods. Therefore the new field of graphic statics was developed. Here were Culmann ( Culmannsche Straight ), Mohr ( Mohr's circle ) and Cremona ( Cremona diagram ) operates. The example of the framework also shows that it is not the task of the engineering sciences to reinsert certain details from which the natural sciences abstracted in their theories, since otherwise they would become completely inexpedient. Instead, a specific technical abstraction is often necessary. Culmanns performance was that of looking at the individual bars of a truss as a rigid body that articulated in the joints stored are. This considerably simplifies the entire calculation with practicable accuracy.
Technical thermodynamics

In 1824 Sadi Carnot published his work The Moving Power of Fire , in which he emphasized the increasing importance of steam engines and made general considerations about them. He found the ideal cycle process named after him and the conditions for a cycle process with maximum work. Julius Robert Mayer discovered that heat is a special form of energy and also found an equation to convert it. The two main principles of thermodynamics come from Rudolf Clausius . The development of thermodynamics started with the machines that were already in existence, the functionality of which was subsequently examined. It is often expressed in a somewhat simpler way: the steam engine has done more for thermodynamics than the other way around.
Zeuner wrote his book Grundzüge der Wärmetheorie in 1859 , in which he collected the previous individual research results and organized them systematically. The material was prepared for the purposes of engineers and contained numerous tables with which one could assess existing machines or design new ones.
Zeuner's pupil Linde examined the relationship between the heat extracted and the energy used in cooling and ice machines, and found that they were far below the theoretically possible efficiency. On the basis of this knowledge he was able to construct improved machines. In Linde's lectures, Rudolf Diesel heard about the low efficiency of steam engines and tried to implement the ideal cycle process. Although he did not succeed in doing this, the internal combustion engine he built was significantly more efficient than the previous steam engines.
Production engineering
The forerunners of production technology go back to the subject “ technology ”. It was taught at universities in the 18th century as part of courses in camera studies . The students were later to work as administrative officials and received descriptions of the various trades, their tools, machines and processes and were to use this in the sense of good economic development. When the training of civil servants became more and more important in the 19th century, the subject of technology slowly lost importance at universities and moved to polytechnic schools.
In 1815, under the technologists Johann Joseph von Prechtl and Georg Altmütter, the Vienna School separated into mechanical technology ( manufacturing technology ) and chemical technology ( process engineering ). In chemical technology, it was not only about the various chemical reactions and other chemical principles, but also considerations and methods for process control, energy and material transport and the required apparatus and systems . Karl Karmarsch is of particular importance in mechanical technology . He collected the various processes, tools and machines, found their similarities and similarities in critical-comparative works, arranged them systematically in his manual of mechanical technology and thus created the basis of production technology. The current system, however, goes back to Otto Kienzle from the 1960s. From around 1870 onwards, many new processes came up that made independent scientific research necessary. Karl Ernst Hartig , for example, carried out force and work measurements on machine tools and Friedrich Kick made deformation tests.
Materials testing and laboratories
Various broken axles on railways and steam boiler explosions gave rise to material-technical investigations into strength parameters. Wöhler made tests with wheel axles while driving, as well as his fatigue strength tests , which formed the basis for further investigations into various load cases such as static, swelling and changing loads. Bauschinger made measurements of material parameters of all common materials in mechanical engineering and civil engineering and checked whether the idealizations of elasticity theory and strength theory were permissible.
Electrical engineering
Lectures on electrotechnical phenomena such as galvanism and electromagnetism have already been held as part of the natural science subjects at the polytechnic schools . When the importance of electrical engineering increased significantly from around 1880 , Siemens personally set up independent professorships. The first professors were Dietrich in Stuttgart, Kittler in Darmstadt and Slaby in Berlin. Electrical engineering initially formed independent departments within the mechanical engineering faculties; it was only later that separate faculties were founded. The focus was on heavy current engineering with generators and electric motors based on the electrodynamic principle found by Siemens.
Engineering research
In the last third of the 19th century, three different types of research institutes emerged: the technical universities, which dealt with both research and teaching, the private research institutes, which were primarily oriented towards applied research, and state research institutes such as Physikalisch -Technische Reichsanstalt (PTR) or the Kaiser Wilhelm Society , which dealt exclusively with research and mostly carried out basic research, but also, like the PTR, were responsible for industrial funding , standardization or other tasks that neither private industry nor individual universities handle could.
Technical language and clubs
In many countries citizens founded associations to promote the local or national economy and were mainly active in the field of education. They published magazines, promoted the exchange of experiences, put on collections, gave lectures, wrote out prize assignments and often founded schools for the general public, as this area of education was often neglected by the state. Since there were often many tradespeople and ordinary citizens in these associations, they were mostly concerned with a more practice-oriented training.
The development of a technical vocabulary was facilitated by the magazines and associations. Numerous terms emerged from complicated paraphrases: For example, Riegelweg (cf. English Railway), iron art route , iron route and rail route were used for “ track ” . Often the trend went from German compound words ( compound words ) to foreign words, often of Latin origin. The steam car was initially replaced by the English locomotion and later by the French locomotive . The term was then Germanized to today's locomotive .
The engineering associations, on the other hand, were associations of engineers, although at that time it was still unclear who exactly belonged to them. The Association of German Engineers, the most important association, was founded by graduates of the Berlin Trade Academy , but was also open to anyone else who was committed to technology in any way. In England there was the Society of Civil Engineers and the Institution of Civil Engineers for the railway and civil engineers, and the Institution of Mechanical Engineers for the mechanical engineers. In America there were similar associations with the same name. In France, the graduates of the Ecole Polytechnique formed one association and the graduates of the various Ecoles des Arts et Métiers formed another.
England
England was the starting point of the industrial revolution. For a long time, historical research assumed that scientific knowledge did not play a particular role. For a long time, individual people and inventions were at the center of research and the history of technology . Hardly any of the early English engineers had a higher formal education. Thomas Newcomen, the inventor of the first steam engine was a blacksmith, James Watt, who made the decisive improvement, was a precision mechanic. Some did not even have a completed apprenticeship. Modern historical research has put this picture into perspective again: the engineers did not have a formal high level of training, but sufficient scientific and technical knowledge could be acquired even without attending school and also played a greater role than had long been assumed. Newcomen built on the experiments of Papin and Huygens and used Guericke's findings on air pressure. Watt dealt with the properties of water vapor in self-study, in particular the heat of vaporization , which at that time was still called "latent heat", was important here. The engineers of the 18th and 19th centuries found themselves in a society that allowed easy access to scientific theories. The Royal Society published new research in its journal and small communities such as the Lunar Society in Birmingham formed in many cities , where scientists, entrepreneurs, doctors, craftsmen and inventors met and exchanged ideas. In the educated middle class, an ideology of progress spread which was very positive about technology and natural sciences. However, the theoretical knowledge was only available outside the established educational institutions of the churches, schools and universities; the Scottish universities in Glasgow and Edinburgh are an exception.
- Engineers
The engineers were quite capable of gaining urgently needed scientific knowledge themselves if necessary. When Thomas Telford and Robert Stephenson wanted to build steel bridges, they didn't have enough experience with the new material. They therefore carried out the corresponding tests themselves. Their bridges were then the reason to further develop the theory of suspension bridges for science. The experience of the new steamers was that the hold was not big enough to carry enough coal to cross the Atlantic. The great engineer Brunel , who was revered as a national hero and even received a state funeral, pointed out that the drag of a ship depends on its cross-section and thus on the square of its dimensions, while the hold volume depends on the third power. So if you built the ship big enough, he concluded, you can use it to cross the Atlantic.
- Mechanical engineering and shop culture
Specific cultures and levels of knowledge developed in the various mechanical engineering factories and workshops. So the engineers' knowledge mainly depended on the companies in which they had learned. The machine tool manufacturers Maudslay and Whitworth , for example, paid particular attention to precision in their operations and trained numerous engineers. Whitworth, for example, was a student of Maudslay himself. This is why the English and American systems are usually described as “workshop culture” and differentiated from the Franco-German “school culture”.
- education
With industrialization advancing very rapidly, it was believed that the British system was the best possible. When new schools were founded in France and in German-speaking countries for engineers working in industry, this was rather ridiculed in England. For example, Brunel advised a young engineer that he should read the French theoretical books, but that in a few hours in a blacksmith's workshop he would learn a lot more of what he needed. The British lead in industrialization was enormous: more than half of the world's steel production came from English smelters. It was similar in many other important areas. At the first world exhibition in London in 1851, England presented itself as the “workshop of the world”. When the USA and the new German Empire overtook England in the industrialization process from around 1870, English contemporaries primarily blamed the poor formal training of their engineers for this. Modern research was only to a relatively small extent the cause of the German-American upswing and the falling back of England compared to these countries; nevertheless, in response to this, the first schools and courses were established in England.
Rankine became professor of electrical engineering at the University of Glasgow as early as 1850 . In 1868 Jenkins followed in the University of Edinburgh. In the same year a study program for engineers was established at Owen College in Manchester. In 1881 the Central Institution South Kensington and the Finsbury Technical College were founded and eventually merged to form Imperial College .
- societies
In English, the word engineer can mean both engineer and mechanic. In order to be able to differentiate oneself from the latter, various engineering associations were formed in England that only accepted people who had already made a living as engineers. The Society of Civil Engineers , founded by John Smeaton in 1771, emphasized the contrast to the military engineers and was an exclusive circle with relatively few members who were accepted by co-option . In 1818, railway engineers founded the Institution of Civil Engineers , which, because of Telford's influence, had much more to offer its members and was very popular. The Institution of Mechanical Engineers , founded in 1848, was less exclusive . The ironworkers united in the Iron and Steel Institute and the telegraphy engineers in the Institution of Electrical Engineers .
United States
In the USA , as in England, engineers were initially trained primarily in practice. Over time, simple surveyors became surveyors. In contrast to England, however, there was a small number of engineers with good formal training from the start. The first school of great importance to American civil engineering is the Westpoint Military School , founded in 1802. Most of the graduates went into civil service, but some were also involved in building the channels. Many of the teachers at this school had studied at Ecole Polytechnique themselves or were at least familiar with its educational system. In addition, from 1824 there was the Rensselaer Polytechnic Institute in Troy, NY. Mechanical engineers were also trained here. A wave of founding began in 1845 with the School of Engineering at Union College in Schendactady and the Polytechnic Institute in Brooklyn . In 1846 the Lawrence Scientific School followed as a branch of Harvard University and a year later the Sheffield Scientific School at Yale . In 1852 there was an engineering department at the University of Michigan . The first private college for engineers is the Massachusetts Institute of Technology , which enjoys a special international reputation. The Stevens Institute, founded in 1870, is still important for mechanical engineering .
- societies
First of all, local or regional associations were founded. In 1852 the American Society of Civil Engineers was founded for civil engineers, which gained in importance after a reorganization from 1867. Like its English namesake, it was very exclusive. In 1871 the American Institute of Mining Engineers was founded. In fact, however, it was an association of the mine owners . Both associations had little to offer most of the engineers who were employed in companies. This changed in 1880 with the establishment of the American Society of Mechanical Engineers for mechanical engineers and the American Institute of Electrical Engineers .
France
year | Surname |
1803 | Ecole des Arts et Métiers in Châlons-sur-Marne |
1811 | Ecole des Arts et Métiers in Angers |
1829 | Ecole Centrale des Arts et Manufactures |
1843 | Ecole des Arts et Métiers Aix-en-Provence |
1882 | Ecole Municipale de Physique et de Chimie Industrielles |
1894 | École supérieure d'électricité in Paris |
1891 | Ecole des Arts et Métiers in Cluny |
1900 | Ecole des Arts et Métiers in Lille |
1912 | Ecole des Arts et Métiers in Paris |
1925 | Ecole des Arts et Métiers in Strasbourg |
At the beginning of the 19th century, the Ecole Polytechnique was the predominant scientific institution. Almost all new knowledge in engineering came from here. The training was very mathematical and scientific and the graduates then studied for another year at one of the special schools for mining, road and bridge construction, artillery or fortifications. So almost all of them worked in the civil service.
They were too theoretically trained for the private sector, so that new schools were founded on the private side. The various Ecole des Arts et Métiers (for example: School for Technology and Trade) imparted knowledge that consisted half of theory and practical craftsmanship and thus a level that roughly corresponded to that of a master craftsman. The trade schools in Germany had a similar level . The three schools in Châlons-sur-Marne (1803), Angers (1811) and Aix-en Provence (1843) were initially important. From 1829, the Ecole Centrale des Arts et Manufactures (short: Ecole Centrale, roughly: Central School for Technology and Manufactures) provided training for the higher management level in companies . Their students were 20% landowners, 35% large merchants, 7% academic professions and 10% craftsmen. Engineering schools were thus available in France for all skill levels and technical areas.
When electrical engineering and the chemical industry became increasingly important in the second half of the century, special schools were founded: in 1882 the Ecole Municipale de Physique et de Chimie Industrielles and in 1894 the École supérieure d'électricité . In addition, technical institutes were established at the old universities, the most important of which were in Grenoble, Nancy, Toulouse, Lille and Lyon. The state supported the establishment of the institutes, but was not prepared to finance the permanent operation. Therefore, these institutes geared their research and teaching to the needs of the regional industries, which led to financial support and a relatively high degree of practical relevance. According to estimates from 1913, around half of all engineers came from the Ecoles des Arts et Métiers, a quarter from the university institutes and the rest from the Grandes écoles , i.e. the Ecole Polytechnique and the Ecole Centrale. At the beginning of the 20th century, the Ecoles des Arts et Métiers also received the right, initially the better half of their graduates, and soon after all, to officially graduate as engineers .
The civil engineers of the three Ecole des Arts et Métiers and those of the Ecole Centrale merged in 1848 to form the Société des ingénieurs civils and thus leaned on the Society of Civil Engineers by name. In France, however, the various graduate associations were more important than the engineering associations. The graduates of the Ecole Polytechnique, the so-called Polytechniciens, often found employment in industrial research laboratories alongside their civil service. The Centraliciens, the graduates of the Ecole Centrale, were employed in the upper management level of companies and the Gadzarts, the graduates of the Ecole des Arts et Métiers in the middle level. In contrast, in Germany, graduates from technical universities found work in the state and in business.
German-speaking area
In the German-speaking countries, numerous schools were founded in the course of industrialization at the beginning of the 19th century . Technical and trade schools trained for an intermediate level and were about the level of the French Écoles des Arts et Métiers . The so-called polytechnic schools were founded for higher education, which were also based on the Ecole Polytechnique by name, but seldom reached their level. The first was founded in Prague in 1806. However, only the second foundation was of particular importance: the Vienna Polytechnic Institute . In the 1830s and 1840s, the founding in Karlsruhe was followed by a wave of foundings in many of the German small and medium-sized states. The school founded in Zurich in 1855 (today ETH Zurich ) was a role model. Like the universities, it was divided into faculties and employed numerous mathematicians and natural scientists. Until about 1880 the other schools also received the university constitution and were then officially renamed the Technical University . Around 1900 they received the right to award doctorates and were thus formally equal to the older universities
Political, economic and social influences
In the 18th century the power of the emperor in the Holy Roman Empire was low compared to the individual German princes. When the empire dissolved in 1806, a patchwork of sovereign states remained. This fragmentation turned out to be a political weakness, but it turned into an educational strength, as the individual states often established schools and universities in their area, so that numerous training and research opportunities were available for engineers. In 1848 there was a revolution in the German states . The numerous polytechnic schools that had contributed to the rise of the bourgeoisie were now elevated to "citizen universities" and contrasted with the traditional universities. After the unsuccessful revolution, the Association of German Engineers (VDI) was founded in 1856 . The name was a little sensation: there was no "Germany" back then, only individual states such as Prussia or Bavaria. Accordingly, there were previously only regional associations such as the Polytechnic Association in Bavaria .
The Industrial Revolution in Germany came to England several decades late. The technology transfer proved to be difficult because the English skilled workers were subject to an emigration ban and the machines were subject to an export ban. The German states therefore promoted industrial espionage through foreign trips by German engineers or by illegally bringing skilled workers and machines to the continent. Some of the machines were made available to German companies free of charge, but on the condition that anyone interested could show the machine so that they could replicate it. The most important instrument of economic development, however, was the establishment of technical schools and universities.
The 18th century was marked by the Enlightenment and rationalism ; Education was mainly interpreted as vocational training and the practical applicability and usefulness of knowledge was in the foreground. At the beginning of the 19th century, a strong counter-movement emerged with new humanism . Its co-founder Wilhelm von Humboldt criticized the fact that everything is despised that is not useful and opposed the neo-humanist concept of education, according to which true education is human education and is precisely characterized by freedom from purpose.
From around 1870 onwards there were some important changes. The German Empire was founded and the elimination of tariffs and other trade barriers created a large German internal market, which had a favorable effect on economic and industrial development. This, together with new technologies, led to high industrialization in Germany , in which Germany finally changed from an agricultural to an industrial state and, together with the USA, also rose to become the leading industrial nation. The new technologies were no longer imported and copied from England, but developed in Germany. These include the electric motor from Siemens, the internal combustion engines from Otto and Diesel or the Haber-Bosch process for ammonia synthesis. Some companies also rose to become leading global groups, particularly in the chemical and heavy industries. In educational policy, the conflict between engineers and neo-humanists came to a head: It was about equality between the technical universities, secondary schools and upper secondary schools on the one hand and the universities and neo-humanist grammar schools on the other.
schools
Around 1700, in addition to elementary schools , there were also Latin schools in German-speaking countries that prepared for university studies, military academies for a military career and knight academies that were attended by nobles. In the following 200 years numerous types of schools emerged; in many of them science and technology subjects were taught, others tried to keep them out of the class as much as possible. When it came to the question of admission requirements for technical universities, secondary schools also played a major role in making engineering science more scientific.
The numerous schools were then divided into general schools and technical schools that only teach a narrowly defined subject. In addition to technical colleges, they also include commercial and agricultural schools .
Technical schools

Mining has always been one of the most innovative areas. A level of technology was soon reached here that could no longer be mastered without a minimum of theoretical knowledge, such as the operation of steam engines. The mountain schools in which mining, metallurgy , metallurgy and mine separation were taught are therefore among the oldest technical schools . Mathematics, physics, chemistry and drawing were also taught. For the construction industry there were building trade schools , sometimes also called building academies, whose attendance was compulsory for prospective builders.
For the manufacturing industry, numerous trade schools were founded in the 19th century, which differed greatly in terms of training level, teaching concepts and content and which underwent major changes over the course of the century. There were state and private schools, full-time schools, evening or weekend schools. In Prussia, for example, there was a so-called provincial trade school in every administrative district , which was open to 12 to 16 year-old students and lasted three years. The best graduates were then allowed to go to the Berlin trade institute to expand their knowledge. Most went straight into the economy, found work as skilled workers and usually quickly rose to become foremen. In Bavaria there were over 30 similar trade schools, which on the one hand qualified for the master's level and on the other hand made it possible to study at polytechnical schools. Many trade schools continued to raise their level of education and became general or secondary schools towards the end of the 19th century . The industrial institute, on the other hand, was merged with the Berlin Building Academy to form the Technical University. Other trade schools became vocational schools.
Since from around 1870/1880 the trade schools had changed into general education schools and at about the same time the technical universities became more and more theoretical, there was a training gap in the area of intermediate, practically oriented, technical education. This area was closed by the new middle schools from which the technical colleges developed in the 20th century .
General education schools
So-called industrial schools were widespread throughout Europe in the 18th century . In them the boys learned handicrafts, spinning and weaving and the girls learned housekeeping free of charge. In fact, these were companies in which child labor was used. The proceeds were used to finance the schools. From around 1740 secondary schools were founded that offered both theoretical and practical lessons and were therefore close to technical schools. In the 19th century the practical part decreased; More math and science were taught. In the decades before 1800, philanthropists were founded who were entitled to study and therefore taught Latin, but otherwise placed more emphasis on new languages, mathematics and natural sciences.
In the neo-humanist grammar schools, much emphasis was placed on ancient languages (Latin and Ancient Greek), history, literature, art and music, while technology was completely pushed out of the classroom. Contrary to some efforts - including the headmaster of a grammar school and later philosopher Hegel , who considered it superfluous - the natural sciences were able to keep themselves in class, but possibly only because they now emphasized their theoretical interest in knowledge much more than practical application. In Bavaria, in the school plan of 1804, which emphasized technical and natural science subjects, the four-year secondary schools and grammar schools were formally equated. In 1808, the superiority of the new humanistic grammar schools, which henceforth led to the Abitur, was established over the secondary schools, which only led to the so-called "one year old". This included the right to only have to serve one year as a soldier instead of the usual three-year military service. The bourgeois upper class found scientific and technical education now mostly superfluous, partly precisely because it was benefit-oriented. This also hindered the spread of relevant knowledge in the lower social classes.
In the second half of the century, the secondary and commercial schools became upper secondary schools and secondary schools, in which the proportion of mathematics, technology and natural science was higher. The last few decades have been marked by the dispute over the equality between them and the new humanist high schools. At the school conference of 1890 one tried in vain to abolish the upper secondary schools as a "half-measure". At the conference of 1900 their equal status was decided: high schools increased the proportion of science and technology subjects, high schools and high schools expanded the teaching of Latin. At the same time, there were two other important developments in the higher education sector: The technical universities increased the level of entrance to the Abitur and, with the right to award doctorates, were on an equal footing with older universities.
Colleges
year | Name (bold characters were exemplary) |
1806 | Prague |
1815 | Vienna |
1825 | Karlsruhe |
1827 | Munich |
1828 | Dresden |
1829 | Stuttgart |
1831 | Hanover |
1835 | Braunschweig |
1837 | Darmstadt |
1855 | Zurich |
1870 | Aachen |
1879 | Berlin (through the merger of |
Trade institute (1821) and the building academy (1799)) | |
1904 | Danzig |
1910 | Wroclaw |
In the higher education sector at the beginning of the 18th century there were only those universities from the Middle Ages where no scientific-technical research or teaching was practiced. The only exception was the subject of technology , which was taught within the framework of camera studies. Here, however, it was more about a description of the trades, trades and the predominant methods and tools, not about their application or further development. In the second half of the century, three mining academies were founded: Freiberg 1765, Berlin 1770, Clausthal 1775. Sometimes the Bergakademie in Schemnitz in present-day Slovenia is also included because it was controlled by the Habsburgs at the time. In contrast to the mountain schools , they had a very high scientific level and were also a model for the Ecole des Mines in France in 1783 . Although it would theoretically have been possible to add further technical subjects, they remained of no importance for the further course of technical higher education. The Ecole Polytechnique , founded in France in 1794, developed a great influence and was then the express model that the numerous German universities referred to when it was founded in the 19th century. The predominant focus there on natural sciences and especially mathematics, however, was deliberately not imitated. On the one hand, there was a lack of appropriate teaching staff; on the other hand, technology was not only taught as an applied natural science, but also many practical elements were to be integrated into the lessons.
It all started with the Polytechnic School in Prague in 1806 . However, only the second foundation in Vienna in 1815 was significant. The Viennese institute developed a certain charisma on the other German small and medium-sized states. Here one deviated from the Paris model and integrated the actual technical lectures directly into the institute, whose organizational structure with its “departments” was quite comparable to the faculties of the universities. In 1810, around the same time, the humanistic university was founded in Berlin, today's Humboldt University . The third foundation in 1825 was the Polytechnic School in Karlsruhe, which, like the Vienna Institute, had an organization similar to that of the universities and, due to its high scientific level, also served as a role model and triggered a wave of founding: By 1835 polytechnic schools were established in Munich, Augsburg and Nuremberg , Dresden, Stuttgart, Kassel, Hanover, Darmstadt and Braunschweig. It is noticeable here that most of them were in capital cities. Most of them were divided into three main areas: civil engineering, mechanical technology (mechanical engineering) and chemical technology (process engineering). However, their level was somewhere between high school and college. Almost every student was accepted and, corresponding to the rather low level of previous education, arithmetic, reading and general education in history and geography were also taught. In the middle of the century they increased their level of education and the entry requirements, until the end of the century the calculus was an integral part of the course and the Abitur was the ticket to it. However, good experiences had been made with the students at the vocational schools. This increase in the entry requirements resulted exclusively from the engineers' desire for scientific recognition. And it was less important to what level the lessons reached, but what level it began. In addition, attempts were made to stick as far as possible to the already recognized natural sciences and increased the scope and level of education, especially here and in mathematics. Franz Reuleaux and Franz Grashof made particular contributions to this theorization .
The next important establishment took place in Zurich in 1855. It had a university constitution with faculties and a senate. Following their example, it became customary to appoint mathematicians and natural scientists to chairs. In addition, most vocational schools were reorganized to about 1,870 technical colleges and until 1890 also officially Technical College renamed. From 1860 onwards, engineers criticized the fact that the technical universities in Prussia were subordinate to the Ministry of Commerce and were understood as an instrument of economic policy and not to the Ministry of Culture. After a long struggle in the so-called “departmental war”, responsibility finally changed. The next important stage in terms of academic equality was the right to award doctorates, which was reserved for universities. The engineers and professors stylized the conflict as a "question of life"; Critics from the trade and also within the engineering sciences, for whom theorising went too far, spoke of "doctrianism". In the last two decades the practice-oriented trend became stronger and independent technical research and research laboratories set up for this purpose based on the American model were introduced. This and the increased economic importance of technology for the German Empire prompted the Kaiser to personally end the dispute in 1899 and to give the Prussian technical universities the right to acquire the two academic degrees Diplomingenieur (Dipl.-Ing.) And Doctoral engineer ( Dr .-Ing. ). The spelling with a hyphen and in German was intended as an admission to the demands of the universities, to better distinguish their degrees (Dr. med., Dr. phil, i.e. without a hyphen). The engineers themselves felt that the right to award doctorates, with which at least formally equality was achieved, was the “accolade of science”.
societies
There are three larger groups among the technically oriented associations: The polytechnical associations are the oldest and were mainly formed in the 18th century. Their importance declined from the middle of the following century. The trade associations were associations of traders and came into being in the decades around 1800. The last group is formed by the engineering associations , which consist of two groups: the technical-scientific associations that deal with a specific subject and the professional associations that deal with the professional situation of the Engineers should improve. Some were also active in both fields.
The polytechnical associations arose under the influence of the Enlightenment and often saw themselves as patriotic citizens' initiatives that wanted to promote local trades through technical education, which were under considerable competitive pressure from England and France. In addition, they published club magazines and also had an impact through teaching collections, lectures, prize assignments and exhibitions. Since there was as yet no state supervision of schools, they founded schools themselves - with a tendency towards secondary and commercial schools - and had considerable influence on the curricula. Members were mostly intellectuals: representatives of the administration and university members. Nobles were not found among them, apart from the nobility of merit, and also not the tradespeople as actually affected, since the membership fees were too high. Only a few industrialists were members. The most important representatives include the Association for the Advancement of Industry in Prussia , the Polytechnic Association in Bavaria and the Hamburg Society for the Advancement of the Arts and Useful Crafts .
The technical-scientific associations emerged around individual subject areas. The local and regional associations of architects and engineers from 1824 are among the earliest. They all organized conferences, gave lectures, wrote books, published magazines and were active in the field of standards and guidelines. They also ran training programs for their members. In some cases they have been assigned monitoring and auditing tasks by the state. From these activities later the technical inspection associations emerged, better known as TÜV . The most important and largest representatives are the Association of German Engineers (VDI), which wanted to represent all technical disciplines and thereby set itself apart from the older associations. In fact, mechanical engineering and civil engineering were represented here, as well as in some cases iron and steel industry. The steelworkers joined forces to form the Association of German Ironworkers (VdEh), which also briefly joined forces with the VDI. The numerous electrical engineering associations (ETV) came together in the Association of German Electrical Engineers (VDE).
The professional associations wanted to improve the situation for engineers. Some pursued a class policy for selected groups, such as the engineers who worked in the public service. Others tried to represent the engineers as a whole. They saw themselves discriminated from several sides: the nobility occupied all important positions in politics and the military, the lawyers were predominant in the administration, the educated bourgeoisie represented the cultural superiority of humanistic (non-technical) education and the merchants occupied all important positions in the Management of companies. Although technology and industry became increasingly important for Germany's economic and military strength, engineers as a social group hardly benefited from them for a long time. The most important representatives include the Association of Consulting Engineers (VBI), the Association of German Architects (BDA), the Association of German Graduated Engineers (VDDI), the Association of Technical-Industrial Officials (ButiB) and the German Association of Technicians (DTV) .
20th century
technology
In the 20th century technology developed more rapidly than before; With household and entertainment technology , it also penetrated new areas of life.
In the construction industry there was a move to the use of concrete and reinforced concrete. The buildings grew larger and larger and sometimes significantly exceeded those of their predecessors. Examples are the Three Gorges Dam or the Stuttgart TV Tower . Since one could only fall back to a limited extent on empirical knowledge, a scientific penetration and calculation of the constructions became more and more important.
In mechanical engineering, sewing machines and bicycles were produced at the beginning of the century, but cars, airplanes and finally rockets were soon added. Due to their great importance, the scientific fields of automotive engineering and aerospace engineering developed from this . In the case of prime movers, the trend was from machines with discontinuous back and forth motion, such as piston steam engines and piston engines, to machines with continuous, mostly circular motion, such as jet engines , gas, steam or water turbines , which are more efficient .
The turbines were used in power plants and could thus provide much larger amounts of energy that was not only used for electrical lighting, but also in industry to drive machines. The core technology that emerged from a scientific basic research, meant for the power engineering , which has become a new field, another milestone. In the last few decades the trend has been towards renewable energies such as solar or wind energy . This resulted in energy and environmental technology .
In control and regulation technology, electronic tubes were developed and - through basic scientific research - finally the smaller, lighter and more reliable transistors and diodes . This made it possible to largely automate industrial production and also to build powerful and affordable computers .
The computer itself is not only a product of engineering (and other fields), but also has an effect on it through its applications. In the second half of the 20th century it became common to use computers to design ( CAD ), produce ( CIM , CNC ), or perform complex calculations ( FEM ).
areas of expertise
As early as the end of the 19th century, the number of technical subjects began to grow faster and faster. In 1900 there were around 350. Since then, many more have been added, partly through spin-offs of sub-areas: The machine tools that were part of mechanical technology, for example, became an independent research and teaching area in 1904. Other areas emerged as interface disciplines. At the beginning of the century, mechanical engineers and chemists still worked together in the chemical industry. The special requirements placed on the apparatus (chemical resistance of boilers, pipelines) which should work safely under extreme conditions such as high pressure and temperature, gave rise to the new field of chemical engineering . There are flowing transitions from process engineering and bioprocess engineering to biotechnology .
Many originally purely mechanical machines, such as machine tools or cars, have been increasingly automated by microelectronics . Since knowledge from both specialist areas was required more and more frequently for construction and maintenance, mechatronics originated in Japan . Other interdisciplinary subject areas are computational linguistics and medical technology . The industrial engineering on the other hand is an interdisciplinary degree is between business and one or more engineering.
Control engineering and systems theory have the peculiarity that they neither go back to a natural science, such as technical mechanics or electrical engineering, nor to technology that exists in practice such as vehicle technology, but rather emerged from theoretical engineering research.
Industry, engineers and business
From around 1900 the number of engineers rose rapidly: in Germany before 1914 it was between 100,000 and 150,000, in the 1930s it was 200,000 to 250,000, around 1980 it was around 370,000 (Germany) and in 2000 around 650,000. In 1980 there were around 500,000 engineers in the GDR as defined there. Towards the end of the 19th century there were changes in the engineering profession, which became more and more evident in the 20th century. Independent design offices were established in the larger companies. The construction was thus finally separated from the production, where the foreman and foreman were initially in charge. By Frederick Winslow Taylor's so-called " Scientific Management " or Taylorism were then called operations offices established in which engineers were planning the production: they accepted the design documents, checked them for their feasibility, cared if necessary new tools and means of production and then gave orders to the Workshops continue. The resulting work preparation was also scientifically examined. To this end, new companies were founded in Germany, such as the Reichskuratorium für Wirtschaftlichkeit (RKW), the Committee for Economic Manufacturing (AWF) or the Reichs Committee for Labor Studies ( Refa ).
Taylor was the most prominent member of the so-called rationalization movement, which was about reducing the effort in industrial companies or increasing the yield. An important instrument was in addition to the work studies by Taylor, Gilbreth and Gantt, the standardization and standardization enables the higher volume and lower unit costs. Initially, larger companies introduced their own in-house standards , but could not agree within the industries. With the outbreak of the First World War and the war economy that went with it, these house norms were replaced by military guidelines. From this, the German industrial standards (DIN) arose in Germany . After the war, the engineering associations were particularly active in the field of standardization.
During the world economic crisis between the world wars there was very high unemployment. One even spoke of "misrationalization"; many engineers themselves were also unemployed. The technocracy movement was popular among some engineers in the 1920s and 1930s, especially in the USA and Germany: the economic and political elite had failed to run the country and the increasingly technical environment dictated that engineers should be given the lead. Even smaller associations were formed that formulated this as a political demand. Most of the engineers, however, were extremely apolitical. Since the beginning of the Second World War, the technocratic movement was no longer important.
research
The engineering research was continuously expanded. In the USA, private research institutes such as the Battelle Institute or the Mellon Institute and industrial research laboratories were involved. In Germany it was still the technical universities, the state research companies such as the Physikalisch-Technische Reichsanstalt or the Kaiser Wilhelm Society and also industrial research laboratories.
In the USA from 1945 the model of the “Assembly Line” of the assembly line or assembly line production established itself as a research concept. In the beginning there was basic research. Your results should lead to commercialization through applied research, design and production. The concept was inspired by the experience with nuclear weapons technology. A linear sequence from the discovery of nuclear fission to the operational weapon could be seen here. From the 1960s onwards, there were critical tones about the concept of the assembly line and the importance of basic research. Studies have shown that basic research accounts for less than one percent of technical innovation and that its results are practically not noticeable within 20 years.
Towards the end of the century at the latest, the boundary between basic research and applied research began to blur more and more, so that the distinction only plays a minor role.
Germany
In Germany, there were some peculiarities because of the developments in the 19th century and the events that followed.
From 1900 the Abitur became a prerequisite for studying at the technical universities and the study itself was the normal case for engineers; Skilled workers who rose to become engineers via the foreman level were the exception. Most viewed themselves as apolitical experts, but some also favored technocratic opinions. From this, for example, the Reichsbund deutscher Technik (RDT) and the German Association of Technical and Scientific Associations (DVT) emerged, which functioned as umbrella organizations for technology. After much deliberation, the VDI decided not to join. The National Socialists were the only party to found their own engineering organization, the Kampfbund Deutscher Ingenieure und Techniker (KDAI) with around 2,000 members. In 1937, after the "seizure of power", around 21,000 engineers had a party book of the NSDAP, that is, one in ten. Compared to other academics, they were underrepresented. After 1933, many technical colleges became self-aligned: They implemented the Nazi racial policy, pushed Jewish members out of the colleges, and gave themselves constitutions based on the Führer principle. The well-known mechanical engineering professor Georg Schlesinger was arrested in 1933 on "adventurous charges". When he was released the following year, he took the opportunity to emigrate to England. Other engineers even rose to the top management of the Nazi state: Fritz Todt, was initially responsible for road affairs, later even became a minister and was personally subordinate to Hitler. Albert Speer was his successor after his death in 1942. After the war he was sentenced to prison at the Nuremberg Trials. In the post-war period, many engineers emphasized the value neutrality of technology and shifted responsibility for its use to politics and society.
The 1970 enacted engineering law regulated the use of the term "engineer". Graduates of the technical universities, which have meanwhile been renamed as Technical University or University, were allowed to call themselves "Diplom-Ingenieur (univ.)", With the optional addition of brackets. The graduates of the technical colleges, on the other hand, “Diplom-Ingenieur (FH)” with mandatory brackets. As a result of the Bologna reform, both degrees were replaced by the Anglo-Saxon Bachelor / Master system. In 2000, the universities of applied sciences renamed themselves “university of applied science”, thereby underlining their claim to be scientific, just like the technical universities in the 19th century.
Research history
The Göttingen professor of economics and technology Johann Beckmann is considered the first technology historian today . He recorded the state of the art in his works, but also wrote the book Contributions to the History of Inventions . In the 19th century the engineers began to deal with the history of their own field. Important engineering historians were Friedrich Klemm and Conrad Matschoß , some of whose works are still considered standard works today. However, they wrote more of a mere string of significant inventions and personalities. Technological-historical approaches can also be found in Marx and Engels , but they pay more attention to political and social framework conditions. From around 1960, the history of technology became a historical subject that was researched by studied historians and no longer by engineers. A major exception arose when the SED in the GDR placed an order with the Dresden University of Technology, which was supposed to train engineers to become lecturers in the history of technology. The history of technical sciences , edited by Rolf Sonnemann and Gisela Buchheim , emerged from the following studies in 1990, the first and so far the only complete work. In addition, there have been some presentations since 1990 that deal in detail with the history of individual disciplines such as electrical engineering. A detailed overview of the history of the technical universities is also missing. For many individual universities, however, there are detailed works that deal with them in detail and that were often published for anniversaries.
In addition, since the 1990s philosophy of science and philosophy began not only to deal with the natural sciences, as in the 20th century, but also to include engineering and thus contribute to a clarification of the internal structure of technical knowledge.
literature
-
Armin Hermann , Wilhelm Dettmering (Hrsg.): Technology and culture. VDI publishing house, Düsseldorf.
- Volume 3: Armin Herrmann, Charlotte Schönbeck (ed.): Technology and science. 1991.
- Volume 5: Laetitia Boehm , Charlotte Schönbeck (ed.): Technology and education. 1989.
- Walter Kaiser , Wolfgang König (ed.): History of the engineer - a profession in six millennia. Carl Hanser Verlag, Munich / Vienna 2006, ISBN 3-446-40484-8 .
- Wolfgang König (Hrsg.): Propylaea history of technology. Propylaea, Berlin 1997.
- Volume 1: Dieter Hägermann , Helmuth Schneider : Agriculture and Crafts - 750 BC. until 1000 AD
- Volume 2: Karl-Heinz Ludwig , Volker Schmidtchen : Metals and Power - 1000 to 1600.
- Volume 3: Akos Paulinyi , Ulrich Troitzsch : Mechanization and Machinization - 1600 to 1840.
- Volume 4: Wolfgang König, Wolfhard Weber : Networks, Steel and Electricity - 1840–1914.
- Volume 5: Hans-Joachim Braun , Walter Kaiser: Energy industry, automation, information - since 1914.
Individual evidence
- ^ Sabine Maasen, Mario Kaiser, Martin Reinhart, Barbara Sutter (eds.): Handbuch Wissenschaftssoziologie. Springer, Wiesbaden 2012, p. 111; acatech (Ed.): Technical Sciences. Recognize - create - take responsibility. acatech impuls, Springer, Heidelberg / Berlin 2013, p. 17.
- ^ Karl H. Metz: Origins of technology. Schöningh, Paderborn 2006, p. 25.
- ↑ Ariel M. Bagg, Eva Cancik-Kirschbaum : Technical Experts in Early High Cultures: The Old Orient. In: Walter Kaiser, Wolfgang König (ed.): History of the engineer. Carl Hanser Verlag, Munich / Vienna 2006, pp. 14–17, 19–21.
- ↑ Ariel M. Bagg, Eva Cancik-Kirschbaum: Technical Experts in Early High Cultures: The Old Orient. In: Walter Kaiser, Wolfgang König (ed.): History of the engineer. Carl Hanser Verlag, Munich / Vienna 2006, p. 29.
- ↑ Ariel M. Bagg, Eva Cancik-Kirschbaum: Technical Experts in Early High Cultures: The Old Orient. In: Walter Kaiser, Wolfgang König (ed.): History of the engineer. Carl Hanser Verlag, Munich / Vienna 2006, p. 11f.
- ↑ Ariel M. Bagg, Eva Cancik-Kirschbaum: Technical Experts in Early High Cultures: The Old Orient. In: Walter Kaiser, Wolfgang König (ed.): History of the engineer. Carl Hanser Verlag, Munich / Vienna 2006, p. 10f.
- ↑ Ariel M. Bagg, Eva Cancik-Kirschbaum: Technical Experts in Early High Cultures: The Old Orient. In: Walter Kaiser, Wolfgang König (ed.): History of the engineer. Carl Hanser Verlag, Munich / Vienna 2006, p. 9f.
- ↑ Christoph Scriba, Bertram Maurer: Technology and Mathematics. In: Armin Herrmann, Charlotte Schönbeck (Hrsg.): Technology and science. VDI-Verlag, Düsseldorf 1991, pp. 32-36.
- ^ Karl H. Metz: Origins of technology. Schöningh, Paderborn 2006, p. 39.
- ↑ Dieter Hägermann, Helmuth Schneider: Propylaea history of technology. Volume 1, Propylaen, Berlin 1997, pp. 142f., 150
- ↑ Kurt Maul: Technical Knowledge in Antiquity and the Middle Ages. In: Armin Herrmann, Charlotte Schönbeck (Hrsg.): Technology and science. VDI-Verlag, Düsseldorf 1991, p. 382.
- ↑ Ariel M. Bagg, Eva Cancik-Kirschbaum: Technical Experts in Early High Cultures: The Old Orient. In: Walter Kaiser, Wolfgang König (ed.): History of the engineer. Carl Hanser Verlag, Munich / Vienna 2006, p. 40f.
- ↑ Günter Spur: On the change in the industrial world through machine tools. Carl Hanser Verlag, Munich / Vienna 1991, p. 58.
- ^ Karl H. Metz: Origins of technology. Schöningh, Paderborn 2006, p. 30.
- ↑ Günter Spur: On the change in the industrial world through machine tools. Carl Hanser Verlag, Munich / Vienna 1991, p. 49.
- ↑ Alois Huning: The concept of technology. In: Friedrich Rapp (Ed.): Technology and Philosophy. (= Technology and culture. Volume 1). VDI-Verlag, Düsseldorf 1990, p. 11f.
- ↑ Dieter Hägermann, Helmuth Schneider: Propylaea history of technology. Volume 1, Propylaen, Berlin 1997, pp. 176f.
- ↑ Alois Huning: The philosophical tradition. In: Friedrich Rapp (Ed.): Technology and Philosophy. VDI-Verlag, Düsseldorf 1990, p. 28.
- ↑ Kurt Maul Technical Knowledge in Antiquity and the Middle Ages. In: Armin Herrmann, Charlotte Schönbeck (Hrsg.): Technology and science. VDI-Verlag, Düsseldorf 1991, p. 383.
- ↑ Dieter Hägermann, Helmuth Schneider: Propylaea history of technology. Volume 1, Propylaea, Berlin 1997, pp. 181f.
- ^ Fritz Kraft: Technology and Natural Sciences in Antiquity and the Middle Ages. In: Armin Herrmann, Charlotte Schönbeck (Hrsg.): Technology and science. VDI-Verlag, Düsseldorf 1991, pp. 225-230.
- ^ Fritz Kraft: Technology and Natural Sciences in Antiquity and the Middle Ages. In: Armin Herrmann, Charlotte Schönbeck (Hrsg.): Technology and science. VDI-Verlag, Düsseldorf 1991, pp. 382-385.
- ^ Fritz Kraft: Technology and Natural Sciences in Antiquity and the Middle Ages. In: Armin Herrmann, Charlotte Schönbeck (Hrsg.): Technology and science. VDI-Verlag, Düsseldorf 1991, pp. 387-389.
- ↑ Karl H. Metz: origins of technology Schöningh, Paderborn 2006, p. 36.
- ^ John Peter Oleson: Engineering and technology in the classical world. Oxford university press, Oxford, New York 2008, p. 5.
- ^ Fritz Kraft: Technology and Natural Sciences in Antiquity and the Middle Ages. In: Armin Herrmann, Charlotte Schönbeck (Hrsg.): Technology and science. VDI-Verlag, Düsseldorf 1991, pp. 220-234.
- ↑ Armin Hermann: What do the natural sciences want? In: Armin Herrmann, Charlotte Schönbeck (Hrsg.): Technology and science. VDI-Verlag, Düsseldorf 1991, p. 212.
- ↑ Marcus Popplow: Insecure Careers: Engineers in the Middle Ages and Early Modern Age 500-1750. In: Walter Kaiser, Wolfgang König (ed.): History of the engineer. Carl Hanser Verlag, Munich / Vienna 2006, p. 80.
- ↑ Marcus Popplow: Insecure Careers: Engineers in the Middle Ages and Early Modern Age 500-1750. In: Walter Kaiser, Wolfgang König (ed.): History of the engineer. Carl Hanser Verlag, Munich / Vienna 2006, p. 72f.
- ^ Karl H. Metz: Origins of technology. Schöningh, Paderborn 2006, pp. 40-48.
- ^ Karl H. Metz: Origins of technology. Schöningh, Paderborn 2006, pp. 40-42, 65-68.
- ↑ Marcus Popplow: Insecure Careers: Engineers in the Middle Ages and Early Modern Age 500-1750. In: Walter Kaiser, Wolfgang König (ed.): History of the engineer. Carl Hanser Verlag, Munich / Vienna 2006, p. 71f.
- ^ Fritz Kraft: Technology and Natural Sciences in Antiquity and the Middle Ages. In: Armin Herrmann, Charlotte Schönbeck (Hrsg.): Technology and science. VDI-Verlag, Düsseldorf 1991, pp. 235-237.
- ↑ Alwin Diemer, Gert König: What is science? In: Armin Herrmann, Charlotte Schönbeck (Hrsg.): Technology and science. VDI-Verlag, Düsseldorf 1991, pp. 15-20.
- ↑ Marcus Popplow: Insecure Careers: Engineers in the Middle Ages and Early Modern Age 500-1750. In: Walter Kaiser, Wolfgang König (ed.): History of the engineer. Carl Hanser Verlag, Munich / Vienna 2006, p. 92f.
- ↑ Christoph Scriba, Bertram Maurer: Technology and Mathematics. In: Armin Herrmann, Charlotte Schönbeck (Hrsg.): Technology and science. VDI-Verlag, Düsseldorf 1991, p. 52.
- ^ Karl H. Metz: Origins of technology. Schöningh, Paderborn 2006, pp. 48-52.
- ^ Karl H. Metz: Origins of technology. Schöningh, Paderborn 2006, pp. 61-65, 86-89.
- ^ Charlotte Schönbeck: Renaissance - natural sciences and technology between tradition and new beginnings. In: Armin Herrmann, Charlotte Schönbeck (Hrsg.): Technology and science. VDI-Verlag, Düsseldorf 1991, p. 252.
- ↑ Christoph Scriba, Bertram Maurer: Technology and Mathematics. In: Armin Herrmann, Charlotte Schönbeck (Hrsg.): Technology and science. VDI-Verlag, Düsseldorf 1991, p. 58.
- ^ Karl H. Metz: Origins of technology. Schöningh, Paderborn 2006, pp. 91-94.
- ↑ Karl H. Metz: origins of technology Schöningh, Paderborn 2006, p. 83f.
- ↑ Christoph Scriba, Bertram Maurer: Technology and Mathematics. In: Armin Herrmann, Charlotte Schönbeck (Hrsg.): Technology and science. VDI-Verlag, Düsseldorf 1991, p. 47.
- ↑ Marcus Popplow: Insecure Careers: Engineers in the Middle Ages and Early Modern Age 500-1750. In: Walter Kaiser, Wolfgang König (ed.): History of the engineer. Carl Hanser Verlag, Munich / Vienna 2006, p. 89.
- ^ Charlotte Schönbeck: Renaissance - natural sciences and technology between tradition and new beginnings. In: Armin Herrmann, Charlotte Schönbeck (Hrsg.): Technology and science. VDI-Verlag, Düsseldorf 1991, p. 258.
- ↑ Gerhard Bronner purpose: Technical Science in the industrialization process to the beginning of the 20th century. In: Armin Herrmann, Charlotte Schönbeck (Hrsg.): Technology and science. VDI-Verlag, Düsseldorf 1991, p. 401.
- ↑ Christoph Scriba, Bertram Maurer: Technology and Mathematics. In: Armin Herrmann, Charlotte Schönbeck (Hrsg.): Technology and science. VDI-Verlag, Düsseldorf 1991, p. 54f.
- ↑ Marcus Popplow: Insecure Careers: Engineers in the Middle Ages and Early Modern Age 500-1750. In: Walter Kaiser, Wolfgang König (ed.): History of the engineer. Carl Hanser Verlag, Munich / Vienna 2006, p. 116f.
- ↑ Ulrich Troitzsch: Technical change in state and society between 1600 and 1750. In: Akos Paulinyi, Ulrich Troitzsch: Propylaen technology history. Volume 3, pp. 45-47, 249-264.
- ↑ Marcus Popplow: Insecure Careers: Engineers in the Middle Ages and Early Modern Age 500-1750. In: Walter Kaiser, Wolfgang König (ed.): History of the engineer. Carl Hanser Verlag, Munich / Vienna 2006, pp. 108–118.
- ^ Charlotte Schönbeck: Renaissance - natural sciences and technology between tradition and new beginnings. In: Armin Herrmann, Charlotte Schönbeck (Hrsg.): Technology and science. VDI-Verlag, Düsseldorf 1991, pp. 244-251.
- ↑ Alois Huning: The philosophical tradition. In: Friedrich Rapp (Ed.): Technology and Philosophy. VDI-Verlag, Düsseldorf 1990, pp. 31-33.
- ↑ Alois Huning: The philosophical tradition. In: Friedrich Rapp (Ed.): Technology and Philosophy. VDI-Verlag, Düsseldorf 1990, pp. 33-35.
- ↑ Armin Herrmann, Charlotte Schönbeck (ed.): Technology and science. VDI-Verlag, Düsseldorf 1991, pp. 217f.
- ↑ Christoph Scriba, Bertram Maurer: Technology and Mathematics. In: Armin Herrmann, Charlotte Schönbeck (Hrsg.): Technology and science. VDI-Verlag, Düsseldorf 1991, pp. 55-59.
- ↑ Christoph Scriba, Bertram Maurer: Technology and Mathematics. In: Armin Herrmann, Charlotte Schönbeck (Hrsg.): Technology and science. VDI-Verlag, Düsseldorf 1991, p. 56.
- ^ Andreas Kleinert: Technology and natural science in the 17th and 18th centuries. In: Armin Herrmann, Charlotte Schönbeck (Hrsg.): Technology and science. VDI-Verlag, Düsseldorf 1991, pp. 278f.
- ↑ Christoph Scriba, Bertram Maurer: Technology and Mathematics. In: Armin Herrmann, Charlotte Schönbeck (Hrsg.): Technology and science. VDI-Verlag, Düsseldorf 1991, p. 57f.
- ^ Ulrich Troitzsch: Technical change in state and society between 1600 and 1750. In: Wolfgang König (Hrsg.): Propylaen technology history. Volume 3, pp. 264-267, 259-261.
- ^ Andreas Kleinert: Technology and natural science in the 17th and 18th centuries. In: Armin Herrmann, Charlotte Schönbeck (Hrsg.): Technology and science. VDI-Verlag, Düsseldorf 1991, pp. 282f.
- ^ Andreas Kleinert: Technology and natural science in the 17th and 18th centuries. In: Armin Herrmann, Charlotte Schönbeck (Hrsg.): Technology and science. VDI-Verlag, Düsseldorf 1991, pp. 285-288.
- ↑ Christoph Scriba, Bertram Maurer: Technology and Mathematics. Pp. 50, 57f.
- ↑ Gideon Freudenthal, Oliver Schlaudt: The materialist program. Sabine Maasen, Mario Kaiser, Martin Reinhart, Barbara Sutter (eds.): Handbuch Wissenschaftssoziologie. Springer, Wiesbaden 2012, ISBN 978-3-531-17443-3 , pp. 38–40, pp. 35–45.
- ^ Propylaea history of technology. Volume 3, pp. 231-240.
- ^ Wolfgang König: From civil servant to industrial employee: The engineers in France and Germany 1750-1945. In: Walter Kaiser, Wolfgang König (ed.): History of the engineer. Carl Hanser Verlag, Munich / Vienna 2006, pp. 179–184.
- ^ Wolfgang König: From civil servant to industrial employee: The engineers in France and Germany 1750-1945. In: Walter Kaiser, Wolfgang König (ed.): History of the engineer. Carl Hanser Verlag, Munich / Vienna 2006, pp. 190–192.
- ^ PW Musgrave: Technical Change, the Labor Force and Education. Oxford 1967.
- ↑ Lothar Gall (Ed.): Encyclopedia of German History. Volume 70: Christian Kleinschmidt: Technology and Economy in the 19th and 20th Centuries. Oldenbourg Verlag, Munich, 2007, pp. 1–26.
-
^ Contributions in Wolfgang König (Ed.): Propylaea History of Technology. Propylaea, Berlin 1997:
- Volume 3: Ulrich Troitzsch: Technical Change in State and Society between 1600 and 1750. P. 280–337, 383–428,
- Volume 4: Networks, Steel and Electricity - 1840–1914 :
- Weber: Shortening of time and space - techniques without balance between 1840 and 1880. pp. 59–77, 85–108, 126–133.
- König: Mass Production and Consumption of Technology - Lines of Development and Driving Forces between 1880 and 1914. P. 329–387.
- ↑ Günter Spur: On the change in the industrial world through machine tools. Carl Hanser Verlag, Munich / Vienna 1991, pp. 160-193, 233-279, 343-362.
- ↑ König: Mass production and technology consumption - lines of development and driving forces between 1880 and 1914. In: Wolfgang König, Wolfhard Weber: Propylaen technology history. Volume 4: Networks, Steel and Electricity - 1840–1914.
-
↑ Contributions in: Armin Herrmann, Charlotte Schönbeck (Ed.): Technology and Science. VDI-Verlag, Düsseldorf 1991:
- Christoph Scriba, Bertram Maurer: Technology and Mathematics. Pp. 60-63.
- Gerhard Zweckbronner: Technical science in the industrialization process up to the beginning of the 20th century. Pp. 405-407, 418-422.
- ^ Daniel Bieber: Technology development and industrial work. Campus Verlag, Frankfurt / New York 1997, p. 147.
- ↑ Gerhard Bronner purpose: Technical Science in the industrialization process to the beginning of the 20th century. In: Armin Herrmann, Charlotte Schönbeck (Hrsg.): Technology and science. VDI-Verlag, Düsseldorf 1991, pp. 411-413.
- ↑ Gerhard Bronner purpose: Technical Science in the industrialization process to the beginning of the 20th century. In: Armin Herrmann, Charlotte Schönbeck (Hrsg.): Technology and science. VDI-Verlag, Düsseldorf 1991, pp. 414-416.
- ↑ Günter Spur: On the change in the industrial world through machine tools. Carl Hanser Verlag, Munich / Vienna 1991, pp. 313-325.
- ↑ Gerhard Bronner purpose: Technical Science in the industrialization process to the beginning of the 20th century. In: Armin Herrmann, Charlotte Schönbeck (Hrsg.): Technology and science. VDI-Verlag, Düsseldorf 1991, p. 422f.
- ↑ Gerhard Bronner purpose: Technical Science in the industrialization process to the beginning of the 20th century. In: Armin Herrmann, Charlotte Schönbeck (Hrsg.): Technology and science. VDI-Verlag, Düsseldorf 1991, p. 415f.
- ↑ Gerhard Bronner purpose: Technical Science in the industrialization process to the beginning of the 20th century. In: Armin Herrmann, Charlotte Schönbeck (Hrsg.): Technology and science. VDI-Verlag, Düsseldorf 1991, p. 424f.
-
^ Articles in Laetitia Boehm, Charlotte Schönbeck (ed.): Technology and education
- Claus Priesner: Polytechnical associations and technical education. Pp. 235-258.
- Wolfgang König: Technical associations as an educational institution. Pp. 260-276.
- Hans-Martin Kirchner : Magazines and technical education. Pp. 319-338, especially p. 322.
- ↑ Kees, Gispen: The fettered Prometheus - The engineers in Great Britain and in the United States 1750-1945. In: Walter Kaiser, Wolfgang König (ed.): History of the engineer. Carl Hanser Verlag, Munich / Vienna 2006, pp. 127–175.
- ↑ Kees Gispen: Prometheus Bound - The engineers in the UK and in the United States from 1750 to 1945. In: Walter Kaiser, Wolfgang König (ed.): History of the engineer. Carl Hanser Verlag, Munich / Vienna, 2006, pp. 136f., 140, 143.
- ↑ Kees, Gispen: The fettered Prometheus - The engineers in Great Britain and in the United States 1750-1945. In: Walter Kaiser, Wolfgang König (ed.): History of the engineer. Carl Hanser Verlag, Munich / Vienna 2006, p. 157.
- ^ Propylaea history of technology. Volume 4, pp. 398f.
- ↑ Kees, Gispen: The fettered Prometheus - The engineers in Great Britain and in the United States 1750-1945. In: Walter Kaiser, Wolfgang König (ed.): History of the engineer. Carl Hanser Verlag, Munich / Vienna 2006, p. 148f.
- ^ Propylaea history of technology. Volume 4, pp. 115, 400.
- ↑ Günter Spur: On the change in the industrial world through machine tools. Carl Hanser Verlag, Munich / Vienna 1991, p. 329f.
- ↑ Kees Gispen: Prometheus Bound: The engineers in the UK and in the United States from 1750 to 1945. In: Walter Kaiser, Wolfgang König (ed.): History of the engineer. Carl Hanser Verlag, Munich / Vienna 2006, pp. 150–175.
- ↑ Kees, Gispen: The fettered Prometheus - The engineers in Great Britain and in the United States 1750-1945. In: Walter Kaiser, Wolfgang König (ed.): History of the engineer. Carl Hanser Verlag, Munich / Vienna, 2006, p. 164.
- ^ Wolfgang König: From civil servant to industrial employee: The engineers in France and Germany 1750-1945. In: Walter Kaiser, Wolfgang König (ed.): History of the engineer. Carl Hanser Verlag, Munich / Vienna 2006, pp. 179–229.
- ^ Propylaea history of technology. Volume 4, pp. 111f., 393ff.
- ^ Karl-Heinz Manegold: History of the technical universities. In: Laetitio Boehm, Charlotte Schönbeck (ed.): Technology and education.
- ↑ Laetitio Boehm, Charlotte Schönbeck (ed.): Technology and education. P. 241f.
-
↑ Various articles from: Laetitio Boehm, Charlotte Schönbeck (Hrsg.): Technology and education :
- Helmuth Albrecht: The beginnings of a technical education system
- Wolf Ekkehard Traebert : Technology and general education schools
- Gustav Grüner: Development of technical schools
- ↑ Laetitio Boehm, Charlotte Schönbeck (ed.): Technology and education. P. 240f.
- ↑ Wolf Ekkehard Traebert: Technology and general education schools. In: Laetitio Boehm, Charlotte Schönbeck (ed.): Technology and education. P. 158.
- ↑ Lothar Gall (Ed.): Encyclopedia of German History. Volume 82: Hans-Christof Kraus: Culture, Education and Science in the 19th Century. Oldenbourg Verlag, Munich 2008, p. 45.
- ^ Karl-Heinz Manegold: History of the technical universities. In: Laetitio Boehm, Charlotte Schönbeck (ed.): Technology and education. Pp. 209-231.
- ^ Wolfgang König: From civil servant to industrial employee: The engineers in France and Germany 1750-1945. In: Walter Kaiser, Wolfgang König (ed.): History of the engineer. Carl Hanser Verlag, Munich / Vienna 2006, pp. 207f.
-
↑ Laetitio Boehm, Charlotte Schönbeck (ed.): Technology and education. Pp. 235–276:
- Claus Priesner: Polytechnical associations and technical education
- Wolfgang König: Technical associations as an educational institution
- ^ Walter Kaiser: Engineers in the Federal Republic of Germany. In: Walter Kaiser, Wolfgang König (ed.): History of the engineer. Carl Hanser Verlag, Munich / Vienna 2006, pp. 247–266.
- ↑ Heinz Blenke: Technical Sciences in Transition. In: Armin Herrmann, Charlotte Schönbeck (Hrsg.): Technology and science. VDI-Verlag, Düsseldorf 1991.
- ^ Walter Kaiser: Engineers in the Federal Republic of Germany. In: Walter Kaiser, Wolfgang König (ed.): History of the engineer. Carl Hanser Verlag, Munich / Vienna 2006, pp. 217–233, 244.
- ^ Propylaea history of technology. Volume 5, pp. 512-516.
- ^ Walter Kaiser: Engineers in the Federal Republic of Germany. In: Walter Kaiser, Wolfgang König (ed.): History of the engineer. Carl Hanser Verlag, Munich / Vienna 2006, pp. 217–241.
- ↑ Wolfgang König, Helmuth Schneider (Ed.): The technology-historical research in Germany from 1800 to the present. kassel university press, Kassel 2007, ISBN 978-3-89958-318-2 , pp. 248, 293f. ( Full text ; PDF; 5.1 MB)
- ↑ Klaus Kornwachs (Ed.): Technological knowledge - development, methods, structures. Springer, Berlin 2010, p. 11.